ABSTRACT
Periodontitis is one of the most common oral diseases worldwide and is caused by a variety of interactions between oral bacteria and the host. Here, pathogens induce inflammatory host responses that cause the secretion of proinflammatory cytokines such as IL-1β, IL-6, and IL-8 by oral epithelial cells. In various systems, it has been shown that inflammation compromises physical barriers, which enables bacteria to invade the tissue. In this study, we investigated the barrier properties of the oral mucosa under physiological and inflamed conditions. For this purpose, we assessed the influence of IL-1β on the transepithelial electrical resistance and in particular on tight junctions in vitro in human stratified squamous epithelium models. Indirect immunofluorescence and western blot analyses were performed to investigate localization and expression of tight junction proteins in primary gingival cells, immortalized gingival cells and native gingiva. Furthermore, the TEER of gingival keratinocytes was assessed. The results showed that IL-1β led to strengthening of the gingival keratinocyte barrier. This was demonstrated by an increase in TEER, the upregulation of TJ proteins, and an increase in the formation of TJ strands. The IL-1β-mediated upregulation of occludin was prevented by the NF-κB inhibitor BAY 11–7085. These observations provide insights into host responses in the early stages of periodontal disease and offer information about TJ formation in human gingival epithelial cells under physiological and inflammatory conditions. Comprehensive knowledge of the physical barrier during inflammation may help in developing strategies to effectively target the inflammatory barrier to improve the bioavailability of drugs for the treatment of periodontitis.
Introduction
Periodontitis is a bacterially induced inflammatory disease of the oral mucosaCitation1 that extends to the periodontium and gradually destroys the periodontal fibers and alveolar bone.Citation2 Approximately 50% of the world’s population shows clear symptoms of mild or moderate forms of periodontitis and even 10–12% suffer from more severe forms of periodontitis.Citation3,Citation4 If left untreated, periodontitis is associated with increased systemic inflammatory diseases, with potential for an increased risk of stroke, myocardial infarction, atherosclerosis, and diabetes mellitus.Citation5,Citation6
In recent years, there has been a paradigm shift in which the role of bacteria has receded in favor of the inflammatory process and the host response. Periodontitis is, therefore, considered to be an inflammation that is initially induced by subgingival microorganisms that subsequently provoke an inflammatory host response, which is responsible for the tissue loss.Citation7 As a first line of epithelial defense, oral mucosal epithelial cells secrete proinflammatory cytokines such as IL-1β, IL-6, and IL-8 [CXCL8].Citation8-Citation10 Various studies have shown that IL-1β, in particular, is elevated in diseased gingival tissue and gingival crevicular fluid.Citation11,Citation12 After activation and secretion, IL-1β induces a series of inflammatory reactions in cells of the periodontium, which contributes to the course of periodontitis.Citation13,Citation14 Apart from this chemical barrier characterized by a number of soluble mediators, the gingiva also forms a physical barrier to resist mechanical forces and prevent the penetration of pathogens that may damage gingival cells. The physical barrier consists primarily of the stratum corneum, the outermost layer of the stratified squamous epithelium, and the underlying tight junctions (TJs).Citation15 TJs are intercellular junctions that seal adjacent keratinocytes.Citation15 Under physiological conditions, TJs act as a semipermeable barrier for the paracellular transport of ions, solutes, and waterCitation16-Citation19 and maintain tissue homeostasis.Citation20,Citation21
In contrast to simple epithelia, the skin and gingiva consist of several layers whose cells undergo different stages of differentiation. Some TJ proteins appear to be expressed primarily in the stratum granulosum (SG), whereas individual TJ proteins are expressed beyond the SG).Citation22,Citation23,Citation24,Citation25
Although TJs vary in composition, the tetraspan transmembrane proteins occludin (OCLN) and members of the claudin (CLDN) family have been identified in all stratified mammalian epithelia.Citation26 The cytoplasmic parts of these transmembrane proteins are associated with a dense plaque structure containing proteins such as ZO-1.Citation26,Citation27 Knock-out experiments revealed that CLDN-1 is essential for sealing the epidermis.Citation17 Since healthy gingival tissue can only be examined in a limited way, there are little data available to describe the healthy human situation in the gingiva. The expression of claudin-1, −2, and occludin has been shown in primary and immortalized gingival keratinocytes.Citation28,Citation29,Citation30,Citation31 Gene expression analyses of multilayer gingival epithelial cell cultures showed a strong expression of claudin-1, −4, −12, −17, −25, JAM-A, and occludin.Citation32 At the protein level, claudin-1, −4, −5, and occludin were found to be expressed in primary and immortalized human gingival keratinocytes.Citation29,Citation33
It has been shown that TJs are influenced by cytokines in various tissues.Citation34 In the present study, we addressed the question of whether pro-inflammatory mediators affect the physical barrier of the gingival epithelium. We therefore investigated the influence of the periodontitis-relevant cytokine IL-1β on TJs in in vitro models derived from primary and immortalized gingival keratinocytes.
Materials and methods
Cells and cell culture
For experiments, hTERT-immortalized human gingival keratinocytes (OKG4; kindly provided by Susan Gibbs, Amsterdam) were used.Citation35,Citation36 Primary gingival epithelial cells (GECs) were dissected from gingival tissue samples obtained from tooth extractions and kindly provided by the Department of Oral and Maxillofacial Surgery, Charité. The study was approved by the ethical committee of the Charité – Universitätsmedizin Berlin (Number of the request: EA2/185/16). All volunteers were informed before sampling of the tissues and gave written informed consent. All experiments followed the guidelines of good clinical/laboratory practice (GCP/GLP) and the WHO Declaration of Helsinki 1964, latest update Fortaleza 2013 (64th WMA General Assembly, Brazil, October 2013).
Epithelial cells were seeded on collagen A- (Biochrom, Berlin, Germany; for GEC) or collagen IV-coated (Sigma-Aldrich, St. Louis, USA; for OKG4) cell culture dishes (Corning, Corning, USA) and grown at 37°C and 5% CO2 in DermaLife K Serum-Free Keratinocyte Growth Medium that contained 60 µM Ca2+ (Lifeline® Cell Technology, Frederick, USA), supplemented with 1% Penicillin-Streptomycin (PAN Biotech, Aidenbach, Germany). GECs additionally received 1% Amphotericin B (Biochrom, Berlin, Germany).
Cell differentiation was induced by increasing the Ca2+ level in the medium from 60 µM Ca2+ (low Ca2+) to 1.4 mM (high Ca2+). To achieve this, the medium was exchanged for DermaLife K Serum-Free Keratinocyte Culture Medium that contained 1.4 mM Ca2+. Inflammation of OKG4 and primary gingival cells was induced by administration of 10 ng/ml IL-1β (Immunotools, Friesoythe, Germany) to the cell culture medium. The medium containing IL-1β and the control medium were changed every day. Unstimulated cells served as controls.
To investigate the influence of the NF-κB pathway on occludin expression, cells were pretreated with 10 µM BAY 11–7085 (Sigma-Aldrich, St. Louis, USA) for 1 h.
Human cytokine antibody array
To perform the human cytokine antibody array, which allows the simultaneous detection of 42 different cytokines (Abcam, Cambridge, UK), 2.5 x 105 OKG4 cells per well were seeded into 6-well dishes (Corning, Corning, USA). After the Ca2+ increase, the cells were cultured for 24 h and then stimulated using IL-1β. After 72 h, the medium was collected and stored at −20°C until use. The array was carried out according to the manufacturer’s instructions. A 1 ml volume of culture medium was added to the membrane. Signals were visualized using a VersaDocTM 4000 MP and QuantityOne® 4.6.5 software (BioRad Laboratories, Hercules, USA). For signal quantification, ImageJ’s Protein Array Analyzer plug-in was used (Wayne Rasband, National Institutes of Health, USA, version 1.52a). Signal intensities below 10% of the positive control were excluded from evaluation to avoid false-positive results.
ELISA detecting IL-6 and IL-8
Sandwich ELISAs (Immunotools, Friesoythe, Germany) were performed to quantify cytokine concentrations. The cell culture supernatants were collected as previously described. In brief, medium from 6-well plates (Corning, Corning, USA) was removed from the culture after 6, 24, or 72 h of stimulation time and stored at −20°C until use. Samples were subjected to ELISA. The assay was performed in accordance with the manufacturer’s instructions using 100 µl of culture medium per well.
MTT assay
Cells (1x10⁴) were seeded in 100 µl growth medium per well in 96-well plates. Wells without cells (blank), unstimulated cells and cells lysed with 4% Triton X-100 before performing the MTT assay served as controls. The stimulation was performed by changing the medium the following day. The MTT assay was performed 24 h, 48 h, and 72 h after stimulation. After 4 h of incubation with 10 µl MTT solution (Thiazolyl Blue Tetrazolium Bromide, Sigma-Aldrich, St. Louis, USA) per well, the supernatant was aspirated. By applying 100 µl 5% 1 N HCl (v/v) in isopropanol (Thermo Fisher, Waltham, USA) per well, the reduction of the dye to violet-colored formazan was achieved. The colorimetric reaction was measured photometrically at 570 nm and subsequently quantified.
SRB assay
The cells were seeded, cultured, and stimulated analogously to the MTT assay. After stimulation, 100 µl of 10% TCA (Carl Roth, Karlsruhe, Germany) per well was added and incubated at 4°C for 1 h. The cells were incubated with 100 µl SRB solution (0.057% sulforhodamine B) (m/v; Sigma-Aldrich, St. Louis, USA) in 1% acetic acid per well at room temperature. The cells were repeatedly washed using 200 µl 1% acetic acid and were dried at room temperature. Tris solution (10 mM; pH 10.5; Carl Roth, Karlsruhe, Germany) was added (200 µl per well) and incubated for 15 minutes at room temperature. The cell density was monitored using a microplate reader at 492 nm. Three independent experiments were performed in triplicate.
Measurement of the transepithelial electrical resistance (TEER)
Gingival keratinocytes (2.7 x 104) were cultivated on collagen-coated polycarbonate filters (12 mm, 0.4 µm; Corning, Corning, USA) in DermaLife cell culture medium that contained 60 µM Ca2+. When cell confluency was achieved, the cells were transferred to high Ca2+ medium containing 1.4 mM Ca2+. TEER values were measured using an EndOhm-12 chamber (World Precision Instruments, Sarasota, USA) in combination with a Millicell ERS-2 volt-ohm meter (Merck Millipore, Burlington, USA). The measurement was performed after equilibration in the respective medium. To determine the influence of IL-1β on the course of the resistance, the TEER values were monitored after 24, 48, and 72 h in IL-1β-treated and untreated cells. The cells cultivated on filters in the presence of 60 µM Ca2+ were kept as negative controls. TEER values were calculated by background (blank filters) subtraction and multiplication of the surface area.
Western blot analysis
OKG4 cells (2.5 x 105) were placed in 60 mm-culture dishes (Corning, Corning, USA) in DermaLife, differentiated as described above and stimulated with IL-1β for 24 and 72 h. For Western blotting, cells were washed with PBS and then lysed in lysis buffer (50 mM β-glycerophosphate pH 7.6, 1.5 mM EGTA, 1.0 mM EDTA, 1% (v/v) Triton X-100, 0.2% (v/v) protease inhibitor cocktail (PIC; Serva, Heidelberg, Germany), 0.4% (v/v) PMSF, 100 mM natrium vanadate, 500 mM NaF). Cell supernatants were denatured by boiling in Laemmli’s buffer for 5 min at 95°C. Then, 60 μg of protein was separated under reducing conditions by 10% SDS-PAGE and blotted onto nitrocellulose membranes (Thermo Fisher, Waltham, USA) for 1 h at 0.25 A. The membranes were subsequently blocked in 5% BSA/TBST and then incubated with the respective primary antibodies in blocking buffer overnight at 4°C. Primary antibodies and respective working concentrations are listed in . After incubation with appropriate horseradish peroxidase (HRP)-conjugated secondary antibodies, signals were detected using Western Lightning® Plus ECL (PerkinElmer, Waltham, USA) chemiluminescence reagents and a VersaDocTM 4000 MP system with QuantityOne® software. Signals were quantified using ImageJ and subsequently normalized to the respective loading control.
Histology and immunofluorescence analysis
For immunostaining, immortalized and primary gingival epithelial cells were seeded at 1.2 x 104 cells per well on collagen-coated 8-well NuncTM Lab-Tek II Chamber SlidesTM (Thermo Fisher, Waltham, USA) and treated as described above. Furthermore, cryo-sections of human gingiva with a thickness of 7 μm were subjected to immunofluorescence analysis.
Cells and sections were fixed for 15 min at RT with 4% paraformaldehyde (m/v) in PBS+/+ (1 mM Ca2+, 0.5 mM Mg2+), pH 7.4. After blocking, the cells were incubated with primary antibodies overnight at 4°C (Appendix Table 1). After extensive washing with PBS+/+, an appropriate secondary antibody (Thermo Fisher, Waltham, USA) was added for 1 h at RT. Cell nuclei were stained with Hoechst 33342 dye (Sigma-Aldrich, St. Louis, USA). Cover slips were mounted using FluoromountTM Mounting Medium (Sigma-Aldrich, St. Louis, USA). For histological evaluation, sections were stained with Mayer’s hematoxylin and eosin (HE; Carl Roth, Karlsruhe, Germany).
Images were captured with an LSM700MAT confocal laser scanning microscope (Zeiss, Oberkochen, Germany) or an Axiovert 200 fluorescence microscope (Zeiss, Oberkochen, Germany) and processed with ZEN software (Zeiss, Oberkochen, Germany) or AxioVision Release 4.8.2 SP3 (Zeiss, Oberkochen, Germany). For comparison, images were acquired using identical settings for exposure and processing.
Statistical analysis
All experiments were performed at least three times. Data were displayed as the mean value (bars) and standard deviation (error bars) unless stated otherwise. Pairwise datasets were tested for significant differences using Student’s t-test. Significance was indicated by P-values and P < .05 was considered significant.
To compare IL-1β-treated and untreated cells, the starting points of stimulation were set to 100% due to different TEER starting values. Subsequently, the test groups were compared to the controls at the respective time point.
For Western blot analyses, the controls were set to 1, and the test groups were presented as relative values.
Results
IL-1β induces an inflammatory response in gingival epithelial cells
Since IL-1β has been identified as an essential component in the pathogenesis of periodontitis,Citation12,Citation37,Citation38 we investigated whether this proinflammatory cytokine is able to induce an inflammatory response in the immortalized gingival keratinocyte cell line OKG4 using a human cytokine antibody array. After 72 h of IL-1β stimulation, not only the amount of IL-1β but also the amount of the inflammation marker IL-8 was increased (); ). The concentration determined for IL-6, a classical mediator of the early inflammatory response, was very low in both experiments.
Figure 1. IL-1β induces the secretion of the proinflammatory cytokines IL-6 and IL-8 in gingival keratinocytes.
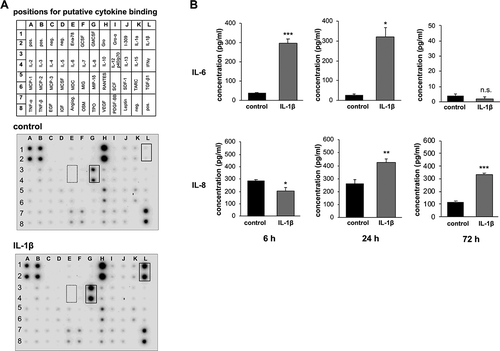
We verified the results of the cytokine array for IL-8 and investigated the influence of IL-1β on the secretion of IL-6 at earlier time points using the ELISA technique ()). After 72 h of stimulation, the ELISA results confirmed the data obtained with the cytokine array for both cytokines. While IL-6 could hardly be detected in the supernatants of IL-1β-treated and untreated cells (IL-1β: 1.77 ± 1.37 pg/ml, control: 3.66 ± 1.24 pg/ml), IL-8 was significantly upregulated by IL-1β after 72 h (IL-1β: 330.99 ± 14.39 pg/ml, control: 118.12 ± 6.02 pg/ml).
We found IL-6 to be expressed earlier. After 6 h of stimulation, the secretion of IL-6 was highly significantly increased in IL-1β-treated cells (296.85 ± 21.84 pg/ml) compared to control cells (37.62 ± 4.32 pg/ml). We found this cytokine to be still expressed at significantly higher levels in IL-1β-treated cells (319.47 ± 48.87 pg/ml) compared to the control cells (26.86 ± 6.37 pg/ml) after 24 h.
Unexpectedly, the IL-8 concentration of untreated cells after 6 h was higher compared to untreated cells after 72 h. IL-1β slightly reduced the concentration of IL-8 (202.36 ± 27.91 pg/ml) compared to control cells (285.29 ± 11.47 pg/ml) after 6 h. After 24 h, IL-8 was significantly elevated in cells treated with IL-1β (423.73 ± 26.72 pg/ml) compared to control cells (259.55 ± 30.32 pg/ml).
Overall, IL-6 and IL-8 showed different secretion patterns in unstimulated cells. IL-1β caused a rapid upregulation of IL-6, which decreased over time, whereas the IL-1β-induced secretion of IL-8 was delayed but more stable over time. These data showed that IL-1β induced an inflammatory response in gingival epithelial cells.
Even though the number of proinflammatory mediators secreted upon IL-1β stimulation was limited, we were able to show that this cytokine leads to a persistent inflammatory response over a period of 72 h in gingival epithelial cells.
IL-1β does not affect proliferation or viability but enhances the physical barrier of gingival epithelial cells
We further investigated whether IL-1β causes cytotoxic effects or affects cell proliferation. We found that IL-1β did not have an impact on metabolic activity/cell viability nor on cell proliferation using the MTT and SRB assays, respectively (). Furthermore, nuclei of IL-1β-treated and untreated cells were stained with Hoechst dye. Fluorescence analysis and the counting of cell nuclei from randomly chosen visual fields of each experimental condition confirmed that IL-1β had no influence on cell proliferation ()).
Figure 2. IL-1β does not influence the metabolic activity or proliferation of OKG4 cells.
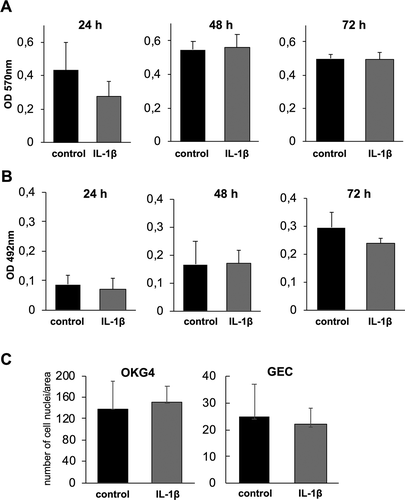
Moreover, the visualization of cell membranes of OKG4 cells by fluorescence-labeled lectin wheat germ agglutinin (WGA) revealed that IL-1β had no effect on cell morphology. This was also confirmed for primary oral keratinocytes (GECs) isolated from biopsies of human gingiva ().
In the gingiva, epithelial cells form a physical barrier consisting of the stratum corneum and the TJs,Citation15 which among other functions, hinder the penetration of pathogens. To assess whether the strength of the physical barrier is affected by IL-1β, we measured the transepithelial electrical resistance (TEER). The switch to a medium containing a high Ca2+ concentration of 1.4 mM led to a biphasic resistance curve over time in unstimulated GECs. Here, the cells exhibited a maximum of resistance of 706.50 ± 470.29 Ω x cm2 at d 9 ()). Subsequently, the TEER decreased but remained stable until d 14, with a mean value of 264.11 ± 39.85 Ω x cm2. GECs cultured at a low Ca2+ concentration of 60 µM showed a consistent average TEER of 8.73 ± 2.54 Ω x cm2 over 14 d.
Figure 3. IL-1β increases transepithelial resistance in a human 3D epithelial model.
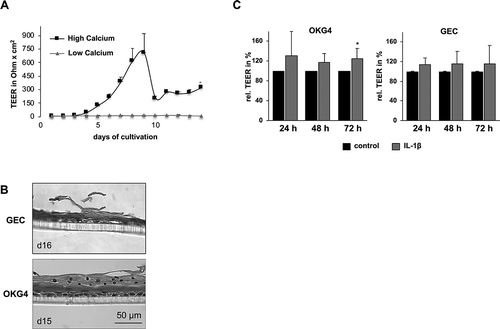
The calcium switch induced differentiation in GECs and OKG4 cells, where the cells formed a multilayered tissue with signs of desquamation, as shown by HE-stained microsections ()). Therefore, these constructs could be regarded as simplified 3D in vitro models of stratified squamous gingival epithelium.
Additional treatment of OKG4 cells with IL-1β for 24, 48 and 72 h led to an increase in resistance of between 20% and 30% compared to untreated cells. After 72 h, this change was found to be significant. A similar effect was observed in GECs, although the clear trend did not reach statistical significance ()).
IL-1β increases TJ formation and the expression of TJ proteins in gingival epithelial cells
TJs are part of the physical barrier, and the existence of TJs and the expression of tight junction proteins in gingival epithelial cells have been described.Citation33,Citation39,Citation40 Nevertheless, the exact distribution of the numerous different tight junction proteins in the healthy human gingiva is unknown. Thus, we initially studied the localization of claudin-1 (CLDN1), zonula occludens-1 (ZO-1), and occludin (OCLN) in healthy gingiva using indirect immunofluorescence analysis (). This study revealed that all three proteins were expressed in mature human gingiva. For CLDN1 and ZO-1, distinct staining of intercellular contacts in the stratum granulosum was observed. Additional signals in lower layers appeared more diffuse. Both proteins were excluded from the stratum corneum and the stratum basale. OCLN was mainly expressed in the stratum granulosum (). Costaining with antibodies directed against OCLN and ZO-1 revealed signs of colocalization only in superficial parts of the stratum granulosum ().
Figure 4. Expression and localization of CLDN1, ZO-1 and OCLN in healthy human gingiva.
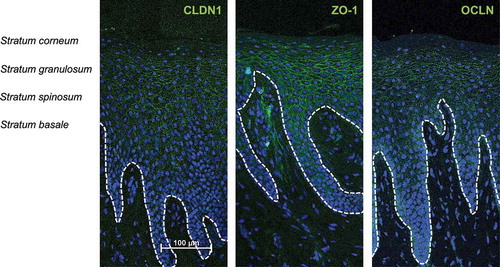
Since IL-1β increased the TEER in gingival epithelial cells, we investigated whether this increase is associated with altered expression or localization of the tight junction components CLDN-1, ZO-1, and OCLN in human gingival epithelial cells. In OKG4 cells and GECs cultured in the presence of 1.4 mM Ca2+, CLDN-1 was observed in intercellular contacts. Since Ca2+ is an important inducer of TJ formation,Citation41 tight junctions were absent in cells that had been cultured in the presence of a low Ca2+ concentration of 60 µM ().
A detailed fluorescence analysis revealed that Ca2+ induced the organization of defined strands containing ZO-1 and OCLN in GECs ()) and in OKG4 cells () under the control conditions. Quantification of the length of ZO-1/OCLN-positive TJ strands revealed that the cytokine treatment significantly increased the quantity of TJ strands in both cell types compared to that in untreated cells, respectively (–c)).
Figure 5. IL-1β increases the expression of OCLN and enhances the formation of TJ stands in gingival keratinocytes.
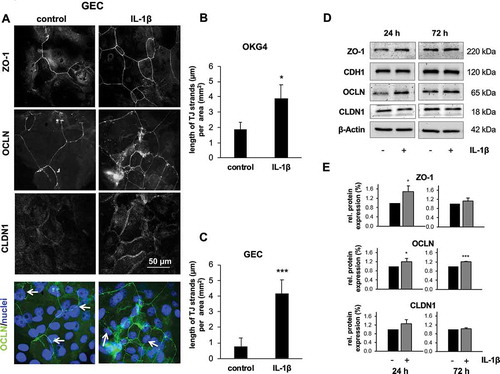
This IL-1β-dependent enhanced formation of TJ strands was accompanied by increased expression of ZO-1 and OCLN, as shown by Western blot analysis using OKG4 cells ()). The upregulation of ZO-1 and OCLN after 24 h was found to be significant ()), while expression of CLDN1 and E-cadherin (CDH1), a cell adhesion molecule localized to adherens junctions, remained unaffected by IL-1β. Quantification of Western blot signals revealed that the amount of OCLN in IL-1β-treated cells was still significantly increased after 72 h compared to that in control cells ()).
The counterstaining of cell nuclei with Hoechst 33342 showed that the OCLN-positive TJ strands partially crossed the cell nuclei (), lower panel, marked with arrows). Therefore, we determined the distribution of OCLN and ZO-1 in IL-1β-treated and untreated cells more precisely and acquired z-stacks of TJ strands of OCLN/ZO-1 overlays (). The reconstructed orthogonal projections revealed that untreated OKG4 cells were primarily organized in flat monolayers, with most cells being positive for ZO-1 (xz1). In areas of visible TJ strands, some cells and cell nuclei were located above the basal layer. In these areas, we observed OCLN in colocalization with ZO-1 but its distribution also extended above the colocalization area without ZO-1. Additional treatment with IL-1β increased the signals of both colocalized OCLN and ZO-1 and subapical localized OCLN ()).
Figure 6. IL-1β enhances the subapical localization of OCLN in TJs.
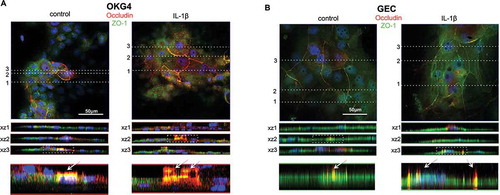
These data were confirmed for primary GECs ()), with distinct staining positive for OCLN and ZO-1, with spots positive for only OCLN in the subapical region of the cells. In GECs, these effects were also enhanced by IL-1β.
Inhibition of NF κB activation prevents the IL-1β-induced upregulation of OCLN
Since NF-κB is a key transcription factor that regulates proinflammatory responses in various cells, including epithelial cells,Citation42 we investigated whether NF-κB activation contributes to the IL-1β-induced upregulation of OCLN. For this purpose, OKG4 cells were stimulated with BAY 11–7085, an inhibitor of IκB α phosphorylation that prevents NF-κB activation, in the presence and absence of IL-1β and analyzed occludin expression using Western blotting. As shown in , the IL-1β-induced increase in occludin was prevented by the inhibitor. A slightly decrease in occludin expression caused by BAY 11–7085 was also observed under control conditions.
Figure 7. Inhibition of NF-κB activation prevents IL-1β-induced upregulation of OCLN.
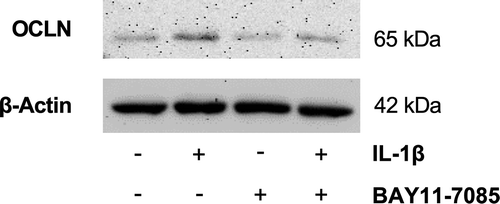
Discussion
The oral mucosa presents a stratified squamous epithelium that forms important physical and immunological barriers that prevent pathogens from invading the tissue. Impairment of the barrier facilitates penetration of plaque-associated bacteria, which may cause periodontal inflammation.Citation43
During inflammatory processes in the oral cavity, the concentration of IL-1 increases in the gingival crevicular fluidCitation44 and in the inflamed epithelium.Citation12 Periodontitis-relevant pathogens such as P. gingivalis and F. nucleatum also provoke a high IL-1 release,Citation45 and the biological effects of IL-1 are directly associated with periodontal inflammatory processes, including collagenase activation, bone resorption, and the induction of other inflammatory mediators such as IL-6.Citation8,Citation46 Inhibition of IL-1 activity in a nonhuman primate model led to reduction of the inflammation, attachment loss, and bone resorption induced by periodontal pathogens.Citation47 Thus, IL-1 is regarded as a key cytokine of periodontitis and was therefore selected in this study to generate an inflammatory oral mucosa model derived from gingival epithelial cells.
Characterization of the influence of IL-1β on OKG4 cells revealed that this cytokine induced the secretion of the inflammatory markers IL-6 and IL-8. This is consistent with findings of other groups, which showed that gingival epithelial cells produce IL-6 and IL-8 in response to IL-1;Citation38,Citation48 these factors are also involved in the course of periodontitis.Citation49 Therefore, our established epithelial system, in which cells undergo a transition from cubic cells on the basal side to squamous cells on the surface, can be regarded as an adequate inflammation model. Here, IL-1β neither impacts proliferation nor influences metabolic activity compared to untreated control cells.
To investigate whether the physical barrier, which is the first line of defense and must resist pathogens, is affected by this inflammatory process, we determined the TEER, which serves as an indicator of epithelial integrity.Citation50 As shown here, GECs formed a tight barrier during differentiation. The TEER values of keratinocytes cultivated in the presence of 1.4 mM Ca2+ were significantly higher than the TEER values of undifferentiated cells, cultivated at 60 µM Ca2+, but were lower than the TEER values measured at commercially available full-thickness equivalents, which also contain connective tissue beneath the epithelium.Citation51
Meyle et al.Citation40 first described the course of resistance of cultured gingival keratinocytes. In their study, the TEER of primary epithelial cells reached its maximum 2 d after the Ca2+ switch to 1.4 mM Ca2+. In our system, the TEER reached its maximum a few days later. It then decreased but remained stable at approximately 250 Ω x cm2. The TEER values obtained with other immortalized cell lines derived from the oral epithelium under similar conditions were all lower than those obtained in our system.Citation52
However, the stability of these systems in culture was limited. Frequently, cell detachment and loss of viability were observed after 7 d.Citation40 In our system, the cell viability and barrier function of gingival epithelial cells was sustained for several weeks.
Treatment of cells with IL-1β strengthened the physical barrier, as TEER values increased over time after cytokine treatment. This was a surprising result since it is known from other tissues, such as the blood-brain barrier,Citation53-Citation55 the intestine,Citation56 and stratified epithelia, including the skin and gingiva,Citation29,Citation43 that inflammatory processes lead to the impairment of barrier function. Thus, a long-term treatment of skin keratinocytes with IL-1β reduced the TEER and the expression of occludin and ZO-1.Citation22 Consistently, a longer infection of gingival keratinocytes with P. gingivalis decreased the TEER and changed the expression pattern of the tight junction components claudin-1, −2, and occludin.Citation29
However, there are also reports that describe a strengthening of the barrier at the early stages of inflammation. Treatment of cultured skin keratinocytes with psoriasis-relevant IL-1β resulted in an immediate increase in barrier resistance.Citation22 It has been further reported that at early stages of P. gingivalis-induced inflammation, the barrier function was increased in oral keratinocytes.Citation29,Citation39
In our models, a significant IL-1β-induced increase in TEER could only be determined for OKG4 cells. In GECs, the resistance was also increased by this cytokine, but this change was not found to be significant. This discrepancy may be explained by the variability among the GEC donors. Cells from donors with a very high TEER per se acted differently in the presence of IL-1β than cells from donors that developed a lower TEER under the control conditions. This differential influence has been already observed by others. Brandner and SchulzkeCitation57 described a donor-dependent decrease or increase in TEER after IL-4 addition to primary skin keratinocytes. They hypothesized that genetic differences as well as a local and unnoticed inflammation before the preparation of the biopsies may be responsible for the discrepancies.
The IL-1β-dependent increase in resistance, observed in OKG4 cells and primary cells from donors with a lower resistance, was accompanied by an increase in the expression of the TJ proteins ZO-1 and OCLN after 24 h in OKG4 cells. An increase in OCLN was still detected after 72 h of IL-1β treatment in these cells. ZO-1, OCLN, and CLDN1 were shown to be expressed in healthy human gingiva. Here, CLDN1 and ZO-1 were observed in suprabasal layers (except the stratum corneum), whereas OCLN was found predominantly in the uppermost layers of the stratum granulosum.
Double-immunolabeling of the gingiva for ZO-1 and OCLN revealed that both proteins were colocalized in few areas of the upper granular cell layer. This specific localization of TJ components has already been described for the mature and developing human skinCitation58 as well as for bovine gingiva.Citation23
In skin, the upregulation of ZO-1 and OCLN in response to IL-1β has also been described.Citation22 The injection of IL-1β in ex vivo porcine skin and human skin models led to a broadened localization of OCLN and ZO-1 within 24 h. Furthermore, Western blot analysis showed an upregulation of ZO-1, whereas the amount of CLDN1 remained unchanged after 24 h. At later stages of psoriasis, downregulation of TJ proteins was observed.Citation22
For gingiva, it has been shown that P. gingivalis is able to elicit a strong IL-1 response.Citation45 It is accepted that P. gingivalis compromises the physical barrier of the oral mucosa by infecting the oral mucosal tissue.Citation29,Citation43 However, it has been found that at very early stages of P. gingivalis-induced inflammation, the expression of TJ proteins is enhanced, leading to an increase in barrier function.Citation29,Citation39 In the skin, pathogenic and nonpathogenic bacteria also cause an upregulation of OCLN and ZO-1 at the beginning of colonization or infection. It was therefore suggested that upregulation of TJ proteins and the associated strengthening of the barrier could be a rescue mechanism after contact between keratinocytes and inflammation-inducing stimuli,Citation59 which is also supported by our data. In particular, OCLN may have an important role in strengthening the barrier, as this protein is stably upregulated after IL-1β treatment. The increase in OCLN expression seems to be controlled by the NF-κB pathway since inhibition of NF-κB activation prevents the upregulation of OCLN. A role for NF-κB for both maintaining normal barrier function and for controlling the inflammatory responses has recently been suggested for the alveolar epithelial barrier.Citation60
For the first time, this study revealed a new perspective on the physical barrier of the gingival epithelium. Even the healthy gingival epithelium is constantly exposed to a variety of commensal and pathogenic bacteria. Thus, a permanent responsiveness is required in order to maintain a healthy homeostasis. Here, the early expression of antimicrobial peptides and pro-inflammatory mediators appear to play a special role.Citation61 This study highlighted a new aspect of how pro-inflammatory mediators may alter resistance to the microbial environment. It was a surprising finding that IL-1β indeed strengthened the physical barrier by increased expression of tight junction proteins, and therewith, increased resistance. Although a significant increase in resistance was only observed in OKG4 cells and not in primary GEC, a significant increase of tight junction strands and a similar change in the distributions of ZO-1 and OCLN after IL-1β treatment were recorded in both cell types. Therefore, we suggest that the strengthening of the physical barrier is an important process in the initial defense mechanisms of gingival epithelial cells.
These data provide insights into host responses at the early stages of inflammation as well as TJ formation in human gingival epithelial cells and their regulation under inflammatory conditions. In particular, ZO-1 and OCLN could be identified as important components of gingival epithelial tight junctions. As mentioned previously, gingival keratinocytes express additional tight junction proteins, which may contribute to barrier function and therefore need to be characterized to achieve comprehensive knowledge of the physical barrier in health and during inflammation. This will help, inter alia, in the design of drug/carrier systems that effectively target the inflamed barrier to improve the bioavailability of drugs for the treatment of periodontitis.
Disclosure of potential conflicts of interest
The authors report no conflicts of interest.
Supplemental Material
Download MS Word (1.8 MB)Acknowledgments
The authors thank Prof. Dr. Jörg-Dieter Schulzke and PD Dr. Susanne Krug (Department of Gastroenterology, Infectiology and Rheumatology, Charité) for providing valuable help for the visualization of tight junctions. We thank Gudrun Mrawietz for excellent technical assistance and Jasmin Berger for quantification of the TJ strands.
Supplementary material
Supplemental data for this article can be accessed on the publisher’s website.
Additional information
Funding
References
- Hajishengallis G, Lamont RJ. Breaking bad: manipulation of the host response by Porphyromonas gingivalis. Eur J Immunol. 2014;44(2):1–17. doi:10.1002/eji.201344202.
- Meyle J, Chapple I. Molecular aspects of the pathogenesis of periodontitis. Periodontol 2000. 2015;69(1):7–17. doi:10.1111/prd.12104.
- Demmer RT, Papapanou PN. Epidemiologic patterns of chronic and aggressive periodontitis. Periodontol 2000. 2010;53:28–44. doi:10.1111/j.1600-0757.2009.00326.x.
- Kassebaum NJ, Bernabé E, Dahiya M, Bhandari B, Murray CJ, Marcenes W. Global burden of severe periodontitis in 1990-2010: a systematic review and meta-regression. J Dent Res. 2014;93(11):1045–1053. doi:10.1177/0022034514552491.
- Preshaw PM, Alba AL, Herrera D, Jepsen S, Konstantinidis A, Makrilakis K, Taylor R. Periodontitis and diabetes: a two-way relationship. Diabetologia. 2012;55(1):21–31. doi:10.1007/s00125-011-2342-y.
- Tonetti MS, Van Dyke TE. Working group 1 of the joint EFP/AAP workshop. Periodontitis and atherosclerotic cardiovascular disease: consensus report of the Joint EFP/AAP Workshop on Periodontitis and Systemic Diseases. J Periodontol. 2013;84(4):S24–29. doi:10.1902/jop.2013.1340019.
- Preshaw PM. Host modulation therapy with anti-inflammatory agents. Periodontol 2000. 2018;76(1):131–149. doi:10.1111/prd.12148.
- Okada H, Murakami S. Cytokine expression in periodontal health and disease. Crit Rev Oral Biol Med. 1998;9(3):248–266. doi:10.1177/10454411980090030101.
- Sfakianakis A, Barr CE, Kreutzer DL. Actinobacillus actinomycetemcomitans-induced expression of IL-1alpha and IL1beta in human gingival epithelial cells: role in IL-8 expression. Eur J Oral Sci. 2001;109:393–401. doi:10.1034/j.1600-0722.2001.00162.x.
- Uchida Y, Shiba H, Komatsuzawa H, Takemoto T, Sakata M, Fujita T, Kawaguchi H, Sugai M, Kurihara H. Expression of IL-1 beta and IL-8 by human gingival epithelial cells in response to Actinobacillus actinomycetemcomitans. Cytokine. 2001;14:152–161. doi:10.1006/cyto.2001.0863.
- Ertugrul AS, Sahin H, Dikilitas A, Alpaslan N, Bozoglan A. Comparison of CCL28, interleukin-8, interleukin-1β and tumor necrosis factor-alpha in subjects with gingivitis, chronic periodontitis and generalized aggressive periodontitis. J Periodontal Res. 2013;48(1):44–51. doi:10.1111/j.1600-0765.2012.01500.x.
- Stashenko P, Fujiyoshi P, Obernesser MS, Prostak L, Haffajee AD, Socransky SS. Levels of interleukin 1 beta in tissue from sites of active periodontal disease. J Clin Periodontol. 1991;18(7):548–554. doi:10.1111/j.1600-051X.1991.tb00088.x.
- Faizuddin M, Bharathi SH, Rohini NV. Estimation of interleukin-1beta levels in the gingival crevicular fluid in health and in inflammatory periodontal disease. J Periodontal Res. 2003;38(2):111–114. doi:10.1034/j.1600-0765.2003.01649.x.
- Groeger SE, Meyle J. Epithelial barrier and oral bacterial infection. Periodontol 2000. 2015;69(1):46–67. doi:10.1111/prd.12094.
- Brandner JM, Zorn-Kruppa M, Yoshida T, Moll I, Beck LA, De Benedetto A. Epidermal tight junctions in health and disease. Tissue Barriers. 2015;3(1–2):e974451. doi:10.4161/21688370.2014.974451.
- Furuse M, Sasaki H, Tsukita S. Manner of interaction of heterogeneous claudin species within and between tight junction strands. J Cell Biol. 1999;147(4):891–903. doi:10.1083/jcb.147.4.891.
- Furuse M, Hata M, Furuse K, Yoshida Y, Haratake A, Sugitani Y, Noda T, Kubo A, Tsukita S. Claudin-based tight junctions are crucial for the mammalian epidermal barrier: a lesson from claudin-1-deficient mice. J Cell Biol. 2002;156(6):1099–1111. doi:10.1083/jcb.200110122.
- Krug SM, Schulzke JD, Fromm M. Tight junction, Selective Permeability, and Related Diseases. Sem Cell Dev Biol. 2014;36:166–176. doi:10.1016/j.semcdb.2014.09.002.
- Tsukita S, Furuse M, Itoh M. Multifunctional strands in tight junctions. Nat Rev Mol Cell Biol. 2001;2:285–293. doi:10.1038/35067088.
- Gonzalez-Mariscal L, Betanzos A, Nava P, Jaramillo BE. Tight junction proteins. Prog Biophys Mol Biol. 2003;81:1–44. doi:10.1016/S0079-6107(02)00037-8.
- Schneeberger EE, Lynch RD. The tight junction: a multifunctional complex. Am J Physiol Cell Physiol. 2004;286:C1213–C1228. doi:10.1152/ajpcell.00558.2003.
- Kirschner N, Poetzl C, von den Driesch P, Wladykowski E, Moll I, Behne MJ, Brandner JM. Alteration of tight junction proteins is an early event in psoriasis: putative involvement of proinflammatory cytokines. Am J Pathol. 2009;175(3):1095–1106. doi:10.2353/ajpath.2009.080973.
- Langbein L. Tight junctions and compositionally related junctional structures in mammalian stratified epithelia and cell cultures derived therefrom. Eur J Cell Biol. 2002;81:419–435. doi:10.1078/0171-9335-00270.
- Brandner JM, Kief S, Grund C, Rendl M, Houdek P, Kuhn C, Tschachler E, Franke WW, Moll I. Organization and formation of the tight junction system in human epidermis and cultured keratinocytes. Eur J Cell Biol. 2002;81:253-263. doi:10.1078/0171-9335-00244.
- Schlüter H, Moll I, Wolburg H, Franke WW. The different structures containing tight junction proteins in epidermal and other stratified epithelial cells, including squamous cell metaplasia. Eur J Cell Biol. 2007;86(11–12).
- Franke WW, Pape FU. Diverse types of junctions containing tight junction proteins in stratified mammalian epithelia. Ann NY Acad Sci. 2012;1257:152–157. doi:10.1111/j.1749-6632.2012.06504.x.
- Stevenson BR, Siliciano JD, Mooseker MS, Goodenough DA. Identification of ZO-1: a high molecular weight polypeptide associated with the tight junction (zonula occludens) in a variety of epithelia. J Cell Biol. 1986;103(3):755–766. doi:10.1083/jcb.103.3.755.
- Fujita T, Firth JD, Kittaka M, Ekuni D, Kurihara H, Putnins EE. Loss of claudin-1 in lipopolysaccharide-treated periodontal epithelium. J Periodontal Res. 2012;47:222–227. doi:10.1111/j.1600-0765.2011.01424.x.
- Groeger S, Doman E, Chakraborty T, Meyle J. Effects of Porphyromonas gingivalis infection on human gingival epithelial barrier function in vitro. Eur J Oral Sci. 2010;118(6):582–589. doi:10.1111/j.1600-0722.2010.00782.x.
- Gröger S, Michel J, Meyle J. Establishment and characterization of immortalized human gingival keratinocyte cell lines. J Periodontal Res. 2008;43(6):604-614. doi:10.1111/jre.2008.43.issue-6.
- Groeger S, Meyle J. Oral Mucosal Epithelial Cells. Front Immunol. 2019;10:208. doi:10.3389/fimmu.2019.00208.
- Belibasakis GN, Kast JI, Thurnheer T, Akdis CA, Bostanci N. The expression of gingival epithelial junctions in response to subgingival biofilms. Virulence. 2015;6(7):704–709. doi:10.1080/21505594.2015.1081731.
- Hatakeyama S, Ishida K, Takeda Y. Changes in cell characteristics due to retinoic acid; specifically, a decrease in the expression of claudin-1 and increase in claudin-4 within tight junctions in stratified oral keratinocytes. J Periodontal Res. 2010;45(2):207–215. doi:10.1111/j.1600-0765.2009.01219.x.
- Zihni C, Balda MS, Matter K. Signalling at tight junctions during epithelial differentiation and microbial pathogenesis. J Cell Sci. 2014;127(16):3401–3413. doi:10.1242/jcs.145029.
- Dickson MA, Hahn WC, Ino Y, Ronfard V, Wu JY, Weinberg RA, Louis DN, Li FP, Rheinwald JG. Human keratinocytes that express hTERT and also bypass a p16(ink4a)-enforced mechanism that limits lifespan become immortal yet retain normal growth and differentiation characteristics. Mol Cell Biol. 2000;20(4):1436–1447. doi:10.1128/MCB.20.4.1436-1447.2000.
- Lindberg K, Rheinwald JG. Three distinct keratinocyte subtypes identified in human oral epithelium by their patterns of keratin expression in culture and in xenografts. Differentiation. 1990;45(3):230–241. doi:10.1111/j.1432-0436.1990.tb00477.x.
- Cheng R, Wu Z, Li M, Shao M, Hu T. Interleukin-1β is a potential therapeutic target for periodontitis: a narrative review. Int J Oral Sci. 2020;12:2–9. doi:10.1038/s41368-019-0068-8.
- Steinberg T, Dannewitz B, Tomakidi P, Hoheisel JD, Müssig E, Kohl A, Nees M. Analysis of interleukin-1β-modulated mRNA gene transcription in human gingival keratinocytes by epithelia-specific cDNA microarrays. J Periodont Res. 2006;41(5):426–446. doi:10.1111/j.1600-0765.2006.00884.x.
- Guo W, Wang P, Liu ZH, Ye P. Analysis of differential expression of tight junction proteins in cultured oral epithelial cells altered by Porphyromonas gingivalis, Porphyromonas gingivalis lipopolysaccharide, and extracellular adenosine triphosphate. Int J Oral Sci. 2018;10(1):e8. doi:10.1038/ijos.2017.51.
- Meyle J, Gültig K, Rascher G, Wolburg H. Transepithelial electrical resistance and tight junctions of human gingival keratinocytes. J Periodontal Res. 1999;34(4):214–222. doi:10.1111/j.1600-0765.1999.tb02244.x.
- Kirschner N, Brandner JM. Barriers and more: functions of tight junction proteins in the skin. Annals of the New York Academy of Sciences. 2012;1257:158-166 1 doi:10.1111/j.1749-6632.2012.06554.x
- Pasparakis M. Regulation of tissue homeostasis by NF-kappaB signalling: implications for inflammatory diseases. Nat Rev Immunol. 2009;9(11):778–788. doi:10.1038/nri2655.
- Choi YS, Kim YC, Jo AR, Ji S, Koo KT, KO Y, Choi Y. Porphyromonas gingivalis and dextran sulfate sodium induce periodontitis through the disruption of physical barriers in mice. Eur J Inflammation. 2013;11(2):419–431. doi:10.1177/1721727X1301100212.
- Kinane DF, Winstanley FP, Adonogianaki E, Moughal NA. Bioassay of interleukin 1 (IL-1) in human gingival crevicular fluid during experimental gingivitis. Arch Oral Biol. 1992;37(2):153–156. doi:10.1016/0003-9969(92)90011-V.
- Stathopoulou PG, Benakanakere MR, Galicia JC, Kinane DF. Epithelial cell pro-inflammatory cytokine response differs across dental plaque bacterial species. J Clin Periodontol. 2010;37:24–29. doi:10.1111/j.1600-051X.2009.01505.x.
- Boch JA, Wara-aswapati N, Auron PE. Interleukin 1 signal transduction - current concepts and relevance to periodontitis. J Dent Res. 2001;80(2):400–470. doi:10.1177/00220345010800020101.
- Delima AJ, Karatzas S, Amar S, Graves DT. Inflammation and tissue loss caused by periodontal pathogens is reduced by interleukin-1 antagonists. J Infect Dis. 2002;186:511–516. doi:10.1086/341778.
- Jennings LR, Colley HE, Ong J, Panagakos F, Masters JG, Trivedi HM, Murdoch C, Whawell S. Development and characterization of in vitro human oral mucosal equivalents derived from immortalized oral keratinocytes. Tissue Engineering: Part C. 2016;22(12):1108–1117. doi:10.1089/ten.tec.2016.0310.
- Park I, Gu H, Park KK. Molecular pathogenesis of bacteria-induced periodontitis. Int J Clin Exp Med. 2018;11:5513–5522.
- Gumbiner B, Simons K. A functional assay for proteins involved in establishing an epithelial occluding barrier: identification of a uvomorulin-like polypeptide. J Cell Biol. 1986;102(2):457–468. doi:10.1083/jcb.102.2.457.
- Schlage WK, Iskandar AR, Kostadinova R, Xiang Y, Sewer A, Majeed S, Kuehn D, Frentzel S, Talikka M, Geertz M, et al. In vitro systems toxicology approach to investigate the effects of repeated cigarette smoke exposure on human buccal and gingival organotypic epithelial tissue cultures. Toxicol Mech Methods. 2014;24(7):470–487. doi:10.3109/15376516.2014.943441.
- Bierbaumer L, Schwarze UY, Gruber R, Neuhaus W. Cell culture models of oral mucosal barriers: A review with a focus on applications, culture conditions and barrier properties. Tissue Barriers. 2018;6(3):1479568. doi:10.1080/21688370.2018.1479568.
- Coisne C, Engelhardt B. Tight junctions in brain barriers during central nervous system inflammation. Antioxid Redox Signal. 2011;15(5):1285–1303. doi:10.1089/ars.2011.3929.
- Engelhardt B. Development of the blood-brain barrier. Cell Tissue Res. 2003;314(1):119–129. doi:10.1007/s00441-003-0751-z.
- Labus J, Häckel S, Lucka L, Danker K. Interleukin-1β induces an inflammatory response and the breakdown of the endothelial cell layer in an improved human THBMEC-based in vitro blood-brain barrier model. J Neurosci Methods. 2014;228:35–45. doi:10.1016/j.jneumeth.2014.03.002.
- Schmitz H, Fromm M, Bentzel CJ, Scholz P, Detjen K, Mankertz J, Bode H, Epple HJ, Riecken EO, Schulzke JD. Tumor necrosis factor-alpha (TNFalpha) regulates the epithelial barrier in the human intestinal cell line HT-29/B6. J Cell Sci. 1999;112:137–146.
- Brandner JM, Schulzke JD. Hereditary barrier-related diseases involving the tight junction: lessons from skin and intestine. Cell Tissue Res. 2015;360:723–748. doi:10.1007/s00441-014-2096-1.
- Pummi K, Malminen M, Aho H, Karvonen SL, Peltonen J, Peltonen S. Epidermal tight junctions: ZO-1 and occludin are expressed in mature, developing, and affected skin and in vitro differentiating keratinocytes. J Invest Dermatol. 2001;117(5):1050–1058. doi:10.1046/j.0022-202x.2001.01493.x.
- Ohnemus U, Kohrmeyer K, Houdek P, Rohde H, Wladykowski E, Vidal S, Horstkotte MA, Aepfelbacher M, Kirschner N, Behne MJ, et al. Regulation of epidermal tight junctions (TJ) during infection with exfoliative toxin-negative Staphylococcus strains. J Invest Dermatol. 2008;128(4):906–916. doi:10.1038/sj.jid.5701070.
- Ward C, Schlingmann B, Stecenko AA, Guidot DM, Koval M. NF-κB inhibitors impair lung epithelial tight junctions in the absence of inflammation. Tissue Barriers. 2015;3(1–2):e982424. doi:10.4161/21688370.2014.982424.
- Dommisch H, Jepsen S. Diverse functions of defensins and other antimicrobial peptides in periodontal tissues. Periodontol 2000. 2015;69:96–110. doi:10.1111/prd.12093.
Appendix A.
Table A1. Primary antibodies used in this study.
Figure A1. Secretion of proinflammatory cytokines in the presence and absence of IL-1β. IL-8 signals obtained by the cytokine array ()) were quantified. Error bars represent the standard deviation and * indicates significant difference between the test and control group (p = .04).
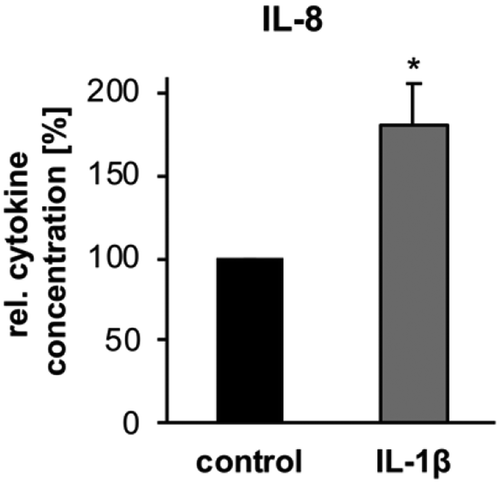
Figure A2. Influence of IL-1β on the morphology of OKG4 cells. Visualization of fluorescence-labeled wheat germ agglutinin (WGA) in OKG4 cells (above) and GECs (below) in control and IL-1β-treated cells. 1.2 × 104 OKG4 cells and GECs were cultured in 8-well chamber slides and stimulated with IL-1β or left untreated for 24 h, respectively. Subsequently, cells were fixed. Membranes were visualized with WGA and cell nuclei were stained with Hoechst 33342. Image processing was performed with ZEN and ImageJ, scale bar, 20 µm.
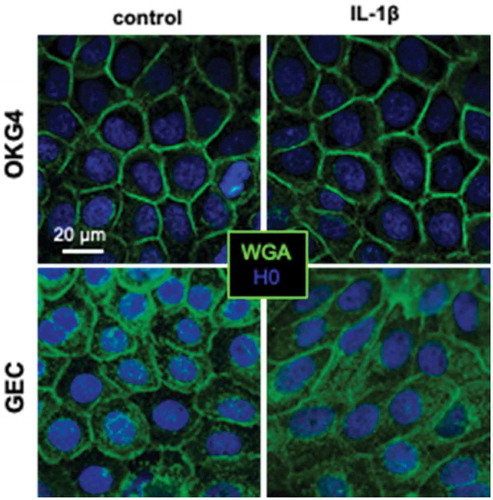
Figure A3. Costaining of ZO-1 and occludin in adult human gingiva.
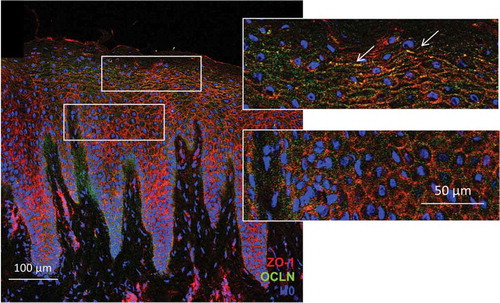
Figure A4. TJ strands are not formed in oral keratinocytes in the presence of a low Ca2+ concentration. 1.2 × 104 cells cultivated in the presence of 60 µM Ca2+ were fixed and stained for claudin-1, ZO-1 and occludin. Image processing was performed with ZEN and ImageJ. Scale bar, 50 µm.
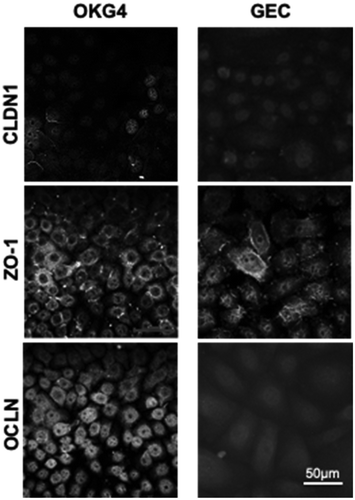
Figure A5. IL-1β increases the expression of occludin and enhances the formation of TJ strands in OKG4 cells. 1.2 × 104 OKG4 cells treated with IL-1β or left untreated for 24 h were fixed and stained for claudin-1, ZO-1 and occludin. Image processing was performed with ZEN and ImageJ. Scale bar, 50 µm.
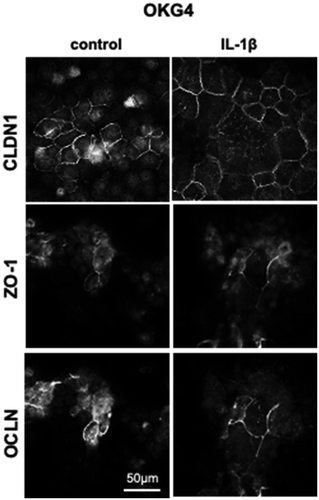