ABSTRACT
The oral cavity is directly exposed to a variety of environmental stimuli and contains a diverse microbiome that continuously interacts with the oral epithelium. Therefore, establishment and maintenance of the barrier function of the oral mucosa is of paramount importance for its function and for the body’s overall health. The adherens junction is a cell–cell adhesion complex that is essential for epithelial barrier function. Although a considerable body of work has associated barrier disruption with oral diseases, the molecular underpinnings of these associations have not been equally investigated. This is critical, since adherens junction components also possess significant signaling roles in the cell, in addition to their architectural ones. Here, we summarize current knowledge involving adherens junction components in oral pathologies, such as cancer and oral pathogen-related diseases, while we also discuss gaps in the knowledge and opportunities for future investigation of the relationship between adherens junctions and oral diseases.
Introduction: anatomy and tissue composition of the oral mucosa
The oral cavity serves as the entry point for the digestive system. As a part of the body directly exposed to environmental cues, to nutrients, and to pathogens, it plays critical roles in health and homeostasis. Anatomically, the oral cavity begins with the lips, which open to the vestibule, the area between the cheeks, teeth, and lips (Box 1; ). The main area of the oral cavity, or oral cavity proper, consists of the tongue and the alveolar processes containing the teeth. Anteriorly, the roof forms by the hard palate and posteriorly by the soft palate (). The oral cavity is protected throughout by a mucous membrane known as the oral mucosa, which is composed of stratified squamous epithelium and constitutes the inner lining of the mouth (Box 1; ).
Figure 1. Anatomy and structure of the oral cavity. The type of tissue is indicated in parentheses; key is given at the bottom left of the figure.
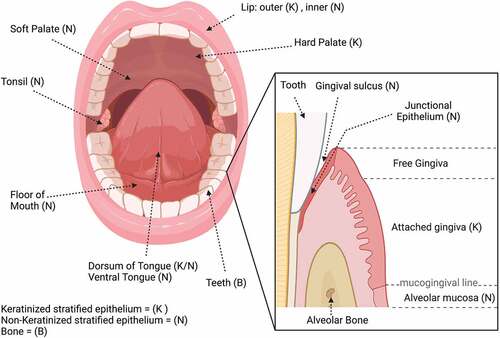
The oral epithelium serves three main, essential functions, which are: a) to form a physical barrier that provides protection from invading pathogens and physiological stress; b) to provide sensation, particularly taste sensation; and c) to secrete salivary fluid to aid in digestion. The oral epithelium undergoes continuous cell shedding and is maintained by cell division, which results in renewal of the epithelial layerevery 14–21 days. Underneath the stratified layer is an underlying lamina propria, which consists mainly of collagen fibers, some elastic fibers of extracellular matrix (ECM), and fibroblasts. The gingiva in particular is the combined epithelial and connective tissue forming a masticatory mucosa attached to the teeth and alveolar bone (; ). Submandibular and sublingual salivary glands secrete fluids that keep the oral cavity moistCitation1–3 and help form an additional barrier that can aid in preventing pathogens from affecting the epithelium (; ).
Situated in the central portion of the oral cavity, the tongue is important in mastication, or chewing to form a bolus of food, and also assists in swallowing. The tongue consists of stratified squamous epithelium and is attached to the floor of the mouth via a folded structure, called the frenulum.Citation4 The ventral portion of the tongue consists of stratified squamous, non-keratinized epithelium, while the dorsum of the tongue is covered by a specialized mucosa that can be either keratinized or non-keratinizedCitation2 (). Taste bud papillae are found predominantly on the tongue and palate. There are three types of papillae: a) fungiform located on the anterior portion; b) circumvallate, which are located on the posterior part of the tongue; and c) foliate papillae located on the lateral sides of the tongue.Citation5
Box 1. Explanation of oral terminology included in the Review.
The oral mucosa is overall classified into three types: lining, masticatory, and the specialized mucosa, which is located on the dorsum of the tongue. The lining mucosa is found on the soft palate, cheeks, inner lips, alveolar mucosa, the floor of the mouth, and the vestibule (; ). The lining mucosa consists of non-keratinized stratified squamous epithelium, which composes the majority of the epithelium. The masticatory mucosa, found on the hard palate and gingiva, is covered by stratified squamous parakeratinized or orthokeratinized epithelium, providing the structures protection from abrasion during the process of mastication (; ).
The gingival epithelium has been extensively studied due to its implication in periodontal disease. The gingival epithelium is composed of keratinizing stratified epithelium and covers the external surface of the gingiva, which surrounds the teeth ().Citation6 An important function of the gingival epithelium is to provide the first line of defense in the oral cavity against pathogens.Citation7 There are several components of the gingiva. The attached gingiva is firmly bonded to the connective tissue over the alveolar bone and is separated from the oral mucosa by the mucogingival line (). The attached gingiva is located apically to the gingival sulcus, which is the space surrounding each tooth and is lined by the oral sulcular epithelium (Box 1; ). This epithelium is stratified and non-keratinized.Citation2 The junctional epithelium is a specialized epithelial component. This epithelium is derived from the enamel epithelium and mediates the attachment of the gingival epithelium to the enamel on the tooth surface ().Citation8 This is an important function of the junctional epithelium, because it forms a barrier against pathogens passing from the oral cavity into the tissue that supports the tooth.Citation9 Due to its role, the junctional epithelium isan area of extensive study in periodontal disease,Citation10 where tooth mobility and loss are found. In comparison to the gingival epithelium, the junctional epithelium shows no keratinization and exhibits much higher proliferation and turnover rateCitation2,Citation7,Citation8 ().
Different areas within the oral cavity contain different cell types and “habitats”, which allow different pathologies to arise.Citation11 Besides oral keratinocytes, other cells, such as Langerhans cells, melanocytes, and Merkel cells can be found in the oral cavity, as well as transient inflammatory migrant cells. Importantly, numerous microbes are present in the oral cavity, both commensally and pathogenically. This brings up the importance of cell–cell junctions, as they are responsible for the formation of the mucosal barrier that protects against invasion of pathogens.Citation2,Citation12 Cell–cell junctional complexes include tight junctions, gap junctions, desmosomes, and adherens junctions, which are present in all oral mucosal cells.Citation13–21 In this review, we focus on the role of adherens junctions in oral health and disease.
The Adherens Junction
Adherens junctions (AJs) are fundamental cell–cell adhesion structures, typically located at apical areas of cell–cell contact in polarized differentiated epithelia, between tight junctions (TJs), which lie more apically, and desmosomes, found more basolaterally.Citation18,Citation22,Citation23 The intimate crosstalk of the AJs with the TJs is essential for formation of the epithelial barrier.Citation24–26 In stratified epithelial tissues, such as those lining the oral mucosa, TJs are exclusively present at the stratum granulosum, where the barrier is eventually established.Citation2,Citation13,Citation18,Citation22,Citation27 Nevertheless, AJs, as well as desmosomes, are also interspersed throughout areas of cell–cell contact in the underlying layers of the stratified epithelium, where they play critical roles for tissue organization, tissue stratification, and mechanical stability.Citation18,Citation22,Citation23 Overall, AJs are abundant throughout most of the oral mucosal epithelium, as well as the gingival epithelium and sulcular epithelium. Relatively fewer AJs, as well as fewer desmosomes, are found in the junctional epithelium, since increased permeability and wide intercellular spaces are desired there to allow passage of immune cells, such as neutrophils and leucocytes that protect the periodontal area from pathogens.Citation2,Citation6,Citation8
The core composition of the AJ includes members of the classical cadherin and the catenin protein families. The cornerstone AJ molecule in epithelial tissues is E-cadherin, a transmembrane glycoprotein. Stratified epithelia also contain P-cadherin at their basal layers.Citation22,Citation28,Citation29 E-cadherin possesses five extracellular domains, which form calcium-dependent interactions both with cadherin molecules of the same cell (cis) and with cadherin molecules of adjacent cells (trans).Citation21,Citation30,Citation31 Both types of cadherin interactions are critical to establish cell–cell adhesions. Intracellularly, E-cadherin binds through its juxtamembrane domain to p120 catenin (p120), and through its catenin-binding domain to β-catenin. p120 regulates the stability and turnover of the AJ complex, whereas β-catenin binds to α-catenin, which establishes connections with the actin cytoskeletonCitation21,Citation30,Citation31 (). In addition to the core cadherin-catenin complex, the AJs are supported by the nectin-afadin complex.Citation32 Like cadherins, nectins are also transmembrane proteins that form intercellular bonds, whereas they bind intracellularly to afadin, which in turn establishes additional connections with the actin cytoskeletonCitation32 (). This cross-talk of the AJs with the actin cytoskeleton is critical for establishing and maintaining cell and tissue architecture, through regulating epithelial cell linkage and dynamics, and through sensing and responding to mechanical stressesCitation31,Citation33–38. During development of stratified epithelia in particular, tensile actin cables form apically first, followed by expansion of a network of actin fibers throughout the epidermal sheet in a Rho/ROCK-dependent manner, which enables cell movement and further formation of cell–cell junctions, eventually generating a stratified tissue.Citation39
Figure 2. Effects of oral pathogens on adherens junctions (AJs) and related phenotypic outcomes. Key is inlcuded in the box on the top right. Cdt is the cytolethal distending toxin.
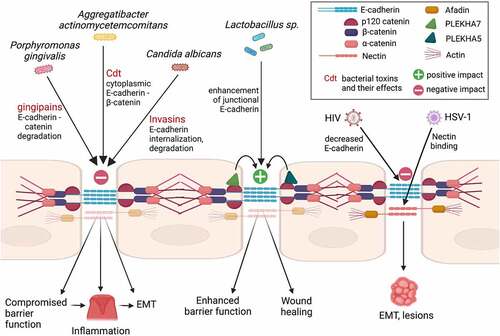
Beyond their structural roles, a large body of work has unraveled numerous signaling functions of AJ components, both in normal physiological conditions and in several diseases, such as cancer.Citation30 A well-studied phenomenon with major implications in tumorigenesis is the so-called “cadherin switching”, which is the hallmark event during the Epithelial-to-Mesenchymal Transition (EMT). During cadherin switching, E-cadherin is downregulated and replaced by mesenchymal cadherins, such as N-cadherin or Cadherin-11. Mesenchymal cadherins overall promote cell migration and metastasis of tumor cells.Citation30,Citation40–42
In addition to cadherin switching, AJ components can influence cell behavior and/or promote disease, either upon AJ disruption or even when E-cadherin is present.Citation30,Citation43 For example, in one of its most-studied roles, β-catenin translocates to the nucleus upon disruption of AJs and acts as a transcriptional activator of the Wnt signaling pathway.Citation21,Citation30 The Wnt signaling pathway plays fundamental roles during development. In particular, excess β-catenin maintains proliferation of keratinocyte stem cells in stratified epithelia.Citation44 However, aberrant activation of Wnt signaling through β-catenin due to AJ disruption in differentiated epithelia, promotes tumorigenesis, especially when additional mutations in the Wnt pathway are introduced.Citation45 AJ disruption through loss of α-catenin has also been shown to promote cell growth and proliferation in stratified epithelia, through activation of the Hedgehog and Hippo pathways, which are otherwise suppressed by α-catenin, e.g., though retention of the transcription factor Yap1.Citation46–48 p120 has also been shown to function as a signaling regulator in several ways. For example, p120 has been identified as an EGFR – Src phosphorylation substrate; p120 phosphorylation by EGFR or Src results in AJ destabilization and promotes cell growth.Citation49–51 Recent work has actually identified a direct association of EGFR with E-cadherinCitation52 and has demonstrated a critical role of EGFR in the fine-tuning of stratified epithelial polarization and barrier function by E-cadherin.Citation24 AJ disruption can also result in translocation of p120 to the nucleus, where it acts as a tumor promoter through sequestering of the tumor suppressing transcription factor Kaiso.Citation53–56 Finally, p120 can promote actin reorganization and cell migration, as well as anchorage-independent growth, through suppressing of the RhoA-ROCK pathway.Citation55,Citation57–59 Counteracting the pro-growth activities of p120, PLEKHA7 (Pleckstrin homology domain containing A7) is a p120 and afadin protein partner that interacts with the microtubule and actin cytoskeleton and has been shown to support barrier function and to exert tumor-suppressing functions, through recruiting of the RNA interference (RNAi) machinery and miRNAs at AJs.Citation60–69
The above highlight the numerous functions of AJ components in epithelial cells and tissues and demonstrate that: a) they are key structural elements, regulating tissue maintenance, barrier function, and responses to mechanical and environmental stimuli; and b) they play critical roles in regulating cellular behavior by being in the “driver’s seat” in numerous pathways. In the following paragraphs, we discuss these regulatory functions of AJs in the context of the oral epithelial homeostasis and disease.
Microbe-oral epithelium interactions and AJs
The human mouth is consistently exposed to various microbes through its exposure to the external environment. The oral cavity is one of the most bacterially diverse sites in the body, harboring more than 700 bacterial species.Citation70 Diet, developmental stage, and decline of immunity due to infection or aging, can contribute to a changing oral microbiome.Citation71 It is well known that various microbes in the oral cavity interact with cell–cell junctions and can alter barrier functionality, by either improving protection for the host or triggering disease.Citation2,Citation72 Bacteria are implicated in common oral diseases, such as gingivitis, periodontitis, and caries (Box 1). A disruption of epithelial cell–cell adhesion, known as “leaky gum” disease, is associated with the progression of periodontal disease.Citation73 For example, it has been noted that there is a significant reduction in the expression of E-cadherin in the oral mucosa of patients with gingivitis or periodontal disease, compared to healthy individuals. The significant reduction in E-cadherin levels in periodontal disease could be a result of periodontal pathogens and can indicate a compromised barrier functionality.Citation74 Pathogens that have been identified to alter the AJs or associated structures are discussed here.
Porphyromonas gingivalis
Porphyromonas gingivalis is a well-studied oral pathogen that has been implicated in periodontitis, an inflammatory condition of the oral epithelium. Infiltration of the gingival barrier by P. gingivalis can trigger an inflammatory reaction in the epithelium,Citation75 consequentially leading to the invasion of more bacterial species, as well as to ultimate bone loss and exfoliation of the dentition. P. gingivalis infections promote increased protein and mRNA expression of key EMT-promoting transcription factors, such as SLUG, SNAI1, and ZEB1 in the oral epithelium.Citation76 As a result, E-cadherin expression and membrane localization is decreased in oral epithelial cellsCitation76 (). Overall, P. gingivalis inhibits both cell-to-cell adhesion and cell-to-matrix adhesion of oral keratinocytesCitation9 and negatively impacts both TJs and AJs,Citation2 through secreting a proteolytic factor called gingipains (). It has been shown that in oral keratinocytes, gingipains target catenins, which are essential for AJ stability, causing AJ dissolution.Citation9 Mechanistically, it has also been shown that P. gingivalis gingipains can degrade E-cadherin, in order to invade the mucosal tissue, contributing to the progression of periodontal disease.Citation75,Citation77 However, it is unknown whether this disruption is the mechanism by which there is detachment of healthy tissue leading to periodontal disease, or if this simply exacerbates already compromised tissue, leaving this an open field of investigation.
Aggregatibacter actinomycetemcomitans
Aggregatibacter actinomycetemcomitans, is another bacterial species that, in addition to P. gingivalis, has been shown to decrease E-cadherin levels and alter its cytosolic distributionCitation78 (). A. actinomycetemcomitans is a Gram-negative facultative anaerobe that is prevalent in 90% of localized aggressive periodontitis and in 40% of severe adult periodontal cases.Citation79 One virulence factor that allows this pathogen to have significant effects on the cell cycle is the Cytolethal distending toxin (Cdt) (). This toxin has been shown to cause extensive damage to the gingival tissue.Citation80 Exposure of gingiva to the toxin results in increased cytosolic distribution of E-cadherin, accompanied by an increase in both β-catenin and β-actin levels,Citation14 indicating AJ remodeling and eventually negatively affecting barrier integrity. It has been shown that these effects on E-cadherin, the gingival barrier, and the concomitant inflammation caused by A. actinomycetemcomitans, are alleviated by the use of Irsogladine maleate (IM), as well as by inhibiting p38 and ERK phosphorylation.Citation81 These studies indicate that changes in the integrity of gingival cells in periodontal disease may be specifically due to AJ alternations. Indeed, it seems that the negative effects of Cdt to AJs contribute to compromised cell–cell adhesion and barrier function and could be a mechanism of interest in the progression of periodontal disease.
Lactobacillus sp. and other probiotic bacterial species
Contrary to species, such as P. gingivalis and A. actinomycetemcomitans, other microbes have a positive effect on cell–cell adhesion. Probiotics are a popular area of study for treating intestinal epithelial barrier function and several microbes are under investigation in this context. One such genus of bacteria that has probiotic effects are Lactobacilli sp. This genus includes Lactobacillus acidophilus, Lactobacillus fermentum, Lactobacillus gasseri, Lactobacillus rhamnosus, and Lactobacillus reuteri. Lactobacillus sp. are Gram-positive rod-shaped bacteria found in fermented foods, and have in recent years been thought to have a potential in intervening in pathogenic infections.Citation82 In the body, L. acidophilus is commonly found in the mouth, and it is thought that Lactobacilli found in the GI system can originate in the oral cavity.Citation83 Lactobacillus sp. have been shown to enhance E-cadherin localization at areas of cell–cell contact,Citation84 as well as E-cadherin overall expression at the mRNA and protein levelsCitation72,Citation84 (). In addition, Lactobacillus sp. increase transepithelial resistance (TEER) of intestinal epithelial cells,Citation84 which reinforces the idea that probiotics enhance epithelial barrier function (). These Lactobacillus sp. might do this through a Lactobacillus-dependent phosphorylation of β-catenin by PKC Ser/Thr protein kinases at the cell membrane,Citation84 strengthening the E-cadherin/β-catenin complex. In addition, it has been noted that Lactobacillus sp. have the ability to intervene in colorectal cancer progression through the Wnt/β-catenin signaling pathway.Citation85 Looking at the oral cavity, Lactobacillus reuteri extracts accelerated the process of wound healing in mouse gingiva via the PI3K/AKT/β-catenin/TGFβ1 pathway.Citation86 However, it is important to note that Lactobacillus sp. are considered opportunistic pathogens and are commonly associated with dental caries, due to the characteristic virulent production of lactic acid.Citation87,Citation88 Therefore, an area of future study would be comparing the effects of all Lactobacillus sp. on oral epithelial cells and intestinal epithelium and determining the potential impact on AJs and associated proteins in different locations. In addition, it is worth further looking into the oral-gut microbiome interplay.
Candida albicans
In addition to bacterial-oral interactions, fungal species, such as Candida albicans, also contribute to infections of the oral mucosa. Candidiasis, or oral yeast infection, occurs as an opportunistic infection when there is an increase in accumulation of C. albicans, leading to appearance of white patches, or thrush. A study has shown that C. albicans produces proteinases, such as Sap5p, that promote degradation of E-cadherin, leading to infiltration of AJs and resulting in further invasion of C. albicans into oral epithelial tissuesCitation89 (). In what seems counterintuitive, another study showed that C. albicans increased E-cadherin – mediated cell–cell adhesion between oral keratinocytes within 3–6 hours after incubation, which was accompanied by the presence of actin stress fibers. Still, after 12 hours of incubation, cell–cell adhesions began to disintegrate due to reduction in E-cadherin levels.Citation90 Another study looked at a synergistic effect between biofilm partners C. albicans and Streptococcus oralis, where both synergistically work to degrade E-cadherin in oral epithelial cells through the exploitation of epithelial calpain, a protease that causes proteolytic cleavage of E-cadherin.Citation91 It has further been shown that Ssa1 and Als3 invasion, which bind to host cell receptors such as E-cadherin and subsequently induce host cells to endocytose the organism, cooperatively contribute to AJ penetration by binding to not only E-cadherin, but also to HER2/EGFR cell receptors in the intestineCitation92 (). This implies that the AJ might be a target for intervention in preventing further pathogenicity of the microbe, and that further studies should be conducted on the mechanisms through which Candida affects junctions and their associated proteins.
Viral – oral epithelial interactions
Viral infections are common in the oral cavity and often manifest as multiple blisters or ulcerations. Viral infections are associated with tumor formation and lesions, and therefore it is of great interest to find ways to prevent progression of these viral infections before they cause more severe pathologies.
A family of viruses that manifest in the oral cavity are Herpes viruses, of which several types are found in humans. Herpes simplex virus type 1 (HSV-1) and Herpes simplex virus type 2 (HSV-2) cause common opportunistic viral infections. Other types of herpesviruses are Varicella zoster, Cytomegalovirus, and Epstein-Barr Virus. HSV-1 reactivates and replicates in the oral epithelium, leading to localized painful vesicles, colloquially often referred to as “cold sores”. These viruses cause lifelong infections and establish latency with periodic reactivations. HSV-1 viruses often spread by close contact and through sharing saliva and are highly infectious, while most people infected are asymptomatic. To infiltrate the cell, HSV-1 viral particles utilize nectin-1 as their cell surface receptor (). In this case, disruption of AJs can expose nectin-1, allowing HSV to bind to it and further infiltrate the tissue.Citation93 Therefore, the integrity of the AJs in the oral epithelium can determine the course of HSV infection, opening up an important area of future study.Citation94
The Human Immunodeficiency Virus (HIV) has the ability to attack the body’s immune system and then lead to Acquired Immunodeficiency Syndrome (AIDS). Notably, oral lesions can indicate infection with HIV, such as in Kaposi sarcoma.Citation95 It is known that HIV is most often spread primarily by vaginal and rectal routes, but there is also evidence that pediatric HIV infections can occur during breastfeeding.Citation96 Therefore, it is important to consider the role of the oral mucosal barrier in this infection. It has been noted that prolonged interaction of HIV proteins tat and gp120 in oral epithelial cells leads to the disruption of AJs of epithelial cells through reduction of E-cadherin, which can make the cells more susceptible to EMTCitation97 (). In addition, HIV-associated disruption of AJs exposes nectin-1, allowing HSV-1 binding and its opportunistic infection throughout the mucosal epitheliumCitation98 (). Therefore, since adhesion molecules act as viral receptors, it would be of paramount interest to further understand the role of the AJs in viral – epithelial interactions in the oral cavity, to further elucidate the mechanisms by which these diseases can spread throughout the epithelium.
Cleft lip and palate and blepharocheilodontic syndrome
Non-syndromic cleft lip with or without cleft palate (NSCL/P) is one of the most common human birth defects. It is caused by the incorrect fusion of the lip or roof of the mouth in early development. Interestingly, three of the 10 alleles that were identified to have implications in NSCL/P are associated with proteins associated with E-cadherin and nectin complexes. More specifically, these three alleles are mutated variants of PLEKHA7, PLEKHA5, and of CTNND1, the gene that encodes p120 (). It is thought that this dysregulation of the epithelial adhesion complex is a mechanism that is at least partly responsible for NSCL/P.Citation99,Citation100 Another recent genome association study also found an association between NSCL/P and several AJ-related genes, including CDH1, CTNND1, CTNNA1, ESRP1, ESRP2, PLEKHA5, and PLEKHA7.Citation101 Corroborating these findings, experimental disruption of the nectin-afadin complex indeed results in cleft palate.Citation100 Furthermore, missense mutations in E-cadherin are also believed to contribute to cleft lip and palate birth defects in some cases.Citation102 Finally, aberrant Wnt signaling is also associated with non-NSCL/P.Citation103,Citation104
In addition to Non-Syndromic Cleft Lip and Palate, genes that cause Syndromic Cleft Lip and Palate SCL/P can be caused by syndromes, such as Van der Woude syndrome, Ectodermal dysplasia, and Blepharocheilodontic syndrome. Blepharocheilodontic syndrome presents at birth with not solely cleft lip and palate, but also with dental and eyelid anomalies. Mutations of the CDH1 gene, which encodes E-cadherin, and in the CTNND1 gene, which encodes p120, have also been linked to Blepharocheilodontic syndrome.Citation105 These mutations disrupt formation of the AJs and cause overall impairment of cell–cell adhesion, resulting in numerous clinical manifestations, such as cancer progression, as well as congenital abnormalities such as neural tube defects or orofacial clefting.Citation106 The above demonstrate the critical roles of AJ components in oral diseases and offer opportunities for further investigation and for the development of new genetic tests for congenital diseases.
Dental enamel development
Ameloblasts are the precursor cells that form enamel, the rigid, outermost surface of the tooth (Box 1). These cells progress through stages that require intercellular contact and communication. AJs are required in order for these cells to fully differentiate. It was demonstrated that the Rho-associated coiled-coil-containing protein kinase (ROCK) regulates ameloblast differentiation as well as enamel production. Inhibition of ROCK hindered polarization of differentiated ameloblasts, as well as formation of the terminal band structure of actin and E-cadherin.Citation107 Furthermore, it was found that mice without nectin-1 exhibited defective enamel formation.Citation108 The integral membrane proteins of ameloblast AJs are E-cadherin and N-cadherin. Also found in these junctions are associated proteins, which include α-catenin, β-catenin, p120, vinculin, actin, and myosin.Citation109,Citation110 One integral protein to this process is MMP20. MMP20 cleaves the extracellular domains of E- and N-cadherin, facilitating ameloblast organization.Citation111 During the secretory stage of enamel formation, AJs are necessary for the attachment-detachment process that allows the pattern of the enamel to form. Indeed, p120 knockout in a mouse model adversely affects dental enamel development, since p120 plays a role directing the attachment and detachment of the secretory stage ameloblasts as they move in rows.Citation112 Mutations in the AJ proteins nectin-1 and nectin-3 also result in enamel defects, such as a hinderance of mineralization and abnormal tooth shape, further denoting the critical involvement of AJ components in enamel epithelia formation and maintenance.Citation113
Oral cancer
Oral cancer accounts for 2.8% of all cancers in the US, with roughly 54,000 new cases every year (source, NCI: https://seer.cancer.gov/statfacts/html/oralcav.html). However, the 5-year survival is relatively poor (67%) and has not improved significantly in recent years, as opposed to other forms of cancer. Multiple risk factors for oral cancer include aging, tobacco, and alcohol use.Citation114 Oral cancers are more prevalent in males. The most prevalent type of cancer in the oral cavity is oral squamous cell carcinoma (OSCC), representing 87% of cases. Other types include oral leukoplakia, as well as oral melanoma, which accounts for only 0.5% of all oral malignancies.Citation115
Oral squamous cell carcinoma (OSCC)
As with other types of cancer, EMT is involved in OSCC pathogenesis, due to downregulation of E-cadherin and upregulation of mesenchymal cadherins, such as N-cadherin, leading to loss of strong cell–cell adhesion and to increased cell motility.Citation116,Citation117 Interestingly, co-downregulation of E-cadherin and β-catenin seems to associate with negative prognosis in OSCC.Citation118 In an evaluation of 93 cases of OSCC, there was loss of CD44, E-cadherin, and β-catenin, which correlated significantly with poor prognoses.Citation119 E-cadherin mRNA expression has also found to be decreased in OSCC tumors. Downregulation at the mRNA level is likely to be an early and constant event in the evolution of OSCC,Citation98 providing a potential prognostic tool for the progression of the disease. However, further studies are needed to establish whether E-cadherin mRNA levels can indeed constitute a solid predictor of lymph node metastasis in OSCC. Another AJ component, namely alpha actinin 4 (ACTN4), which is an actin-binding protein, has been associated with cancer metastasisCitation100 and is of potential interestdue to its overexpression in many cancer types, including OSCC. Indeed, experimental downregulation of ACTN4 resulted in decreased invasion potential of OSCC cell lines. However, no correlation was found between survival rate and overexpression of ACTN4 in patients,Citation120 leaving the role of ACTN4 in OSCC an open area of investigation. Interestingly, an EMT-promoting role in OSCC has been recently attributed to ion channels, such as the mitochondrial calcium uniporter (MCU) or to chlorine (Cl−) channels, such as CFTR or CLIC4, which could introduce another novel area of mechanistic investigation regarding dysregulation of AJs as a means to promote OSCC.Citation121,Citation122
There are several rare variants of OSCC that altogether account for 10–15% of all OSCC cases, such as verrucous carcinoma (VC), adenoid/acantholytic/pseudoglandular squamous cell carcinoma (AdSCC), adenosquamous carcinoma (ASC), basaloid squamous cell carcinoma (BSCC), papillary squamous cell carcinoma (PSCC), and spindle cell carcinoma (SpCC).Citation123 Sporadic evidence from a small number of case reports have identified loss of E-cadherin expression and increased cytoplasmic β-catenin in the non-metastatic VC and in moderately differentiated AdSCC, suggesting AJ dysregulation and EMT even in early stages of the disease.Citation124,Citation125 Similarly, there was an observed decrease in E-cadherin expression in PSCC of low malignant potential in a rat model.Citation126 Interestingly, no prognostic value of E-cadherin or β-catenin was identified in the more aggressive, head-and-neck specific, BSCC.Citation127 However, of all these rare OSCC subtypes, SpCCis particularly characterized by a dysfunctional cadherin-catenin complex, which results in compromised intercellular adhesion and causes tumor cells to shift from a squamous to a spindled type, with increased infiltrative behavior.Citation128,Citation129 SpCC is indeed characterized by dysplasia of the surface epithelium along with an invasive spindle cell element, expressing both epithelial and mesenchymal markers.Citation130 In the upper aerodigestive tract, it is associated with tobacco and alcohol use. Electron microscopy and immunohistochemical analyses support the concept that these cells are of epithelial origin, with the ability to produce mesenchymal intermediate filaments. In patient samples, catenin expression was found altered in two-thirds of SpCCs, with the most frequent alteration being the loss of γ-catenin, a desmosomal junction catenin, although loss of α-catenin and β-catenin were also observed.Citation129 Indeed, this biphasic tumor demonstrates cadherin switching toward a high expression of N-cadherin, which contributed to SpCC metastasis.Citation117,Citation129,Citation130 These findings demonstrate that dysregulation of junctional components and at least partial EMT may be key for development and progression of this type of oral carcinoma.
Taken together, the above demonstrate that there is extensive dysregulation of the AJ complex in several types of OSCC, although this is not always a clear predictor of aggressive tumorigenesis. This also reveals a lack of mechanistic insight into the role of AJ components in OSCC progression, since the vast majority of these studies are mainly observational. Establishing a causal relationship between AJ component dysregulation and OSCC progression would be critical to understand the role of AJs in OSCC and their potential to be a therapeutic target point for regulation of OSCC cancer cell growth.
Oral melanoma
The most common sites for mucosal melanomas are the head and neck region, while most oral malignant melanomas develop in the maxillary anterior gingiva. However, because of the relative rarity of oral melanoma, it is difficult to diagnose and define presentation and risk factors.Citation131 Despite this, research in this field is important as malignant melanoma is a potentially aggressive tumor, with dismal prognosis and poor survival in patients with advanced disease.Citation132 Currently, the mode of treatment is that pigmented lesions should be clinically viewed with suspicion, as biopsy is necessary to determine the cause of the lesion.Citation132 Although there has been moderate research conducted on the implication of AJs in OSCCs, there is very little research that has been conducted investigating the same in oral melanoma. However, some clues were obtained from a study conducted in canine oral melanocytic tumors. In this study, it was found that dogs with oral melanomas exhibited higher frequency of unfavorable clinical outcomes, when E-cadherin expression was absent.Citation133 Still, the role of E-cadherin in human oral melanoma patient samples has been largely understudied, leaving opportunities to further explore in this rare but highly lethal form of oral carcinoma.
Epithelial dysplasia: leukoplakia
The most common premalignant lesion of the oral mucosa is leukoplakia, which can indicate malignant transformation of the epithelium, although not all dysplastic lesions will become malignant.Citation134 A study conducted to assess the relationship between E-cadherin expression and epithelial dysplasia in oral leukoplakia patients revealed that there are differences in E-cadherin expression between normal oral mucosa and low risk oral leukoplakia, as well as differences between low- and high-risk oral leukoplakia. In normal mucosa, E-cadherin was observed strongly in the basal and parabasal layers.. However, there was a reduction in E- cadherin expression in the parabasal layer at increased stages of dysplasia. In the high-risk dysplasia group, there was a marked E-cadherin reduction in all layers of the epithelium. Therefore, as the epithelia undergo dysplasia, the risk of malignant transformation increases, and there is loss of E-cadherin in keratinocytes.Citation135 In addition, there has been decreased β-catenin expression in basal and parabasal layers in higher degrees of oral leukoplakia, when comparing differences in the amount of mucosal dysplasia, which can alter progression of the disease.Citation136 Therefore, dysplasia may occur due to dysregulation of the E-cadherin-catenin complex, potentially through this dysregulation of β-catenin expression.
Conclusion – perspectives
The oral epithelium is a stratified human tissue responsible for protection from direct exposure to the environment and to numerous pathogens. This renders cellular components that regulate integrity of the epithelium, such as the AJs, critical for the health and homeostasis of the tissue. Although there has been significant research in this field, including the studies we discuss in this review, the role of AJs in diseases of the oral epithelium is far from being fully explored, especially when compared to similar research in other epithelial tissues, which have been more extensively studied. For example, the full breadth of the ways that the hundreds of oral microbial species modulate AJ composition, as well as the mechanisms through which they can tamper with AJs to infiltrate host cells, is still not known. More importantly, little is known on the impact that the microbial-AJ crosstalk in intracellular cadherin signaling and eventually in cell behavior. Such signaling could include, for example, investigation of JNK and RhoA activity, which have been specifically associated with intercellular E-cadherin junctions in human gingival epithelial cells.Citation137 Therefore, further investigation of the microbial-AJ interaction could provide novel insights in the regulation of the diseases of the oral epithelium and can be a future field of therapeutic intervention of pathologies that are caused by external pathogens, both bacterial and viral.
Furthermore, recent advances have revealed several new AJ components that are potentially involved in oral pathologies, such as PLEKHA7 and PLEKHA5 in NSCL/P.Citation99 These proteins have been studied in other tissues and cells and have been shown to have important roles in AJ function and integrity.Citation30,Citation62,Citation63,Citation65,Citation66,Citation68,Citation69,Citation138 For example, PLEKHA7 has been shown to recruit and regulate the RNAi machinery at AJs.Citation61,Citation62 However, no mechanistic studies have been conducted on these AJ components with regard to the oral epithelium. Since dysregulation of AJs could impact miRNA function, and PLEKHA7 mutations have been correlated with NSCL/P, it would be interesting to examine whether this correlation is mechanistically explained through the miRNA-related or other reported functions of PLEKHA7. Ultimately, studies like these can pave the way for the development of novel therapeutics. For example, if AJ disruption leads to miRNA dysregulation, there is the potential use of RNAi as replacement therapy.Citation139,Citation140 Indeed, RNAi therapies in the context of cancers, such as oral cancer, could be an important new direction, as the limited current therapies have side effects. In addition, due to the important roles of β-catenin as a structural component of AJs and as a regulator in cancer progression during homeostasis, Wnt/β-catenin signaling could serve as another therapeutic target for HNSCC.Citation141 Finally, recent work has revealed roles of ion channels, such as TRPV4, as being critical for the formation and stability of AJs in the junctional epithelium, and related their loss to periodontal disease, which could be another intriguing avenue of investigation.Citation142,Citation143
In summary, it is likely that additional mechanistic work at the cellular and the molecular level of the oral epithelial AJs, will elucidate the ways that the AJ is involved in the progression of oral diseases, leading to the design of better prognostic, diagnostic, and therapeutic strategies. The study of AJs has the potential to be a novel and critical area of future study in the oral health field.
Acknowledgments
This work was supported by NIH grants R01 DK124553, R21 CA246233, P20 GM103499-21S1, and P30 DK123704 to AK. CK was supported by NIH training grant 5T32DE017551. were created using BioRender.com.
Disclosure statement
No potential conflict of interest was reported by the author(s).
Additional information
Funding
References
- Kamrani P, Sadiq NM. Anatomy, head and neck, oral cavity (mouth). Treasure Island (FL): StatPearls; 2021.
- Groeger S, Meyle J. Oral mucosal epithelial cells. Front Immunol. 2019;10:147. doi:10.3389/fimmu.2019.00208.
- Brizuela M, Winters R. Histology, oral mucosa. Treasure Island (FL): StatPearls; 2021.
- du Toit Df, du Toit DF. The tongue: structure and function relevant to disease and oral health. Sadj. 2003;58(9):375. 80-3.
- AlJulaih GH, Lasrado SA. Head and neck, tongue taste buds. Treasure Island (FL): StatPearls; 2021.
- Hatakeyama S, Yaegashi T, Oikawa Y, Fujiwara H, Mikami T, Takeda Y, Satoh M. Expression pattern of adhesion molecules in junctional epithelium differs from that in other gingival epithelia. J Periodontal Res. 2006;41(4):322–163. doi:10.1111/j.1600-0765.2006.00875.x.
- Schroeder HE, Listgarten MA. The gingival tissues: the architecture of periodontal protection. Periodontol. 1997 2000;13(1):91–120. doi:10.1111/j.1600-0757.1997.tb00097.x.
- Shimono M, Ishikawa T, Enokiya Y, Muramatsu T, Matsuzaka K, Inoue T, Abiko Y, Yamaza T, Kido M, Tanaka T, Hashimoto S. Biological characteristics of the junctional epithelium. J Electron Microsc (Tokyo). 2003;52(6):627–639. doi:10.1093/jmicro/52.6.627.
- Hintermann E, Haake SK, Christen U, Sharabi A, Quaranta V. Discrete proteolysis of focal contact and adherens junction components in porphyromonas gingivalis- infected oral keratinocytes: a strategy for cell adhesion and migration disabling. Infect Immun. 2002;70(10):5846–5856. doi:10.1128/IAI.70.10.5846-5856.2002.
- Yajima-Himuro S, Oshima M, Yamamoto G, Ogawa M, Furuya M, Tanaka J, Nishii K, Mishima K, Tachikawa T, Tsuji T, et al. The junctional epithelium originates from the odontogenic epithelium of an erupted tooth. Sci Rep. 2014;4(1):4867. doi:10.1038/srep04867.
- Gao L, Xu T, Huang G, Jiang S, Gu Y, Chen F. Oral microbiomes: more and more importance in oral cavity and whole body. Protein Cell. 2018;9(5):488–500. doi:10.1007/s13238-018-0548-1.
- Turner JR. Intestinal mucosal barrier function in health and disease. Nat Rev Immunol. 2009;9(11):799–809. doi:10.1038/nri2653.
- Brandner JM, Kief S, Grund C, Rendl M, Houdek P, Kuhn C, Tschachler E, Franke WW, Moll I. Organization and formation of the tight junction system in human epidermis and cultured keratinocytes. Eur J Cell Biol. 2002;81(5):253–263. doi:10.1078/0171-9335-00244.
- Damek-Poprawa M, Korostoff J, Gill R, DiRienzo JM. Cell junction remodeling in gingival tissue exposed to a microbial toxin. J Dent Res. 2013;92(6):518–523. doi:10.1177/0022034513486807.
- Blake CA, Boockfor FR, Nair-Menon JU, Millette CF, Raychoudhury SS, McCoy GL. Effects of 4-tert-octylphenol given in drinking water for 4 months on the male reproductive system of fischer 344 rats. Reprod Toxicol. 2004;18(1):43–51. doi:10.1016/j.reprotox.2003.09.003.
- Gröger S, Michel J, Meyle J. Establishment and characterization of immortalized human gingival keratinocyte cell lines. J Periodontal Res. 2008;43(6):604–614. doi:10.1111/j.1600-0765.2007.01019.x.
- Langbein L, Grund C, Kuhn C, Praetzel S, Kartenbeck J, Brandner JM, Moll I, Franke WW. Tight junctions and compositionally related junctional structures in mammalian stratified epithelia and cell cultures derived therefrom. Eur J Cell Biol. 2002;81(8):419–435. doi:10.1078/0171-9335-00270.
- Samiei M, Ahmadian E, Eftekhari A, Eghbal MA, Rezaie F, Vinken M. Cell junctions and oral health. Excli J. 2019;18:317–330. doi:10.17179/excli2019-1370.
- Tugizov S. Virus-associated disruption of mucosal epithelial tight junctions and its role in viral transmission and spread. Tissue Barriers. 2021;9(4):1943274. doi:10.1080/21688370.2021.1943274.
- Kaiser HW, Ness W, Jungblut I, Briggaman RA, Kreysel HW, O’Keefe EJ. Adherens junctions: demonstration in human epidermis. J Invest Dermatol. 1993;100(2):180–185. doi:10.1111/1523-1747.ep12462801.
- Wan H, Gadmor H, Brown L. Anchoring junctions in the oral mucosa: adherens junctions and desmosomes. In: Bergmeier LA, editor. Oral mucosa in health and disease: a concise handbook. Cham, Switzerland: Springer International Publishing; 2018. p. 31–51.
- Garcia MA, Nelson WJ, Chavez N. Cell-cell junctions organize structural and signaling networks. Cold Spring Harb Perspect Biol. 2018;10(4):a029181. doi:10.1101/cshperspect.a029181.
- Fu R, Jiang X, Li G, Zhu Y, Zhang H. Junctional complexes in epithelial cells: sentinels for extracellular insults and intracellular homeostasis. FEBS J. 2021. doi:10.1111/febs.16174.
- Rubsam M, Mertz AF, Kubo A, Marg S, Jungst C, Goranci-Buzhala G, Schauss AC, Horsley V, Dufresne ER, Moser M, et al. E-cadherin integrates mechanotransduction and EGFR signaling to control junctional tissue polarization and tight junction positioning. Nat Commun. 2017;8(1):1250. doi:10.1038/s41467-017-01170-7.
- Tunggal JA, Helfrich I, Schmitz A, Schwarz H, Gunzel D, Fromm M, Kemler R, Krieg T, Niessen CM. E-cadherin is essential for in vivo epidermal barrier function by regulating tight junctions. EMBO J. 2005;24(6):1146–1156. doi:10.1038/sj.emboj.7600605.
- Niessen CM. Tight junctions/adherens junctions: basic structure and function. J Invest Dermatol. 2007;127(11):2525–2532. doi:10.1038/sj.jid.5700865.
- Ohnemus U, Kohrmeyer K, Houdek P, Rohde H, Wladykowski E, Vidal S, Horstkotte MA, Aepfelbacher M, Kirschner N, Behne MJ, et al. Regulation of epidermal tight-junctions (TJ) during infection with exfoliative toxin-negative staphylococcus strains. J Invest Dermatol. 2008;128(4):906–916. doi:10.1038/sj.jid.5701070.
- Peskoller M, Bhosale A, Gobel K, Lohr J, Miceli S, Perot S, Persa O, Rübsam M, Shah J, Zhang H, et al. How to build and regenerate a functional skin barrier: the adhesive and cell shaping travels of a keratinocyte. J Invest Dermatol. 2022;142(4):1020–1025. doi:10.1016/j.jid.2021.12.034.
- Hirai Y, Nose A, Kobayashi S, Takeichi M. Expression and role of E- and P-cadherin adhesion molecules in embryonic histogenesis. II. skin morphogenesis. Development. 1989;105(2):271–277. doi:10.1242/dev.105.2.271.
- Kourtidis A, Lu R, Pence LJ, Anastasiadis PZ. A central role for cadherin signaling in cancer. Exp Cell Res. 2017;358(1):78–85. doi:10.1016/j.yexcr.2017.04.006.
- Takeichi M. Dynamic contacts: rearranging adherens junctions to drive epithelial remodelling. Nat Rev Mol Cell Biol. 2014;15(6):397–410. doi:10.1038/nrm3802.
- Mandai K, Rikitake Y, Mori M, Takai Y. Nectins and nectin-like molecules in development and disease. Curr Top Dev Biol. 2015;112:197–231.
- Leerberg JM, Gomez GA, Verma S, Moussa EJ, Wu SK, Priya R, Hoffman B, Grashoff C, Schwartz M, Yap A, et al. Tension-sensitive actin assembly supports contractility at the epithelial zonula adherens. Curr Biol. 2014;24(15):1689–1699. doi:10.1016/j.cub.2014.06.028.
- Ratheesh A, Yap AS. A bigger picture: classical cadherins and the dynamic actin cytoskeleton. Nat Rev Mol Cell Biol. 2012;13(10):673–679. doi:10.1038/nrm3431.
- Smutny M, Cox HL, Leerberg JM, Kovacs EM, Conti MA, Ferguson C, Hamilton NA, Parton RG, Adelstein RS, Yap AS, et al. Myosin II isoforms identify distinct functional modules that support integrity of the epithelial zonula adherens. Nat Cell Biol. 2010;12(7):696–702. doi:10.1038/ncb2072.
- Pinheiro D, Bellaiche Y. Mechanical force-driven adherens junction remodeling and epithelial dynamics. Dev Cell. 2018;47(1):3–19. doi:10.1016/j.devcel.2018.09.014.
- Yang YA, Nguyen E, Sankara Narayana GHN, Heuze M, Fu C, Yu H, Mège R-M, Ladoux B, Sheetz MP. Local contractions regulate E-cadherin rigidity sensing. Sci Adv. 2022;8(4):eabk0387. doi:10.1126/sciadv.abk0387.
- Wickstrom SA, Niessen CM. Cell adhesion and mechanics as drivers of tissue organization and differentiation: local cues for large scale organization. Curr Opin Cell Biol. 2018;54:89–97. doi:10.1016/j.ceb.2018.05.003.
- Vaezi A, Bauer C, Vasioukhin V, Fuchs E. Actin cable dynamics and Rho/Rock orchestrate a polarized cytoskeletal architecture in the early steps of assembling a stratified epithelium. Dev Cell. 2002;3(3):367–381. doi:10.1016/S1534-5807(02)00259-9.
- Peglion F, Llense F, Etienne-Manneville S. Adherens junction treadmilling during collective migration. Nat Cell Biol. 2014;16(7):639–651. doi:10.1038/ncb2985.
- Scarpa E, Szabo A, Bibonne A, Theveneau E, Parsons M, Mayor R. Cadherin switch during EMT in neural crest cells leads to contact inhibition of locomotion via repolarization of forces. Dev Cell. 2015;34(4):421–434. doi:10.1016/j.devcel.2015.06.012.
- Yanagisawa M, Anastasiadis PZ. p120 catenin is essential for mesenchymal cadherin-mediated regulation of cell motility and invasiveness. J Cell Biol. 2006;174:1087–1096. doi:10.1083/jcb.200605022.
- Rodriguez FJ, Lewis-Tuffin LJ, Anastasiadis PZ. E-cadherin’s dark side: possible role in tumor progression. Biochim Biophys Acta. 2012;1826(1):23–31. doi:10.1016/j.bbcan.2012.03.002.
- Zhu AJ, Watt FM. beta-catenin signalling modulates proliferative potential of human epidermal keratinocytes independently of intercellular adhesion. Development. 1999;126(10):2285–2298. doi:10.1242/dev.126.10.2285.
- Daulagala AC, Bridges MC, Kourtidis A. E-cadherin beyond structure: a signaling hub in colon homeostasis and disease. Int J Mol Sci. 2019;20(11):2756. doi:10.3390/ijms20112756.
- Schlegelmilch K, Mohseni M, Kirak O, Pruszak J, Rodriguez JR, Zhou D, Kreger B, Vasioukhin V, Avruch J, Brummelkamp T, et al. Yap1 acts downstream of alpha-catenin to control epidermal proliferation. Cell. 2011;144(5):782–795. doi:10.1016/j.cell.2011.02.031.
- Lien WH, Klezovitch O, Fernandez TE, Delrow J, Vasioukhin V. alphaE-catenin controls cerebral cortical size by regulating the hedgehog signaling pathway. Science. 2006;311(5767):1609–1612. doi:10.1126/science.1121449.
- Silvis MR, Kreger BT, Lien WH, Klezovitch O, Rudakova GM, Camargo FD, Lantz DM, Seykora JT, Vasioukhin V. alpha-catenin is a tumor suppressor that controls cell accumulation by regulating the localization and activity of the transcriptional coactivator Yap1. Sci Signal. 2011;4(174):ra33. doi:10.1126/scisignal.2001823.
- Kourtidis A, Ngok SP, Anastasiadis PZ. Chapter eighteen - p120 catenin: an essential regulator of cadherin stability, adhesion-induced signaling, and cancer progression. In: van Roy F editor. Progress in molecular biology and translational science. Cambridge: Academic Press; 2013. p. 409–432.
- Mariner DJ, Davis MA, Reynolds AB. EGFR signaling to p120-catenin through phosphorylation at Y228. J Cell Sci. 2004;117(8):1339–1350. doi:10.1242/jcs.01001.
- Ozawa M, Ohkubo T. Tyrosine phosphorylation of p120(ctn) in v-Src transfected L cells depends on its association with E-cadherin and reduces adhesion activity. J Cell Sci. 2001;114(3):503–512. doi:10.1242/jcs.114.3.503.
- Sullivan B, Light T, Vu V, Kapustka A, Hristova K, Leckband D. Mechanical disruption of E-cadherin complexes with epidermal growth factor receptor actuates growth factor-dependent signaling. Proc Natl Acad Sci U S A. 2022;119(4). doi:10.1075/pnas.2100679119. PubMed ID: 35074920.
- Daniel JM, Reynolds AB. The catenin p120 ctn interacts with kaiso, a novel BTB/POZ domain zinc finger transcription factor. Mol Cell Biol. 1999;19(5):3614–3623. doi:10.1128/MCB.19.5.3614.
- Daniel JM. Dancing in and out of the nucleus: p120(ctn) and the transcription factor Kaiso. Biochim Biophys Acta. 2007;1773(1):59–68. doi:10.1016/j.bbamcr.2006.08.052.
- Kourtidis A, Ngok SP. Anastasiadis PZ. p120 catenin: an essential regulator of cadherin stability, adhesion-induced signaling, and cancer progression. Prog Mol Biol Transl Sci. 2013;116:409–432.
- van Roy Fm, McCrea PD, van Roy FM. A role for kaiso-p120ctn complexes in cancer? Nat Rev Cancer. 2005;5(12):956–964. doi:10.1038/nrc1752.
- Dohn MR, Brown MV, Reynolds AB. An essential role for p120-catenin in Src- and Rac1-mediated anchorage-independent cell growth. J Cell Biol. 2009;184(3):437–450. doi:10.1083/jcb.200807096.
- Anastasiadis PZ, Moon SY, Thoreson MA, Mariner DJ, Crawford HC, Zheng Y, Reynolds AB. Inhibition of RhoA by p120 catenin. Nat Cell Biol. 2000;2(9):637–644. doi:10.1038/35023588.
- Zebda N, Tian Y, Tian X, Gawlak G, Higginbotham K, Reynolds AB, Birukova AA, Birukov KG. Interaction of p190RhoGAP with C-terminal domain of p120-catenin modulates endothelial cytoskeleton and permeability. J Biol Chem. 2013;288(25):18290–18299. doi:10.1074/jbc.M112.432757.
- Paschoud S, Jond L, Guerrera D, Citi S. PLEKHA7 modulates epithelial tight junction barrier function. Tissue Barriers. 2014;2(2):e28755. doi:10.4161/tisb.28755.
- Kourtidis A, Ngok SP, Pulimeno P, Feathers RW, Carpio LR, Baker TR, Carr J, Yan I, Borges S, Perez E, et al. Distinct E-cadherin-based complexes regulate cell behaviour through miRNA processing or Src and p120 catenin activity. Nat Cell Biol. 2015;17(9):1145–1157. doi:10.1038/ncb3227.
- Kourtidis A, Necela B, Lin WH, Lu R, Feathers RW, Asmann YW, Thompson EA, Anastasiadis PZ. Cadherin complexes recruit mRNAs and RISC to regulate epithelial cell signaling. J Cell Biol. 2017;216(10):3073–3085. doi:10.1083/jcb.201612125.
- Nair-Menon J, Daulagala AC, Connor DM, Rutledge L, Penix T, Bridges MC, et al. Predominant distribution of THE RNAi machinery at apical adherens junctions in colonic epithelia is disrupted in cancer. Int J Mol Sci. 2020;21(7): 2559.
- Pence LJ, Kourtidis A, Feathers RW, Haddad MT, Sotiriou S, Decker PA, Nassar A, Ocal IT, Anastasiadis P. PLEKHA7, an apical adherens junction protein, suppresses inflammatory breast cancer in the context of high e-cadherin and p120-catenin expression. Int J Mol Sci. 2021;23(1):22. doi:10.3390/ijms23010022.
- Meng W, Mushika Y, Ichii T, Takeichi M. Anchorage of microtubule minus ends to adherens junctions regulates epithelial cell-cell contacts. Cell. 2008;135(5):948–959. doi:10.1016/j.cell.2008.09.040.
- Pulimeno P, Bauer C, Stutz J, Citi S, Arkowitz RA. PLEKHA7 is an adherens junction protein with a tissue distribution and subcellular localization distinct from ZO-1 and E-cadherin. PLoS One. 2010;5(8):e12207. doi:10.1371/journal.pone.0012207.
- Pulimeno P, Paschoud S, Citi S. A role for ZO-1 and PLEKHA7 in recruiting paracingulin to tight and adherens junctions of epithelial cells. J Biol Chem. 2011;286(19):16743–16750. doi:10.1074/jbc.M111.230862.
- Kourtidis A, Anastasiadis PZ. PLEKHA7 defines an apical junctional complex with cytoskeletal associations and miRNA-mediated growth implications. Cell Cycle. 2016;15(4):498–505. doi:10.1080/15384101.2016.1141840.
- Shah J, Guerrera D, Vasileva E, Sluysmans S, Bertels E, Citi S. PLEKHA7: cytoskeletal adaptor protein at center stage in junctional organization and signaling. Int J Biochem Cell Biol. 2016;75:112–116. doi:10.1016/j.biocel.2016.04.001.
- Aas JA, Paster BJ, Stokes LN, Olsen I, Dewhirst FE. Defining the normal bacterial flora of the oral cavity. J Clin Microbiol. 2005;43(11):5721–5732. doi:10.1128/JCM.43.11.5721-5732.2005.
- Xu X, He J, Xue J, Wang Y, Li K, Zhang K, Guo Q, Liu X, Zhou Y, Cheng L, et al. Oral cavity contains distinct niches with dynamic microbial communities. Environ Microbiol. 2015;17(3):699–710. doi:10.1111/1462-2920.12502.
- Takahashi N, Sulijaya B, Yamada-Hara M, Tsuzuno T, Tabeta K, Yamazaki K. Gingival epithelial barrier: regulation by beneficial and harmful microbes. Tissue Barriers. 2019;7(3):e1651158. doi:10.1080/21688370.2019.1651158.
- Ye P, Chapple CC, Kumar RK, Hunter N. Expression patterns of E-cadherin, involucrin, and connexin gap junction proteins in the lining epithelia of inflamed gingiva. J Pathol. 2000;192(1):58–66. doi:10.1002/1096-9896(2000)9999:9999<::AID-PATH673>3.0.CO;2-T.
- Arun R, Hemalatha R, Arun KV, Kumar T. E-cadherin and CD1a expression in gingival epithelium in periodontal health, disease and post-treatment. Indian J Dent Res. 2010;21(3):396–401. doi:10.4103/0970-9290.70811.
- Abe-Yutori M, Chikazawa T, Shibasaki K, Murakami S. Decreased expression of E-cadherin by Porphyromonas gingivalis -lipopolysaccharide attenuates epithelial barrier function. J Periodontal Res. 2017;52(1):42–50. doi:10.1111/jre.12367.
- Lee J, Roberts JS, Atanasova KR, Chowdhury N, Han K, Ö Y. Human primary epithelial cells acquire an epithelial-mesenchymal-transition phenotype during long-term infection by the oral opportunistic pathogen, porphyromonas gingivalis. Front Cell Infect Microbiol. 2017;7. doi:10.3389/fcimb.2017.00493.
- Katz J, Sambandam V, Wu JH, Michalek SM, Balkovetz DF, Moore RN. Characterization of porphyromonas gingivalis -induced degradation of epithelial cell junctional complexes. Infect Immun. 2000;68(3):1441–1449. doi:10.1128/IAI.68.3.1441-1449.2000.
- Noguchi T, Shiba H, Komatsuzawa H, Mizuno N, Uchida Y, Ouhara K, Asakawa R, Kudo S, Kawaguchi H, Sugai M, et al. Syntheses of Prostaglandin E 2 and E-Cadherin and Gene Expression of -defensin-2 by Human Gingival Epithelial Cells in Response to Actinobacillus actinomycetemcomitans. Inflammation. 2003;27(6):341–349. doi:10.1023/B:IFLA.0000006702.27906.e9.
- Raja M, Ummer F, Dhivakar CP. Aggregatibacter actinomycetemcomitans - a tooth killer? J Clin Diagn Res. 2014;8(8):ZE13–6. doi:10.7860/JCDR/2014/9845.4766.
- Damek-Poprawa M, Haris M, Volgina A, Korostoff J, DiRienzo JM. Cytolethal distending toxin damages the oral epithelium of gingival explants. J Dent Res. 2011;90(7):874–879. doi:10.1177/0022034511403743.
- Fujita T, Kishimoto A, Shiba H, Hayashida K, Kajiya M, Uchida Y, Matsuda S, Takeda K, Ouhara K, Kawaguchi H, et al. Irsogladine maleate regulates neutrophil migration and E-cadherin expression in gingival epithelium stimulated by Aggregatibacter actinomycetemcomitans. Biochem Pharmacol. 2010;79(10):1496–1505. doi:10.1016/j.bcp.2010.01.017.
- Reid G, Burton J. Use of Lactobacillus to prevent infection by pathogenic bacteria. Microbes Infect. 2002;4(3):319–324. doi:10.1016/S1286-4579(02)01544-7.
- Dal Bello F, Hertel C. Oral cavity as natural reservoir for intestinal lactobacilli. Syst Appl Microbiol. 2006;29(1):69–76. doi:10.1016/j.syapm.2005.07.002.
- Hummel S, Veltman K, Cichon C, Sonnenborn U, Schmidt MA. differential targeting of the E-Cadherin/β-Catenin complex by gram-positive probiotic lactobacilli improves epithelial barrier function. Appl Environ Microbiol. 2012;78(4):1140–1147. doi:10.1128/AEM.06983-11.
- Ghanavati R, Akbari A, Mohammadi F, Asadollahi P, Javadi A, Talebi M, Rohani M. Lactobacillus species inhibitory effect on colorectal cancer progression through modulating the Wnt/β-catenin signaling pathway. Mol Cell Biochem. 2020;470(1–2):1–13. doi:10.1007/s11010-020-03740-8.
- Han N, Jia L, Su Y, Du J, Guo L, Luo Z, Liu Y. Lactobacillus reuteri extracts promoted wound healing via PI3K/AKT/beta-catenin/TGFbeta1 pathway. Stem Cell Res Ther. 2019;10(1):243. doi:10.1186/s13287-019-1324-8.
- Cross SE, Kreth J, Wali RP, Sullivan R, Shi W, Gimzewski JK. Evaluation of bacteria-induced enamel demineralization using optical profilometry. Dent Mater. 2009;25(12):1517–1526. doi:10.1016/j.dental.2009.07.012.
- Byun R, Nadkarni MA, Chhour K-L, Martin FE, Jacques NA, Hunter N. Quantitative analysis of diverse lactobacillus species present in advanced dental caries. J Clin Microbiol. 2004;42(7):3128–3136. doi:10.1128/JCM.42.7.3128-3136.2004.
- Villar CC, Kashleva H, Nobile CJ, Mitchell AP, Dongari-Bagtzoglou A. mucosal tissue invasion by Candida albicans is associated with e-cadherin degradation, mediated by transcription factor rim101p and protease Sap5p. Infect Immun. 2007;75(5):2126–2135. doi:10.1128/IAI.00054-07.
- Rollenhagen C, Wollert T, Langford GM, Sundstrom P. Stimulation of cell motility and expression of late markers of differentiation in human oral keratinocytes by Candida albicans. Cell Microbiol. 2009;11(6):946–966. doi:10.1111/j.1462-5822.2009.01303.x.
- Xu H, Sobue T, Bertolini M, Thompson A, Dongari-Bagtzoglou A. Streptococcus oralis and Candida albicans Synergistically Activate μ-Calpain to Degrade E-cadherin From Oral Epithelial Junctions. J Infect Dis. 2016;214(6):925–934. doi:10.1093/infdis/jiw201.
- Sun JN, Solis NV, Phan QT, Bajwa JS, Kashleva H, Thompson A, Liu Y, Dongari-Bagtzoglou A, Edgerton M, Filler SG, et al. Host cell invasion and virulence mediated by Candida albicans Ssa1. PLoS Pathog. 2010;6(11):e1001181. doi:10.1371/journal.ppat.1001181.
- Yoon M, Spear PG. Disruption of adherens junctions liberates nectin-1 to serve as receptor for herpes simplex virus and pseudorabies virus entry. J Virol. 2002;76(14):7203–7208. doi:10.1128/JVI.76.14.7203-7208.2002.
- Petermann P, Rahn E, Thier K, Hsu M-J, Rixon FJ, Kopp SJ, Knebel-Mörsdorf D. Role of nectin-1 and herpesvirus entry mediator as Cellular receptors for herpes simplex virus 1 on primary murine dermal fibroblasts. J Virol. 2015;89(18):9407–9416. doi:10.1128/JVI.01415-15.
- Coogan MM, Greenspan J, Challacombe SJ. Oral lesions in infection with human immunodeficiency virus. Bull World Health Organ. 2005;83:700–706. 9 S0042-96862005000900016.
- Heron SE, Elahi S. HIV infection and compromised mucosal immunity: oral manifestations and systemic inflammation. Front Immunol. 2017;8:241. doi:10.3389/fimmu.2017.00241.
- Lien K, Mayer W, Herrera R, Rosbe K, Tugizov SM, Silvestri G. HIV-1 proteins gp120 and tat induce the epithelial-mesenchymal transition in oral and genital mucosal epithelial cells. PLoS One. 2019;14(12):e0226343. doi:10.1371/journal.pone.0226343.
- Sufiawati I, Tugizov SM, Krummenacher C. HIV-associated disruption of tight and adherens junctions of oral epithelial cells facilitates HSV-1 infection and spread. PLoS One. 2014;9(2):e88803. doi:10.1371/journal.pone.0088803.
- Cox LL, Cox TC, Moreno Uribe LM, Zhu Y, Richter CT, Nidey N, Standley JM, Deng M, Blue E, Chong JX, et al. Mutations in the epithelial cadherin-p120-catenin complex cause Mendelian non-syndromic cleft lip with or without cleft palate. Am J Hum Genet. 2018;102(6):1143–1157. doi:10.1016/j.ajhg.2018.04.009.
- Lough KJ, Spitzer DC, Bergman AJ, Wu JJ, Byrd KM, Williams SE. Disruption of the nectin-afadin complex recapitulates features of the human cleft lip/palate syndrome CLPED1. Development. 2020;147(21).
- Li M, Wang H. Pathway analysis identified a significant association between cell-cell adherens junctions-related genes and non-syndromic cleft lip/palate in 895 Asian case-parent trios. Arch Oral Biol. 2022;136:105384. doi:10.1016/j.archoralbio.2022.105384.
- Vogelaar IP, Figueiredo J, van Rooij IA, Simoes-Correia J, van der Post RS, Melo S, Seruca R, Carels CEL, Ligtenberg MJL, Hoogerbrugge N, et al. Identification of germline mutations in the cancer predisposing gene CDH1 in patients with orofacial clefts. Hum Mol Genet. 2013;22(5):919–926. doi:10.1093/hmg/dds497.
- Chiquet BT, Blanton SH, Burt A, Ma D, Stal S, Mulliken JB, Hecht JT. Variation in WNT genes is associated with non-syndromic cleft lip with or without cleft palate. Hum Mol Genet. 2008;17(14):2212–2218. doi:10.1093/hmg/ddn121.
- He F, Chen Y. Wnt signaling in lip and palate development. Front Oral Biol. 2012;16:81–90.
- Kievit A, Tessadori F, Douben H, Jordens I, Maurice M, Hoogeboom J, Hennekam R, Nampoothiri S, Kayserili H, Castori M, et al. Variants in members of the cadherin-catenin complex, CDH1 and CTNND1, cause blepharocheilodontic syndrome. Eur J Hum Genet. 2018;26(2):210–219. doi:10.1038/s41431-017-0010-5.
- Figueiredo J, Melo S, Carneiro P, Moreira AM, Fernandes MS, Ribeiro AS, Guilford P, Paredes J, Seruca R. Clinical spectrum and pleiotropic nature of CDH1 germline mutations. J Med Genet. 2019;56(4):199–208. doi:10.1136/jmedgenet-2018-105807.
- Otsu K, Kishigami R, Fujiwara N, Ishizeki K, Harada H. Functional role of Rho-kinase in ameloblast differentiation. J Cell Physiol. 2011;226(10):2527–2534. doi:10.1002/jcp.22597.
- Barron MJ, Brookes SJ, Draper CE, Garrod D, Kirkham J, Shore RC, Dixon MJ. The cell adhesion molecule nectin-1 is critical for normal enamel formation in mice. Hum Mol Genet. 2008;17(22):3509–3520. doi:10.1093/hmg/ddn243.
- Nishikawa S. Cytoskeleton, intercellular junctions, planar cell polarity, and cell movement in amelogenesis. J Oral Biosci. 2017;59(4):197–204. doi:10.1016/j.job.2017.07.002.
- Sorkin BC, Wang MY, Dobeck JM, Albergo KL, Skobe Z. The cadherin-catenin complex is expressed alternately with the adenomatous polyposis coli protein during rat incisor amelogenesis. J Histochem Cytochem. 2000;48(3):397–406. doi:10.1177/002215540004800309.
- Guan X, Bartlett JD. MMP20 modulates cadherin expression in ameloblasts as enamel develops. J Dent Res. 2013;92(12):1123–1128. doi:10.1177/0022034513506581.
- Bartlett JD, Dobeck JM, Tye CE, Perez-Moreno M, Stokes N, Reynolds AB, Fuchs E, Skobe Z. Targeted p120-catenin ablation disrupts dental enamel development. PLoS One. 2010;5(9):e12703. doi:10.1371/journal.pone.0012703.
- Yoshida T, Miyoshi J, Takai Y, Thesleff I. Cooperation of nectin-1 and nectin-3 is required for normal ameloblast function and crown shape development in mouse teeth. Dev Dyn. 2010;239(10):2558–2569. doi:10.1002/dvdy.22395.
- Rivera C. Essentials of oral cancer. Int J Clin Exp Pathol. 2015;8:11884–11894.
- Hicks MJ, Flaitz CM. Oral mucosal melanoma: epidemiology and pathobiology. Oral Oncol. 2000;36(2):152–169. doi:10.1016/S1368-8375(99)00085-8.
- Jayanthi P, Varun BR, Selvaraj J. Epithelial-mesenchymal transition in oral squamous cell carcinoma: an insight into molecular mechanisms and clinical implications. J Oral Maxillofac Pathol. 2020;24:189. doi:10.4103/jomfp.JOMFP_334_19.
- Krisanaprakornkit S, Iamaroon A. Epithelial-mesenchymal transition in oral squamous cell carcinoma. ISRN Oncol. 2012;2012:681469. 2012. doi:10.5402/2012/681469.
- da Silva Sd, Morand GB, Alobaid FA, Hier MP, Mlynarek AM, Alaoui-Jamali MA, da Silva SD, Kowalski LP. Epithelial-mesenchymal transition (EMT) markers have prognostic impact in multiple primary oral squamous cell carcinoma. Clin Exp Metastasis. 2015;32(1):55–63. doi:10.1007/s10585-014-9690-1.
- Bankfalvi A, Krassort M, Buchwalow IB, Vegh A, Felszeghy E, Piffko J. Gains and losses of adhesion molecules (CD44, E-cadherin, and beta-catenin) during oral carcinogenesis and tumour progression. J Pathol. 2002;198(3):343–351. doi:10.1002/path.1204.
- Yamada S, Yanamoto S, Yoshida H, Yoshitomi I, Kawasaki G, Mizuno A, Nemoto TK. RNAi-mediated down-regulation of alpha-actinin-4 decreases invasion potential in oral squamous cell carcinoma. Int J Oral Maxillofac Surg. 2010;39(1):61–67. doi:10.1016/j.ijom.2009.10.003.
- Kei K, Yoshie S, Tsuji S, Murono S, Hazama A. Dysfunction of Cl(-) channels promotes epithelial to mesenchymal transition in oral squamous cell carcinoma via activation of Wnt/beta-catenin signaling pathway. Biochem Biophys Res Commun. 2021;555:95–101. doi:10.1016/j.bbrc.2021.03.130.
- Zheng S, Wu R, Deng Y, Zhang Q. Dihydroartemisinin represses oral squamous cell carcinoma progression through downregulating mitochondrial calcium uniporter. Bioengineered. 2022;13(1):227–241. doi:10.1080/21655979.2021.2012951.
- Pathak J, Swain N, Patel S, Poonja L. Histopathological variants of oral squamous cell carcinoma-institutional case reports. J Oral Maxillofac Pathol. 2014;18(1):143–145. doi:10.4103/0973-029X.131945.
- de Moraes Rv, Oliveira DT, Landman G, de Carvalho F, Caballero O, Nonogaki S, de Moraes RV, de Carvalho F, Nishimoto I, Kowalski LP. E-cadherin abnormalities resulting from CPG methylation promoter in metastatic and nonmetastatic oral cancer. Head Neck. 2008;30(1):85–92. doi:10.1002/hed.20666.
- Bunn B, Hunter K, Khurram SA, van Heerden WF. Adenoid dysplasia of the oral mucosa. Oral Surg Oral Med Oral Pathol Oral Radiol. 2014;118(5):586–592. doi:10.1016/j.oooo.2014.08.009.
- Oliveira PA, Colaco A, la Cruz Lf D, Lopes P, Lopes C. E-cadherin expression during urothelial carcinogenesis induced by N-butyl-N-(4-hydroxybutyl) nitrosamine in rats. J Exp Clin Cancer Res. 2006;25:425–432.
- Hanemann JA, Oliveira DT, Nonogaki S, Nishimoto IN, de Carli ML, Landman G, Kowalski LP. Expression of E-cadherin and beta-catenin in basaloid and conventional squamous cell carcinoma of the oral cavity: are potential prognostic markers? BMC Cancer. 2014;14(1):395. doi:10.1186/1471-2407-14-395.
- Neville BW, Allen CM, Damm DD, Chi AC. Oral and maxillofacial pathology. In: 4th Edition. Missouri: Elsevier; 2016.
- Zidar N, Gale N, Kojc N, Volavsek M, Cardesa A, Alos L, Höfler H, Blechschmidt K, Becker K-F. Cadherin-catenin complex and transcription factor Snail-1 in spindle cell carcinoma of the head and neck. Virchows Arch. 2008;453(3):267–274. doi:10.1007/s00428-008-0649-y.
- Nguyen PT, Kudo Y, Yoshida M, Iizuka S, Ogawa I, Takata T. N-cadherin expression is correlated with metastasis of spindle cell carcinoma of head and neck region. J Oral Pathol Med. 2011;40(1):77–82. doi:10.1111/j.1600-0714.2010.00966.x.
- Rapini RP, Golitz LE, Greer RO Jr., Krekorian EA, Poulson T. Primary malignant melanoma of the oral cavity. A review of 177 cases. Cancer. 1985;55(7):1543–1551. doi:10.1002/1097-0142(19850401)55:7<1543::AID-CNCR2820550722>3.0.CO;2-F.
- van der Waal Ri, Snow GB, Karim AB, van der Waal DW, van I. Primary malignant melanoma of the oral cavity: a review of eight cases. Br Dent J. 1994;176(5):185–188. doi:10.1038/sj.bdj.4808406.
- Silvestri S, Porcellato I, Mechelli L, Menchetti L, Iussich S, De Maria R, Sforna M, Bongiovanni L, Brachelente C. E-Cadherin Expression in Canine Melanocytic Tumors: histological, immunohistochemical, and survival analysis. Vet Pathol. 2020;57(5):608–619. doi:10.1177/0300985820934385.
- van der Waal I, Schepman KP, van der Meij Eh, Smeele LE, van der Meij EH. Oral leukoplakia: a clinicopathological review. Oral Oncol. 1997;33(5):291–301. doi:10.1016/S1368-8375(97)00002-X.
- von Zeidler Sv, de Souza Botelho T, Mendonca EF, Batista AC, von Zeidler SV. E-cadherin as a potential biomarker of malignant transformation in oral leukoplakia: a retrospective cohort study. BMC Cancer. 2014;14(1):972. doi:10.1186/1471-2407-14-972.
- Silva BS, Castro CA, Von Zeidler SL, Sousa SC, Batista AC, Yamamoto-Silva FP. Altered beta-catenin expression in oral mucosal dysplasia: a comparative study. J Appl Oral Sci. 2015;23(5):472–478. doi:10.1590/1678-775720150150.
- Lee G, Kim HJ, Kim H-M. RhoA-JNK regulates the E-Cadherin junctions of human gingival epithelial cells. J Dent Res. 2016;95(3):284–291. doi:10.1177/0022034515619375.
- Rea K, Roggiani F, De Cecco L, Raspagliesi F, Carcangiu ML, Nair-Menon J, Bagnoli M, Bortolomai I, Mezzanzanica D, Canevari S, et al. Simultaneous E-cadherin and PLEKHA7 expression negatively affects E-cadherin/EGFR mediated ovarian cancer cell growth. J Exp Clin Cancer Res. 2018;37(1):146. doi:10.1186/s13046-018-0796-1.
- Rao DD, Vorhies JS, Senzer N, Nemunaitis J. siRNA vs. shRNA: similarities and differences. Adv Drug Deliv Rev. 2009;61(9):746–759. doi:10.1016/j.addr.2009.04.004.
- Abdelrahim M, Safe S, Baker C, Abudayyeh A. RNAi and cancer: implications and applications. J RNAi Gene Silencing. 2006;2:136–145.
- Alamoud KA, Kukuruzinska MA. Emerging insights into wnt/beta-catenin signaling in head and neck cancer. J Dent Res. 2018;97(6):665–673. doi:10.1177/0022034518771923.
- Kitsuki T, Yoshimoto RU, Aijima R, Hatakeyama J, Cao AL, Zhang JQ, Ohsaki Y, Mori Y, Kido MA. Enhanced junctional epithelial permeability in TRPV4-deficient mice. J Periodontal Res. 2020;55(1):51–60. doi:10.1111/jre.12685.
- Yoshimoto RU, Aijima R, Ohyama Y, Yoshizumi J, Kitsuki T, Ohsaki Y, Cao A-L, Danjo A, Yamashita Y, Kiyoshima T, et al. Impaired junctions and invaded macrophages in oral epithelia with oral pain. J Histochem Cytochem. 2019;67(4):245–256. doi:10.1369/0022155418812405.