ABSTRACT
Roundabout guidance receptor 4 (Robo4) is an endothelial-specific membrane protein that suppresses pathological angiogenesis and vascular hyperpermeability by stabilizing endothelial cells. Robo4 suppresses severe systemic inflammation induced by pathogens and endotoxins and inhibits tumor growth and metastasis, therefore serving as a potential therapeutic target. Although the regulation of Robo4 expression through transcription factors and epigenetic mechanisms has been studied, the role of histone deacetylases (HDACs) has not been explored. In the present study, we investigated the involvement of HDACs in the regulation of Robo4 expression. An HDAC inhibitor, MS-275, which inhibits HDAC1, HDAC2, and HDAC3, was found to suppress Robo4 expression in endothelial cells. Small interfering RNA (siRNA)-mediated knockdown of HDAC3, but not of HDAC1 and 2, also decreased its expression level. MS-275 downregulated the expression of the transcription factor complex GABP, in addition to suppressing Robo4 promoter activity. GABP expression was also downregulated by the siRNA against HDAC3. MS-275 decreased the transendothelial electrical resistance of a monolayer of mouse endothelial cells and increased the rate of leakage of Evans blue dye in the mouse lungs. In addition, MS-275 accelerated cell migration through the endothelial cell monolayer and augmented cell extravasation in the mouse lungs. Taken together, we demonstrated that MS-275 suppresses Robo4 expression by inhibiting HDAC3 in endothelial cells and enhances endothelial and vascular permeability. Thus, we demonstrated a novel mechanism regulating Robo4 expression and vascular permeability, which is anticipated to contribute to future therapies for infectious and inflammatory diseases.
Introduction
Endothelial cells form a layer on the inner surface of the vasculature. The permeability of blood vessels is regulated by modulation of the VE-cadherin-mediated adherens junction between endothelial cells. Endothelial permeability is regulated by various stimulators, such as vascular endothelial growth factor (VEGF) and tumor necrosis factor α (TNFα). Roundabout guidance receptor 4 (Robo4) is an endothelial-specific membrane protein that stabilizes endothelial cells and the vasculature.Citation1,Citation2 It suppresses vascular hyperpermeability through multiple mechanisms by interacting with different proteins, including Slit2, Unc5B, and TRAF7.Citation3–5 Robo4 improves the survival rate in mouse systemic inflammation models injected with pathogens and endotoxins by suppressing endothelial and vascular hyperpermeability.Citation3,Citation6 Thus, Robo4-mediated regulation of vascular permeability could serve as a potential therapeutic target. It is, therefore, important to understand the mechanisms governing the regulation of Robo4 expression and to devise strategies to regulate its expression.
Robo4 expression is regulated by a 3 kb promoter.Citation7 SP1 and GA-binding protein (GABP), transcription factors that bind to the proximal region of this promoter, are essential for its activity, and thereby for Robo4 expression.Citation7,Citation8 GABP is a heterodimeric complex of proteins consisting of two structurally unrelated subunits: GABPα and GABPβ/γ. GABPα binds to the DNA via the ETS domain, whereas GABPβ and its isoform, GABPγ, interact with GABPα. In addition to the transcription factors, epigenetic mechanisms also regulate Robo4 expression. For example, the methylation pattern of the proximal promoter regulates Robo4 expression.Citation9,Citation10 The components of the polycomb repressor complex 2 (PRC2) binding to the upstream promoter regulate DNA methylation and Robo4 expression.Citation11 These findings implied that other epigenetic factors, such as histone deacetylases (HDACs), may also be involved in the regulation of Robo4 expression in endothelial cells. However, the involvement of such factors has not been studied yet.
HDACs are enzymes that remove acetyl groups from histones and other proteins. Eighteen characterized members of the HDAC superfamily have been identified in humans and are divided into four classes.Citation12 HDACs regulate the acetylation of histones in nucleosomes, which in turn modulates chromatin conformation and gene expression. HDACs also deacetylate non-histone proteins, including transcription factors, and regulate cellular processes under physiological and pathological conditions.
Several inhibitors targeting various combinations of HDAC subtypes have been generated. Certain inhibitors, including the pan-HDAC inhibitor trichostatin A (TSA), have been shown to regulate the expression of endothelial genes and endothelial hyperpermeability in inflammation.Citation13,Citation14 In the present study, we investigated whether Robo4 expression is regulated by HDACs by treating endothelial cells with HDAC inhibitors. We determined that MS-275 suppresses Robo4 expression by inhibiting HDAC3 and enhances vascular permeability. Herein, we demonstrated a hitherto unknown mechanism of HDAC-mediated regulation of Robo4 expression and vascular permeability, and proposed a novel strategy for regulating vascular permeability with small molecules.
Materials and methods
Cell culture
Human umbilical vein endothelial cells (HUVECs) were obtained from Lonza (Basel, Switzerland) and maintained in EGM-2-MV medium (Lonza). The mouse pancreatic endothelial cell line, Mile Sven 1 (MS1), and mouse melanoma cell line, B16-F10, were obtained from the American Type Culture Collection (ATCC; Manassas, VA); they were maintained in Dulbecco’s modified Eagle’s medium (Nacalai Tesque, Kyoto, Japan), supplemented with 10% fetal bovine serum, 100 U/mL penicillin, and 100 µg/mL streptomycin. All the cells were cultured under a humidified atmosphere with 5% CO2 at 37°C.
Quantitative reverse transcription-polymerase chain reaction
For the assay using HDAC inhibitors, HUVECs were treated with 0.3 μM TSA (FUJIFILM, Osaka, Japan), 40 μM TC-H 106 (Tocris, Bristol, UK), 5–20 μM MS-275 (Santa Cruz Biotechnology, Dallas, TX; AdooQ BioScience, Irvine, CA; Carbosynth, Berkshire, UK), 10 μM MC1568 (MedChemexpress, Monmouth Junction, NJ), 100 μM bufexamac (FUJIFILM), or 0.5% dimethyl sulfoxide (DMSO; as a control). MS1 cells were treated with 20 μM MS-275. For the assay using small interfering RNAs (siRNAs), HUVECs (2 × 105 cells) were transfected with 9–30 pmol siRNAs against hHDAC1 (SI00070609; Qiagen, Hilden, Germany), HDAC2 (s6494; Thermo Fisher Scientific, Waltham, MA), hHDAC3 (SI03057901; Qiagen), or their control siRNA (SI03650318; Qiagen) (Table S1). Total RNA from the HDAC inhibitor-treated or siRNA-transfected cells was prepared using the RNeasy Mini Kit (Qiagen) and subsequently reverse-transcribed into complementary DNA (cDNA) using Superscript VILO Master Mix (Invitrogen, Carlsbad, CA). Real-time PCR was performed using cDNA as a template, QuantiTect SYBR Green PCR Kit (Qiagen), and specific primers (Table S1). The copy number of the target transcripts was calculated from standard curves prepared using known amounts of plasmids containing the target sequences. The expression levels of Robo4, SP1, GABP subunits, and HDAC1–3 were normalized against glyceraldehyde 3-phosphate dehydrogenase (GAPDH) levels.
Western blot analysis
Total cell extracts from HUVECs treated with HDAC inhibitors were separated by sodium dodecyl sulfate-polyacrylamide gel electrophoresis, and transferred onto polyvinylidene fluoride membranes. The membranes were incubated with primary antibodies against Robo4 (AF2366; R&D Systems, Minneapolis, MN) or GAPDH (MAB374; Merck Millipore, Burlington, MA) and horseradish peroxidase-conjugated secondary antibodies. Immunoreactive bands were detected using the ImageQuant LAS4010 system (GE Healthcare, Chicago, IL).
Reporter assay
HUVECs (1 × 105 cells) were transfected with 1 µg of a luciferase reporter plasmid containing the Robo4 promoter (pGL3-Robo4Citation7) using FuGENE6 (Promega, Madison, WI) and incubated for 24 h. The transfected cells were then treated with 20 µM MS-275 for 24 h and analyzed by reporter assays using the Dual-Luciferase Reporter Assay system (Promega).
Measurement of transendothelial electrical resistance
MS1 cells (1 × 105 cells) were seeded in cell culture inserts with a pore size of 0.4 µm (BD Falcon, Franklin Lakes, NJ) and incubated for 24 h. The medium in the upper chamber was replaced with fresh medium containing 20 µM MS-275 or DMSO (for control) and transendothelial electrical resistance (TEER) was measured using the CellZscope system (NanoAnalytics, Münster, Germany). Data are expressed for each TEER value (Ω/cm2) of MS-275-treated or DMSO-treated cells normalized to the values at time (t) = 0.
WST-8 assay
MS1 cells (2.5 × 104 cells) were treated with 20 µM MS-275 for 48 h or were transfected with 2.5 pmol of siRNAs against mRobo4 or its control for 48 h and then incubated in a medium containing 10% WST-8 reagent (Dojindo, Kumamoto, Japan) for 1 h. The reaction was terminated by adding 0.1 N HCl and optical density of the samples was measured at 450 nm.
Vascular permeability assay (Miles assay)
Female C57BL/6 N mice (7–8 weeks old) were administered MS-275 (30 mg/kg/day) by oral gavage for four consecutive days. One hour after the final dose, the mice were intravenously injected with PBS containing 1% Evans blue. One hour later, the mice were perfused with PBS containing 2 mM ethylenediaminetetraacetic acid (EDTA) and the lungs were harvested, minced, and incubated in formamide for 48 h. The eluted Evans blue was quantified by measuring the optical density at 620 nm.
Experimental metastasis model
A single-cell suspension of B16-F10 cells was prepared in phosphate-buffered saline (PBS) containing 0.02% EDTA. For the assay using MS-275–treated mice, female C57BL/6 N mice (7–8 weeks old) were administered 200 µL of water containing 1% DMSO and 30% polyethylene glycol 400 with or without MS-275 (30 mg/kg/day) by oral gavage for four consecutive days. On the day of final injection of MS-275, the B16-F10 cell suspension (3 × 105 cells) was intravenously injected into the mice. For the assay using Robo4-deficient mice,Citation3 the same number of B16-F10 cells was intravenously injected into female Robo4-deficient and littermate control mice (7–8 weeks old). After 14 days of the injections, lungs were harvested from these mice, and fixed in Bouin’s solution. The metastasized melanoma colonies on the lung surface were counted under an SZX7 stereo microscope (Olympus, Tokyo, Japan) equipped with an MAS-500 USB camera (Marutsuelec, Tokyo, Japan). All animal experiments were approved by the ethics committee of Osaka University and performed in accordance with the relevant guidelines and regulations.
Transmigration assay
MS1 cells (4 × 104 cells) were seeded in FluoroBlok inserts with a pore size of 8.0 μm (Corning, Corning, NY) and transfected with 10 pmol of siRNA against mRobo4 or LacZ (as a control) (Table S1; Hokkaido System Science, Hokkaido, Japan) using Lipofectamine RNAiMAX (Invitrogen, Carlsbad, CA, USA) or were treated with 20 µM MS-275. Twenty-four hours after the transfection or treatment, B16-F10 cells (2 × 105 cells) labeled with Cellstain Calcein AM solution (Dojindo) were added to the MS1 cells and incubated for 24 h. The transmigrated B16-F10 cells were then counted using a BZ-X700 fluorescence microscope with the BZ-X analyzer software (Keyence, Osaka, Japan).
Statistical analysis
Data are expressed as mean values ± standard error (s.e.m.) and graphically presented as scatter plots. Normality was tested using the Shapiro–Wilk test. For samples with a normal distribution, p-values were calculated using the unpaired t-test and one-way analysis of variance (ANOVA) followed by Bonferroni correction or Dunnett’s test. All statistical analyses were performed using the Prism 9 software (GraphPad Software Inc., San Diego, CA). The statistical significance of differences in the means was determined using the tests indicated in the figure legends. A p-value < 0.05 was considered statistically significant.
Results
HDAC inhibitors suppressed Robo4 expression in endothelial cells
To investigate whether HDACs regulate Robo4 expression in endothelial cells, HUVECs were treated with HDAC inhibitors targeting the different HDAC subtypes. Following this, Robo4 expression was analyzed at both the mRNA and protein levels (). TSA, TC-H 106, and MS-275, which inhibit HDAC1, HDAC2, and HDAC3, respectively, strongly suppressed Robo4 expression; conversely, MC 1568 and Bufexamac, which target other HDACs, resulted in moderate or no effect on Robo4 expression at the mRNA and protein levels. Moreover, MS-275-induced suppression of Robo4 was determined to be dose-dependent (). These results indicated that Robo4 expression is regulated by HDAC1, HDAC2, or HDAC3. To identify the HDAC that primarily regulates Robo4 expression, HUVECs were transfected with siRNAs against HDAC1, HDAC2, and HDAC3. The knockdown of HDAC3, but not of HDAC1 and HDAC2, suppressed Robo4 expression (). This indicated that MS-275 suppresses Robo4 expression by inhibiting HDAC3.
Figure 1. HDAC inhibitors targeting HDAC1-3 suppress Robo4 expression in endothelial cells
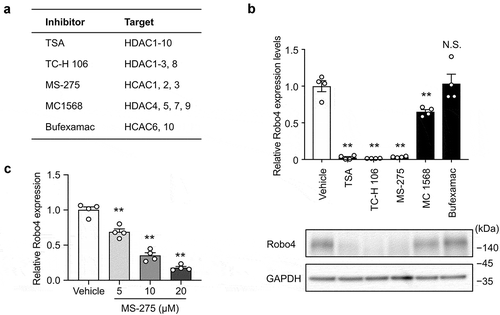
Figure 2. Knockdown of HDAC3 downregulates Robo4 expression in endothelial cells
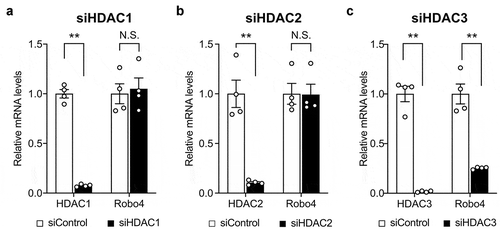
MS-275 suppressed Robo4 promoter activity by downregulating GABP expression
The expression of human Robo4 is regulated by a 3 kb promoter, which is activated by the binding of transcription factors SP1 and GABP to its proximal region (). To investigate whether MS-275 influences Robo4 promoter activity, we analyzed the promoter activity in HUVECs treated with or without MS-275 using a luciferase reporter assay. MS-275 hindered luciferase activity, which indicated that it suppresses Robo4 promoter activity (). We further investigated whether MS-275 regulates the expression of SP1 and GABP using quantitative PCR (). MS-275 suppressed the expression of the GABP subunits but not that of SP1. Similarly, the siRNA against HDAC3 also suppressed the expression of the GABP subunits but not that of SP1 (). Taken together, these results indicated that MS-275 downregulates GABP expression by inhibiting HDAC3 activity and suppresses the activity of the Robo4 promoter.
Figure 3. (a) Schematic illustration of the Robo4 promoter and binding sites of SP1 and GABP in the proximal region.Citation7,Citation8 (b) Effect of MS-275 on Robo4 promoter activity. A reporter plasmid containing the 3 kb Robo4 promoter was transfected into HVUECs treated with or without 20 μM MS-275. Luciferase activity was measured (n = 4). (c, d) Expression of SP1 and GABP subunits in the HUVECs treated with MS-275 (c) or siRNA against HDAC3 (d) was measured by quantitative PCR. Data are expressed as the mean ± s.e.m. Statistical significance was determined by the unpaired t-test (*p < .05, **p < .01; N.S., not significant)
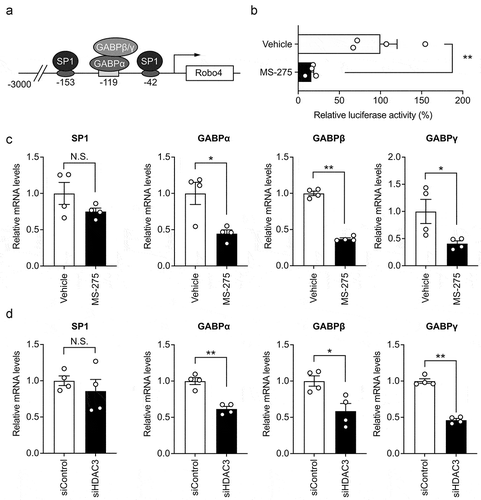
MS-275 enhanced vascular and endothelial permeability
Because Robo4 has been shown to suppress vascular and endothelial permeability, we investigated whether MS-275 impacts vascular permeability. The mice were treated with MS-275 and analyzed for vascular leakage by injecting Evans blue intravenously. MS-275 treatment increased the leakage of Evans blue in the mouse lungs (). Consistent with this result, MS-275 suppressed Robo4 expression in mouse pancreatic endothelial cells (MS1 cells) (), and enhanced the TEER of an MS1 cell monolayer without hampering the viability of these cells ( and d). These results indicated that MS-275 enhances vascular and endothelial permeability.
Figure 4. MS-275 enhances endothelial and vascular permeability
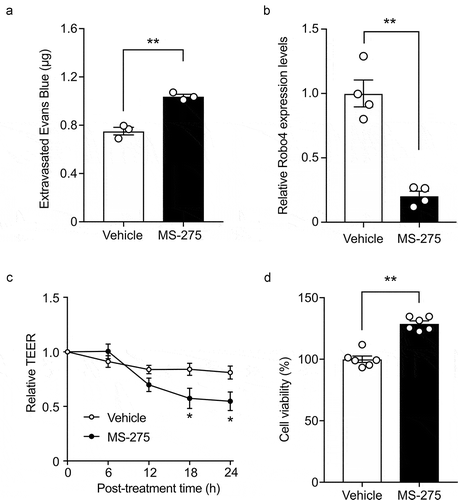
MS-275 enhanced cell migration in an experimental metastasis model
To investigate whether MS-275-induced vascular hyperpermeability regulates cell extravasation from the vasculature, experimental metastasis models were established by intravenously injecting B16-F10 melanoma cells into mice pretreated with or without MS-275. MS-275 treatment increased the number of B16-F10 colonies in the lungs (). Consistent with this in vivo result, treatment of the MS1 cell monolayer with MS-275 enhanced the transendothelial migration of B16-F10 cells without affecting the viability of MS1 cells (). Taken together, these results indicated that MS-275 enhances cell extravasation from the vasculature.
Figure 5. MS-275 promotes the transmigration of cells through the vasculature and endothelial monolayer
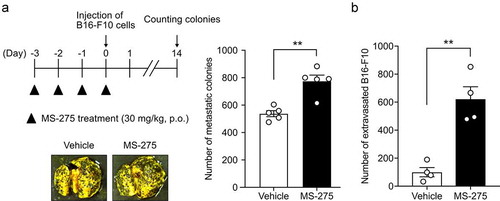
Downregulation of Robo4 enhanced cell extravasation
To investigate whether the downregulation of Robo4 contributes to cell extravasation, the experimental metastasis model was subjected to Robo4-deficient mice. The injection of B16-F10 colonies into Robo4−/− mice resulted in an increased number of colonies in the lungs compared with those in the Robo4+/+ and Robo4−/+ mice (). In the same model, the use of Robo4−/− mice resulted in increased leakage of Evans blue dye in the lungs (). Consistent with this, the siRNA-mediated knockdown of Robo4 in MS1 cells enhanced the transendothelial migration of B16-F10 cells without impacting the viability of MS1 cells ( and d). Taken together, these results indicated that the downregulation of Robo4 enhances endothelial and vascular permeability and cell extravasation.
Figure 6. Downregulation of Robo4 promotes vascular permeability and cell transmigration through the vasculature and endothelial monolayer
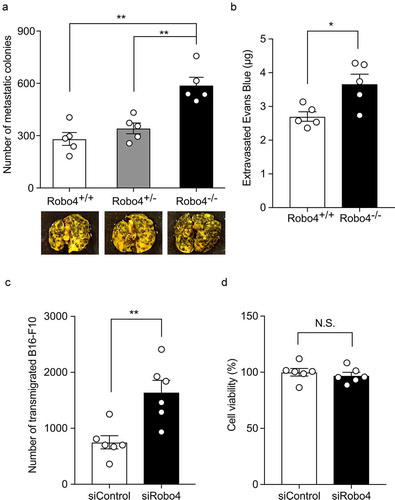
Discussion
Robo4 stabilizes the VE-cadherin-mediated adherens junction and suppresses vascular permeability. It improves the survival of mice under severe infectious and systemic inflammation.Citation3,Citation6 Therefore, an understanding of the mechanism underlying the regulation of Robo4 expression and devising a strategy for controlling its expression is expected to be useful for the development of future therapy. In the present study, we investigated the effects of HDAC inhibitors on Robo4 expression. We demonstrated that MS-275 suppresses Robo4 expression and induces endothelial hyperpermeability and cell extravasation. Mechanistically, MS-275-mediated inactivation of HDAC3 suppressed Robo4 expression by downregulating the expression of GABP. Thus, we successfully demonstrated a novel mechanism for the regulation of Robo4 expression by HDAC and its inhibitor and proposed a strategy for controlling vascular permeability using small molecules.
MS-275 inhibits the activity of HDAC1, HDAC2, and HDAC3, which are ubiquitously expressed in tissues.Citation15 HDAC1 and HDAC2 have almost identical protein structures that contain tandem phosphorylation sites and catalytic domains for deacetylation activity, and are localized only in the nucleus.Citation16 In contrast, HDAC3 contains only one phosphorylation site and shuttles between the nucleus and the cytoplasm using the nuclear export signal.Citation17 HDAC3 is exclusively recruited to the SMRT/NCoR complex, whereas HDAC1 and 2 are found in multiple corepressor complexes.Citation16 Thus, HDAC3 possesses a unique structure and function compared with HDAC1 and HDAC2. Consistent with this fact, siRNA-mediated knockdown of HDAC3, but not of HDAC1 and 2, was observed to suppress Robo4 expression by downregulating the expression of GABP, indicating a unique function of HDAC3 in endothelial cells. However, it remains to be answered as to how MS-275 downregulates GABP by inhibiting the HDAC3 activity. In a previous study, it was suggested that GABPα expression is regulated by a protein complex containing GABP and SP1.Citation18 In addition, physical and functional interactions between SP1 and HDAC3 in gene regulation have been reported.Citation19,Citation20 These findings indicated the possibility that GABPα expression is regulated by the SP1–HDAC3 complex and that MS-275 downregulates Robo4 expression by inhibiting the functioning of this complex. However, there are relatively few studies demonstrating the mechanisms for the regulation of GABP. The MS-275- and HDAC3-mediated gene regulation of GABP needs to be investigated in the future.
MS-275 has been shown to regulate the expression of both pro- and anti-inflammatory genes.Citation21 It activates NF-κB by augmenting its acetylation and nuclear translocation.Citation22 MS-275 also exerts an anti-tumor effect by targeting tumor and immune cells. In the present study, we identified endothelial cells as the novel target cell type for MS-275 and unraveled a unique effect of MS-275 on vascular permeability. We used an experimental metastasis model to readily evaluate the effect of MS-275 treatment on cell extravasation. However, this does not elucidate the effect of MS-275 on autonomous tumor metastasis because this model does not completely reproduce all the processes involved in metastasis, including the migration of tumor cells from the primal tumor into the blood. In fact, the anti-metastasis effect of MS-275 was demonstrated in previous studies on the spontaneous metastasis model (subcutaneous injection model).Citation23,Citation24 In addition, we have previously shown that downregulation of Robo4 increases the transmigration of monocytic cells through the endothelial cell monolayer,Citation3 indicating that MS-275 also promotes the extravasation of immune cells, which may contribute to the anti-tumor effect of MS-275. Furthermore, a recent study showed that Robo4 regulates the expression of α-SMA, Snail, and VE-cadherin, implying that Robo4 modulates endothelial–mesenchymal transition, which is associated with tumor metastasis.Citation25,Citation26 Further investigations on the effect of MS-275 on tumor metastasis are warranted.
In summary, we have successfully demonstrated a novel mechanism for the regulation of Robo4 expression and vascular permeability via HDAC and its inhibitors. The results presented herein would be useful for developing therapies against severe infectious and systemic inflammatory diseases in the future by targeting vascular permeability.
Notes on contributors
T. Kashio, K. Shirakura, M. Kinoshita, N. Hino, S. Koyama, R., Suzuki, Y. Yoshioka, T. Aoshi, T. Doi, and Y. Okada designed and conceived the study. T. Kashio, M. Kinoshita, M. Morita, R. Ishiba, K. Muraoka, T. Kanbara, M. Tanaka, and R. Funatsu performed the experiments. T. Kashio, K. Shirakura, M. Kinoshita, N. Hino, S. Koyama, R. Suzuki, Y. Yoshioka, T. Aoshi, T. Doi, and Y. Okada interpreted the data. T. Kashio, K. Shirakura, and Y. Okada wrote the manuscript.
Supplemental Material
Download MS Word (33.6 KB)Disclosure statements
Y. Yoshioka is employed by the Research Foundation for Microbial Diseases of Osaka University. The remaining authors declare no conflicts of interest.
Supplementary material
Supplemental data for this article can be accessed on the publisher’s website.
Additional information
Funding
References
- Huminiecki L, Gorn M, Suchting S, Poulsom R, Bicknell R. Magic roundabout is a new member of the roundabout receptor family that is endothelial specific and expressed at sites of active angiogenesis. Genomics. 2002;79(4):1–11. doi:https://doi.org/10.1006/geno.2002.6745.
- Park KW, Morrison CM, Sorensen LK, Jones CA, Rao Y, Chien CB, Wu JY, Urness LD, Li DY. Robo4 is a vascular-specific receptor that inhibits endothelial migration. Dev Biol. 2003;261(1):251–267. doi:https://doi.org/10.1016/S0012-1606(03)00258-6.
- Shirakura K, Ishiba R, Kashio T, Funatsu R, Tanaka T, Fukada SI, Ishimoto K, Hino N, Kondoh M, Ago Y, et al. The Robo4-TRAF7 complex suppresses endothelial hyperpermeability in inflammation. J Cell Sci. 2019;132(1):jcs220228. doi:https://doi.org/10.1242/jcs.220228.
- Koch AW, Mathivet T, Larrivee B, Tong RK, Kowalski J, Pibouin-Fragner L, Bouvrée K, Stawicki S, Nicholes K, Rathore N, et al. Robo4 maintains vessel integrity and inhibits angiogenesis by interacting with UNC5B. Dev Cell. 2011;20(1):33–46. doi:https://doi.org/10.1016/j.devcel.2010.12.001.
- Jones CA, London NR, Chen H, Park KW, Sauvaget D, Stockton RA, Wythe JD, Suh W, Larrieu-Lahargue F, Mukouyama Y-S, et al. Robo4 stabilizes the vascular network by inhibiting pathologic angiogenesis and endothelial hyperpermeability. Nat Med. 2008;14(4):448–453. doi:https://doi.org/10.1038/nm1742.
- London NR, Zhu W, Bozza FA, Smith MC, Greif DM, Sorensen LK, Chen L, Kaminoh Y, Chan AC, Passi SF, et al. Targeting Robo4-dependent slit signaling to survive the cytokine storm in sepsis and influenza. Sci Transl Med. 2010;2(23):23ra19. doi:https://doi.org/10.1126/scitranslmed.3000678.
- Okada Y, Yano K, Jin E, Funahashi N, Kitayama M, Doi T, Spokes K, Beeler DL, Shih S-C, Okada H, et al. A three-kilobase fragment of the human Robo4 promoter directs cell type-specific expression in endothelium. Circ Res. 2007;100(12):1712–1722. doi:https://doi.org/10.1161/01.RES.0000269779.10644.dc.
- Okada Y, Jin E, Nikolova-Krstevski V, Yano K, Liu J, Beeler D, Spokes K, Kitayama M, Funahashi N, Doi T, et al. A GABP-binding element in the Robo4 promoter is necessary for endothelial expression in vivo. Blood. 2008;112(6):2336–2339. doi:https://doi.org/10.1182/blood-2008-01-135079.
- Okada Y, Funahashi N, Tanaka T, Nishiyama Y, Yuan L, Shirakura K, Turjman AS, Kano Y, Naruse H, Suzuki A, et al. Endothelial cell-specific expression of roundabout 4 is regulated by differential DNA methylation of the proximal promoter. Arterioscler Thromb Vasc Biol. 2014;34(7):1531–1538. doi:https://doi.org/10.1161/ATVBAHA.114.303818.
- Tanaka T, Izawa K, Maniwa Y, Okamura M, Okada A, Yamaguchi T, Shirakura K, Maekawa N, Matsui H, Ishimoto K, et al. ETV2-TET1/TET2 complexes induce endothelial Cell-Specific Robo4 expression via promoter demethylation. Sci Rep. 2018;8(1):5653. doi:https://doi.org/10.1038/s41598-018-23937-8.
- Izawa K, Shirakura K, Kakiuchi K, Funahashi N, Maekawa N, Hino N, Tanaka T, Doi T, Okada Y. PRC2 components maintain DNA hypermethylation of the upstream promoter and regulate Robo4 expression in endothelial cells. Biol Pharm Bull. 2020;43(4):742–746. doi:https://doi.org/10.1248/bpb.b19-01014.
- Seto E, Yoshida M. Erasers of histone acetylation: the histone deacetylase enzymes. Cold Spring Harb Perspect Biol. 2014;6(4):a018713. doi:https://doi.org/10.1101/cshperspect.a018713.
- Adcock IM. HDAC inhibitors as anti-inflammatory agents. Br J Pharmacol. 2007;150(7):829–831. doi:https://doi.org/10.1038/sj.bjp.0707166.
- Kovacs L, Kovacs-Kasa A, Verin AD, Fulton D, Lucas R, Su Y. Histone deacetylases in vascular permeability and remodeling associated with acute lung injury. Vessel Plus. 2018;2:2. doi:https://doi.org/10.20517/2574-1209.2018.06.
- Wang Y, Stowe RL, Pinello CE, Tian G, Madoux F, Li D, Zhao L, Li J-L, Wang Y, Wang Y, et al. Identification of histone deacetylase inhibitors with benzoylhydrazide scaffold that selectively inhibit class I histone deacetylases. Chem Biol. 2015;22(2):273–284. doi:https://doi.org/10.1016/j.chembiol.2014.12.015.
- Yang XJ, Seto E. The Rpd3/Hda1 family of lysine deacetylases: from bacteria and yeast to mice and men. Nat Rev Mol Cell Biol. 2008;9(3):206–218. doi:https://doi.org/10.1038/nrm2346.
- Yang WM, Tsai SC, Wen YD, Fejer G, Seto E. Functional domains of histone deacetylase-3. J Biol Chem. 2002;277(11):9447–9454. doi:https://doi.org/10.1074/jbc.M105993200.
- Patton J, Block S, Coombs C, Martin ME. Identification of functional elements in the murine Gabp alpha/ATP synthase coupling factor 6 bi-directional promoter. Gene. 2006;369:35–44. doi:https://doi.org/10.1016/j.gene.2005.10.009.
- Huang W, Tan D, Wang X, Han S, Tan J, Zhao Y, Lu J, Huang B. Histone deacetylase 3 represses p15(INK4b) and p21(WAF1/cip1) transcription by interacting with Sp1. Biochem Biophys Res Commun. 2006;339(1):165–171. doi:https://doi.org/10.1016/j.bbrc.2005.11.010.
- Kim Y, Kim K, Park D, Lee E, Lee H, Lee YS, Choe J, Jeoung D. Histone deacetylase 3 mediates allergic skin inflammation by regulating expression of MCP1 protein. J Biol Chem. 2012;287(31):25844–25859. doi:https://doi.org/10.1074/jbc.M112.348284.
- Leus NG, Van Den Bosch T, Van Der Wouden PE, Krist K, Ourailidou ME, Eleftheriadis N, Kistemaker LEM, Bos S, Gjaltema RAF, Mekonnen SA, et al. HDAC1-3 inhibitor MS-275 enhances IL10 expression in RAW264.7 macrophages and reduces cigarette smoke-induced airway inflammation in mice. Sci Rep. 2017;7(1):45047. doi:https://doi.org/10.1038/srep45047.
- Dai Y, Rahmani M, Dent P, Grant S. Blockade of histone deacetylase inhibitor-induced RelA/p65 acetylation and NF-kappaB activation potentiates apoptosis in leukemia cells through a process mediated by oxidative damage, XIAP downregulation, and c-Jun N-terminal kinase 1 activation. Mol Cell Biol. 2005;25(13):5429–5444. doi:https://doi.org/10.1128/MCB.25.13.5429-5444.2005.
- Rao-Bindal K, Koshkina NV, Stewart J, Kleinerman ES. The histone deacetylase inhibitor, MS-275 (entinostat), downregulates c-FLIP, sensitizes osteosarcoma cells to FasL, and induces the regression of osteosarcoma lung metastases. Curr Cancer Drug Targets. 2013;13(4):411–422. doi:https://doi.org/10.2174/1568009611313040005.
- Srivastava RK, Kurzrock R, Shankar S. MS-275 sensitizes TRAIL-Resistant breast cancer cells, inhibits angiogenesis and metastasis, and reverses Epithelial-Mesenchymal transition in vivo. Mol Cancer Ther. 2010;9(12):3254–3266. doi:https://doi.org/10.1158/1535-7163.MCT-10-0582.
- Gould RA, Aziz H, Woods CE, Seman-Senderos MA, Sparks E, Preuss C, Wünnemann F, Bedja D, Moats CR, McClymont SA, et al. ROBO4 variants predispose individuals to bicuspid aortic valve and thoracic aortic aneurysm. Nat Genet. 2019;51(1):42–50. doi:https://doi.org/10.1038/s41588-018-0265-y.
- Gurzu S, Turdean S, Kovecsi A, Contac AO, Jung I. Epithelial-mesenchymal, mesenchymal-epithelial, and endothelial-mesenchymal transitions in malignant tumors: an update. World J Clin Cases. 2015;3(5):393–404. doi:https://doi.org/10.12998/wjcc.v3.i5.393.