Abstract
Laurel seed lipase was entrapped within chitosan beads with ionotropic gelatin method using tripolyphosphate (TPP) as multivalent covalent counter ion. Immobilization yield was 78%. First, optimum immobilization conditions were determined, and morphology of chitosan beads was characterized by scanning electron microscopy. Optimum pH and temperature were evaluated as 6.0 and 40 °C, respectively. The immobilized beads saved about 55% of its activities at 60° while saved about 32% at 70 °C for 30 min. Vmax/Km values were determined as 31.75 and 2.87 using olive oil as substrate for immobilized beads and free enzyme, respectively. Immobilized beads showed the activities during 30 days at +4 °C.
Introduction
Immobilization is attaching or entrapping of enzymes on/in an inert and insoluble material. There are some advantages of immobilization including increase in enzyme stability to changes like pH or temperature, continuous or repeated use of the enzyme, easy removal from the reaction media, and low cost of the operation (Sheldon Citation2007). Immobilization methods are generally categorized into four groups: adsorption, covalent binding, crosslinking and entrapment. The entrapment method is one of the methods used frequently for enzyme immobilization because it is moderate and, encompasses relatively little damage to the native structure of the enzymes. In this method, enzyme is entrapped within natural biopolymers including chitosan, sodium alginate, gelatin, and κ-carrageenan, which are non-toxic and biocompatible (Kara et al. Citation2006, Pulat et al. Citation2013, Tumturk et al. Citation2008).
Chitosan is an N-acetylglucosamine polymer that has recently been used in immobilization technologies (Klein et al. Citation2012). It has hydroxyl and amino groups which are excellent functional groups for ionotropic gelatin method. In this method, multivalent covalent counter ions like tripolyphosphate (pentasodium triphosphate) are added to polycationic chitosan solution. This application is a simple and widely used for enzyme immobilization (Alsarra et al. Citation2004). Also, gels prepared with the different methods in this manner from natural chitosan polymer have been successfully employed for lipase entrapment (Aimée et al. Citation2008, Kumar et al. Citation2013, Pulat et al. Citation2014, Siodmiak et al. Citation2013, Toscano et al. Citation2014, Zhou et al. Citation2014).
Lipases (triacylglycerol ester hydrolases, EC 3.1.1.3) are ubiquitous enzymes that catalyze the hydrolysis of fats and oils to give free fatty acids, diacylglycerols, monoglycerides, and glycerol in the aqueous medium (Ak et al. Citation2014, Pimentel et al. Citation2007). Besides this, they are also effective in various organic reactions such as esterification and transesterification by using organic solvents. Recently they have gained attention due to these potentials in synthetic reactions (Villeneuve et al. Citation2000). The applications of lipases in industrial processes include food chemistry, oil chemistry, organic synthesis, paper manufacturing, the synthesis of products such as surfactant, cosmetic, pharmaceutical, biodiesel, detergent (Mohamed et al. Citation2000, Rubin and Dennis Citation1997, Sharma et al. Citation2002).
Lipases can be obtained from animals, microorganisms and plants, which showed the different catalytic properties as a function of the source. The plant lipases have great potential as the commercial enzymes, which oilseeds especially considered as underutilized (Enujiugha et al. Citation2004). In this study, bay laurel lipase (Lauris nobilis L.) was entrapped in chitosan gel and their hydrolytic activity were determined using olive oil as substrate. The knowledge in the area of bay laurel lipase is limited and there is no data available about its immobilization. Isbilir et al. (Citation2008) have isolated lipase from laurel seeds and determined their biochemical properties by using laurel oil as substrate. One of the authors of this study has taken place in that study. The aim of this investigation is to immobilize the laurel lipase within chitosan beads with ionotropic gelatin method using tripolyphosphate (TPP) as multivalent covalent counter ion, to optimize the immobilization conditions, and to determine some biochemical properties of immobilized enzyme.
Methods
Enzyme isolation and assay of lipase activity
The laurel seeds were collected from Hatay, TURKEY. 10 g of laurel seeds peeled off were ground in Waring blender and mixed with 70 mL of Tris-HCl buffer (0.2 M, pH 7.4) on a magnetic stirrer at cold for 1 h. The obtained homogenate was filtered from cheesecloth, and lipase enzyme recovered in the supernatant (Isbilir et al. Citation2008).
Hydrolytic activity of laurel lipase was determined in olive oil emulsions as described previously (Hoppe and Theimer Citation1996). Protein concentration was determined by using Lowry method with bovine serum albumin as standard (Lowry et al. Citation1951).
Lipase immobilization
Laurel lipase-loaded hydrogel beads were prepared according to the modified method of Alsarra et al. using tripolyphosphate (TPP) as the gelling counter ion (Alsarra et al. Citation2004). After 200 mg of chitosan was dissolved in 8 ml of 1% (v/v) acetic acid, 2 mL of laurel lipase solution was added to this chitosan solution in order to prepare chitosan dispersion. Bubble-free mixture stood at the 4 °C was extruded drop-wise through a syringe (0.8 mm diameter) in to a gently agitated 0.4% (w/v) TPP solution prepared in 0.05 M Tris-HCl buffer (pH 7.0). The chitosan beads were cured in the TPP solution for 75 min and then washed twice using 0.05 M Tris-HCl buffer (pH 7.0). The filtered TPP solution and two washings were collected for protein determination. Obtained beads were stored at 4 °C until use.
SEM analysis of chitosan beads
The surface morphologies of chitosan beads were examined by a field emission scanning electron microscopy (FEI-Quanta FEG 250 model, at 10,000-fold magnifications) at Scientific and Technological Research Center of Namik Kemal University (NABILTEM), Tekirdag, Turkey. The acceleration voltages of 2 and 5 kV were used to acquire SEM images.
Optimization of preparation conditions of hydrogel beads
Various amounts of chitosan (150 mg, 200 mg and 250 mg) were dissolved in 8 ml of 1% (v/v) acetic acid to study the effect of chitosan concentration on bead permeability. The formation of beads was carried out by 0.4% (w/v) pentasodium triphosphate (TPP) solution prepared in 0.05 M Tris-HCl buffer (pH 7.0).
In order to determine the effect of pH of TPP solution on the hardness of beads, chitosan solution was extruded drop-wise into TPP solutions prepared different pHs (6.5, 7.0 and 7.5) by fixing TPP concentration at 0.5% (w/v). The concentration of TPP in the gelling solution was determined by using TPP solutions prepared at different concentrations from 0.3 to 0.5% (w/v) with the pH fixed at 7.0.
Different enzyme loading in the beads was examined by incorporating various amounts of enzyme (1 mL, 2 mL, and 3 mL) in 200 mg of chitosan solution dissolved in 1% (v/v) acetic acid.
The effect of bead size on the lipase activity was investigated by altering the diameters of the syringe needle used in immobilization assays (0.45, 0.8, and 3 mm diameter).
The effect of chitosan bead amounts on the laurel lipase activity was determined by using various bead amounts (0.25, 0.5, and 1 g beads).
Some biochemical properties of immobilized enzyme
The effect of pH on the activity of immobilized laurel lipase was investigated by using Tris-maleate buffer solutions prepared at different pHs from 5.0 to 9.0.
The influence of temperature on the activity of immobilized lipase was studied by incubating the reaction mixture at different temperatures (30, 40, and 50 °C).
The immobilized laurel lipase samples were incubated at different temperatures between 40 and 80 °C for 30 and 60 min to study the thermal stabilities of immobilized lipase in chitosan beads.
The effect of substrate concentration on the activity of immobilized lipase was studied by using different amounts of olive oil (0.25–0.75 g). Km and Vmax were calculated from the Lineweaver–Burk plots.
In order to test the reuse of entrapped lipase, the hydrolytic activity of laurel lipase in the beads was assayed several times. For this purpose, lipase-loaded hydrogel beads and olive oil emulsions stabilized by emulsifying agents such as gum arabic and deoxicholate were incubated for 15 min, beads were removed by filtering, and then reaction was stopped then the reaction with ethanol-acetone mixture. After each lipase activity assay, the filtered beads were washed thoroughly with distilled water and stored at 4 °C for 1 h. Then the beads were reassayed for hydrolytic activity, and the same process was repeated until there is no activity in the beads.
The immobilized chitosan beads were stored in Tris-maleate buffer (0.2 M, pH 6.0) at 4 °C, and assayed in terms of their hydrolytic activities at regular time intervals.
All experiments were performed by repeating at least three times. The values of arithmetic mean and standard deviation values (± SD) were determined using Microsoft Office Excel program.
Results and discussion
In this study, the plant-based lipase isolated laurel seeds was immobilized within chitosan beads using entrapment method for the first time. The entrapment method is one of the methods commonly used for lipase immobilization. The major advantages of entrapment method are cheap, simple, and mild immobilization conditions (Desai et al. Citation2004, Kim et al. Citation2012, Pirozzi et al. Citation2009, Reetz et al. Citation1998, Won et al. Citation2005). For the enzyme immobilization, chitosan is a popular biomaterial which has biocompatibility, biodegradation, non-toxicity, high mechanical resistance, physically inertness. The chitosan have been frequently used as support material to immobilize the various enzymes including lipase (Alsarra et al. Citation2004, Altun and Çetinus Citation2007, Amorim et al. Citation2003, Chiou and Wu Citation2004, Foresti and Ferreira Citation2007, Huang et al. Citation2007, Palla et al. Citation2011). In this study, the entrapment of laurel lipase within chitosan beads was carried out according to the method of Alsarra et al. (Citation2004). Also optimum immobilization conditions required to entrap laurel lipase in beads were determined. Protein determination was done with Lowry method (y = 0.0018x + 0.0356, R2 = 0.9992). When immobilization within chitosan was carried out in optimum conditions, immobilization yield was determined to be as high as 78%. The enzymatic activity during immobilization is affected by several factors including the concentration of the support material, the concentration of the dropping solution, the amount of enzyme, amount of bead, and size bead. The immobilization conditions can highly increase immobilization efficiency, and also can improve the some properties of enzyme such as the reusability, thermal, and operational stability (Alsarra et al. Citation2004).
The SEM photomicrographs of the fresh tripolyphosphate (TPP)-chitosan beads and chitosan beads with lipase are shown in ). The morphologies of both chitosan beads are spherical, uniform and well dispersed. As seen in , when lipase immobilized in chitosan beads using TPP, their surface covered with enzyme molecules.
Figure 1. Scanning electron microscopy images of chitosan beads (a, c, e), immobilized lipase in chitosan beads (b, d, f) with different magnifications (130×, 1000×, 10,000×, respectively).
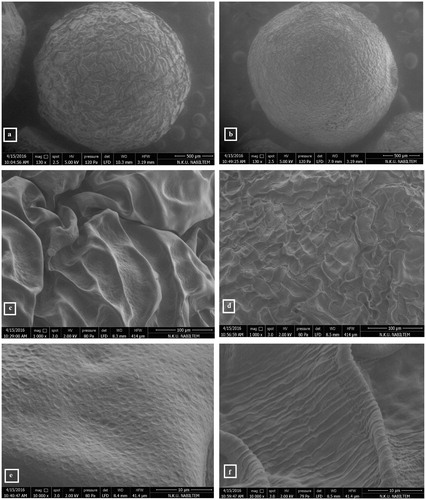
The chitosan concentration of immobilized beads was first optimized immobilization parameter. For this purpose; 150, 200, and 250 mg of chitosan were added to gel mixture to optimize, and hydrolytic activities of beads were assayed. The optimum activity was determined in the beads prepared using 250 mg of chitosan (). Because solution prepared with 250 mg of chitosan is too high viscosity, there was troublesome in the dropping process. Thus, the optimum amount of chitosan used to prepare beads was preferred as 200 mg. The number of chitosan molecules per unit solution increases, also increasing binding sites for TPP in higher chitosan concentrations. Increasing the number of crosslink bound possibly cause more densely chitosan gel structure. Consequently thinner walls in beads may be formed. Although the dense membrane structure is expected to create diffusion resistance for substrate input or product output, the reason of higher hydrolytic activities of beads prepared with 250 mg of chitosan may be decrease in the thicknesses of membrane capsules (Idris and Suzana Citation2006).
In the optimization study of dropping solution, TPP concentration and pH of Tris-HCl buffer (0.05 M) were determined. The concentrations of TPP solution assayed for this purpose were 0.3, 0.4 and 0.5% (w/v). pHs of Tris-HCl buffer were pH 6.5, 7.0 and 7.5. Optimum TPP concentration and optimum pH of Tris-HCl buffer were found to be 0.4% and 7.0, respectively ( and ).
Figure 3. Optimization of tripolyphosphate (TPP) concentration used as multivalent covalent counter ion in immobilization procedure.
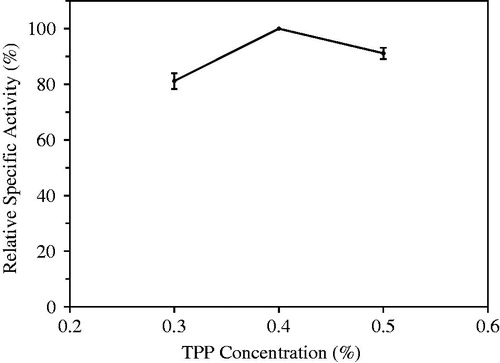
Figure 4. Optimization of buffer pH prepared tripolyphosphate (TPP) used as multivalent covalent counter ion.
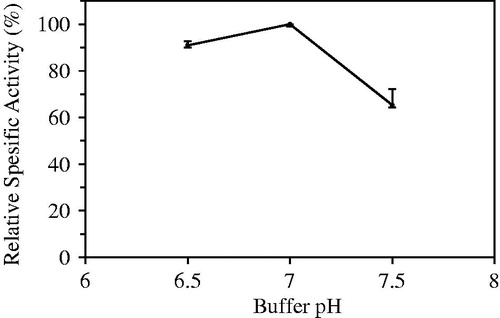
The preparation of cross-linking chitosan matrices using anions like TPP was found to be simpler and milder. Chitosan-TPP complexation may be affected both chitosan/TPP mass ratio and pH of buffer. pH of dropping solution determines chitosan protonation degree and therefore both its binding ability and its self-association, and volume ratio between the solutions (Nasti et al. Citation2009, Shu and Zhu Citation2002). Highest hydrolytic activity was observed when dropped gel mixture containing 200 mg of chitosan, 8 ml of 1% (v/v) acetic acid, and 2 mL laurel lipase solution to 0.4% (w/v) of TPP prepared in Tris-HCl buffer (pH 7.0).
Enzyme amounts (mL) containing 1, 2, and 3 mg protein were assayed to optimize the enzyme amount loaded immobilization procedure. The best hydrolytic activity was determined when enzyme amount was used as 2 mL in the entrapment procedure (). When enzyme amount loaded to chitosan beads was increased to 3 ml, enzymatic activities of beads decreased. The lower efficiencies were possibly observed blocking or inhibiting access to enzyme active sites due to molecular crowdedness within beads and surface of the matrix.
The immobilized beads were prepared using the syringe needles with the various diameters (0.45, 0.8, and 2 mm diameter) to determine the effect of bead size on the hydrolytic activity. The diameters of obtained beads were measured as 2.2, 2.9, and 4 mm. The highest hydrolytic activity was observed in beads having a diameter of 2.9 mm prepared using syringe needle with diameters of 0.8 mm (). Size of the needle used and the viscosity of the chitosan solution affect the diameter and the shape of the beads formed. The result showed that spherical beads had diameter of 2.9 mm, which allowed optimum diffusion for substrate input or product output.
The effect of bead amount on the hydrolytic activity was investigated using 0.25, 0.5, and 1 g of beads. Maximum activity was determined with 0.25 g of beads (). As seen in , specific activities decreased in increasing bead amounts (mg) due to possible saturation with substrate molecules of active sites in the constant substrate concentration.
Figure 7. Optimization of bead amount used in assays of hydrolytic activity catalyzed laurel lipase.
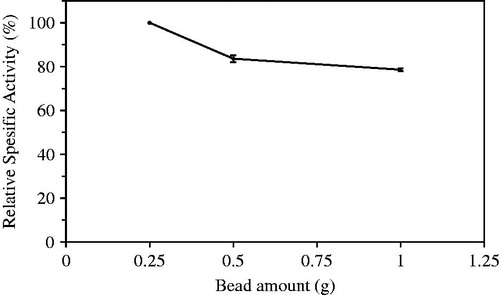
After determined optimization immobilization conditions, some properties of entrapped laurel lipase within chitosan beads including optimum pH, optimum temperature, Km and Vmax values, reusing, thermal and storage stability were determined.
Immobilization is a method that operating conditions of enzymes makes more moderate, and optimum pH of enzyme may be changed or expanded. 0.2 M Tris-maleat buffers in the pH range of 5.0–9.0 were used to determine optimum pH of immobilized laurel lipase. The most hydrolytic activity was seen at pH 6.0 ().
Candida rugosa lipase immobilized in chitosan was investigated hydrolytic activity of soybeans oil in the pH range of 5.0–11.0, and optimum pH was determined as 8.0 (Ting et al. Citation2006). Candida rugosa lipase was immobilized in chitosan, and its optimum pH was found to be 9.0 by Hung et al. (Citation2003). Pereira et al. (Citation2001) reported that optimum pH of immobilized Candida rugosa lipase was determined to be 6.0. Isbilir et al. (Citation2008) determined that the free laurel lipase exhibited maximum activity at 8.0 by using laurel oil as substrate.
When the study of optimum temperature was carried at out 30 °C, 40 °C and 50 °C, the most hydrolytic activity of immobilized laurel lipase was seen to be at 40 °C (). Optimum temperature for lipase isolated from Pentaclethra macrophylla was determined at 30 °C (Enujiugha et al. Citation2004). Candida rugosa lipase immobilized in chitosan by Pereira et al. (Citation2001) showed its most activity at 50 °C.
Thermal stability experiments were carried out for immobilized enzyme, which beads were incubated in the absence of substrate at various temperatures (40–80 °C). After waiting for 30 and 60 min, beads were placed in an ice bath. Then their hydrolytic activities were evaluated ().
The immobilized beads preserved about 50% of its initial hydrolytic activities at 60° while they preserved about 30% at 70 °C for 30 min. Chiou and Wu carried out the thermal stability study in the range of 25–60 °C for Candida rugosa lipase immobilized in chitosan with activated hydroxyl groups. Any activity loss was not observed for the immobilized lipase up to a temperature of 40 °C, and wet immobilized beads saved about 60% of activity at 60 °C (Chiou and Wu Citation2004).
Km and Vmax values of immobilized bay seed lipase was found by the Lineweaver-Burk plot (). For this purpose, experiments were carried out using the substrate solutions with the different concentrations prepared by weighing 0.25, 0.5 g, 0.6 g, and 0.75 g of olive oil. For immobilized bead, Km and Vmax values were determined 0.858 g and 27.25 U/mg protein, respectively. Km and Vmax values of free enzyme were 1.62 g and 4.66 U/mg protein, respectively. These values were found as 18.01 mM and 96.1 U/mg protein for Candida rugosa lipase entrapped in chitosan (Hung et al. Citation2003).
In the reusing assay, 1 g of immobilized beads was assayed for lipase hydrolytic activity. The laurel lipase entrapped in chitosan lost after two cycles. Huang et al. (Citation2007) determined that Candida rugosa lipase immobilized on chitosan-nanofibrous membrane saved 46% of its activity after 10 cycles.
In order to determine the storage stability of immobilized laurel lipase, immobilized beads stored at 4–8 °C were assayed every other day during 30 days, and beads saved their activities at this period (). 50% of the activities of beads remained for about 16 days. Candida rugosa lipase immobilized in chitosan stored at 25 °C saved 67% of relative activity during 7 days (Hung et al. Citation2003). Candida rugosa lipase immobilized in chitosan with activated hydroxyl groups preserved over 90% of their activities after 30 days (Chiou and Wu Citation2004).
Conclusion
Lipase isolated from laurel seeds was successfully entrapped in chitosan beads using tripolyphosphate. Effects of immobilization conditions on hydrolytic activities of laurel lipase were investigated. The immobilized laurel lipase had weak reusability while successful thermal and storage stability. Optimum pH and temperature were mild and economic for industrial applications, 6.0 and 40 °C, respectively. The kinetic parameters of immobilized lipase were better than those of free laurel lipase. Consequently, these immobilized beads by improving with additional experiments can be used in industrial processes.
Funding information
This research was funded by Research Fund of Trakya University (TUBAP-2011/157).
Disclosure statement
The authors report no conflicts of interest. The authors alone are responsible for the content and writing of this article.
References
- Aimée W, Alloue M, Destain J, El Medjoub T, Ghalfi H, Kabran P, Thonart P. 2008. Comparison of Yarrowia lipolytica lipase immobilization yield of entrapment, adsorption, and covalent bond techniques. Appl Biochem Biotechnol. 150:51–63.
- Ak G, Aktuna Y, Kartal F, Kilinc A. 2014. The effect of pretreatment with substrates on the activity of immobilized pancreatic lipase. Artif Cells Nanomed Biotechnol. 42:146–150.
- Alsarra IA, Neau SH, Howard MA. 2004. Effects of preparative parameters on the properties of chitosan hydrogel beads containing Candida rugosa lipase. Biomaterials. 25:2645–2655.
- Altun GD, Cetinus SA. 2007. Immobilization of pepsin on chitosan beads. Food Chem. 100:964–971.
- Amorim R, Melo E, Carneiro-da-Cunha M, Ledingham W, Campos-Takaki G. 2003. Chitosan from Syncephalastrum Racemosum used as a film support for lipase immobilization. Bioresour Technol. 89:35–39.
- Chiou SH, Wu WT. 2004. Immobilization of Candida rugosa lipase on chitosan with activation of the hydroxyl groups. Biomaterials. 25:197–204.
- Desai PD, Dave AM, Devi S. 2004. Entrapment of lipase into K-carrageenan beads and its use in hydrolysis of olive oil in biphasic system. J Mol Catal B Enzym. 31:143–150.
- Enujiugha VN, Thani FA, Sanni TM, Abigor RD. 2004. Lipase activity in dormant seeds of the African oil bean (Pentaclethra macrophylla Benth). Food Chem. 88:405–410.
- Foresti ML, Ferreira ML. 2007. Chitosan-immobilized lipases for the catalysis of fatty acid esterifications. Enzyme Microb Technol. 40:769–777.
- Hoppe A, Theimer RR. 1996. Titrimetric test for lipase activity using stabilized triolein emulsions. Phytochemistry. 42:973–978.
- Huang XJ, Ge D, Xu ZK. 2007. Preparation and characterization of stable chitosan nanofibrous membrane for lipase immobilization. Europ Poly J. 43:3710–3718.
- Hung TC, Giridhar R, Chiou SH, Wu WT. 2003. Binary immobilization of Candida rugosa lipase on chitosan. J Mol Catal B Enzym. 26:69–78.
- Idris A, Suzana W. 2006. Effect of sodium alginate concentration, bead diameter, initial pH and temperature on lactic acid production from pineapple waste using immobilized Lactobacillus delbrueckii. Process Biochem. 41:1117–1123.
- Isbilir SS, Ozcan HM, Yagar H. 2008. Some biochemical properties of lipase from bay laurel (Laurus nobilis L.) seeds. JAOCS. 85:227–233.
- Kara F, Demirel G, Tumturk H. 2006. Immobilization of urease by using chitosan-alginate and poly(acrylamide-co-acrylic acid)/kappa-carrageenan supports. Bioprocess Biosyst Eng. 29:207–211.
- Kim MH, An S, Won K, Kim HJ, Lee SH. 2012. Entrapment of enzymes into cellulose–biopolymer composite hydrogel beads using biocompatible ionic liquid. J Mol Catal B Enzym. 75:68–72.
- Klein MP, Nunes MR, Rodrigues RC, Benvenutti EV, Costa TMH, Hertz PF, Ninow JL. 2012. Effect of the support size on the properties of β-galactosidase immobilized on chitosan: advantages and disadvantages of macro and nanoparticles. Biomacromolecules. 13:2456–2464.
- Kumar V, Jahan F, Raghuwanshi S, Mahajan RV, Saxena RK. 2013. Immobilization of Rhizopus oryzae lipase on magnetic Fe3O4-chitosan beads and its potential in phenolic acids ester synthesis. Biotechnol Bioprocess Eng. 18:787–795.
- Lowry O, Rosenbrough N, Farr A, Randall R. 1951. Protein measurement with the folin phenol reagent. J Biol Chem. 193:265–275.
- Mohamed MA, Mohamed TM, Mohamed SA, Fahmy AS. 2000. Distribution of lipases in the gramineae. partial purification and characterization of esterase from Avena fatua. Bioresour Technol. 73:227–234.
- Nasti A, Zaki NM, de Leonardis P, Ungphaiboon S, Sansongsak P, Rimoli MG, Tirelli N. 2009. Chitosan/TPP and chitosan/TPP-hyaluronic acid nanoparticles: systematic optimisation of the preparative process and preliminary biological evaluation. Pharmaceut Res. 26:1918–1930.
- Palla CA, Paheco C, Carrin ME. 2011. Preparation and modification of chitosan particles for Rhizomucor miehei lipase immobilization. Biochem Eng J. 55:199–207.
- Pereira EB, Castro HFD, Moraes FFD, Zanin GM. 2001. Kinetic studies of lipase from Candida rugosa: a comparative study between free and immobilized enzyme onto porous chitosan beads. Appl Biochem Biotechnol. 91–93:739–752.
- Pimentel MCB, Leao ABF, Melo EHM, Ledingham WM, Lima JL, Sivewright M, Kennedy JF. 2007. Immobilization of Candida rugosa lipase on magnetized Dacron: kinetic study. Artif Cells Blood Substit Immobil Biotechnol. 35:221–235.
- Pirozzi D, Fanelli E, Aronne A, Pernice P, Mingione A. 2009. Lipase entrapment in a zirconia matrix: sol–gel synthesis and catalytic properties. J Mol Catal B Enzym. 59:116–120.
- Pulat M, Akalin GO, Karahan ND. 2014. Lipase release through semi-interpenetrating polymer network hydrogels based on chitosan, acrylamide and citraconic acid. Artif Cells Nanomed Biotechnol. 42:121–127.
- Pulat M, Akalin GO. 2013. GO Preparation and characterization of gelatin hydrogel support for immobilization of Candida rugosa lipase. Artif Cells Nanomed Biotechnol. 41:145–151.
- Reetz MT, Zonta A, Vijayakrishnan V, Schimossek K. 1998. Entrapment of lipases in hydrophobic magnetite-containing sol-gel materials: magnetic separation of heterogeneous biocatalysts. J Mol Catal A: Chem. 134:251–258.
- Rubin B, Dennis EA. 1997. Methods Enzymology: Lipases. Vol. 286. New York (NY): Academic Press, pp. 351–387.
- Sharma R, Soni SK, Vohra RM, Gupta LK, Gupta JK. 2002. Purification and characterisation of a thermostable alkaline lipase from a new thermophilic Bacillus sp. RSJ-1. Process Biochem. 37:1075–1084.
- Sheldon RA. 2007. Enzyme immobilization: the quest for optimum performance. Adv Synth Catal. 349:1289–1307.
- Shu XZ, Zhu KJ. 2002. Controlled drug release properties of ionically cross-linked chitosan beads: the influence of anion structure. Int J Pharm. 233:217–225.
- Siodmiak T, Ziegler-Borowska M, Marszall MP. 2013. Lipase-immobilized magnetic chitosan nanoparticles for kinetic resolution of (R, S)-ibuprofen. J Mol Catal B: Enzym. 94:7–14.
- Ting WJ, Tung KY, Giridhar R, Wu WT. 2006. Application of binary immobilized Candida rugosa lipase for hydrolysis of soybean oil. J Mol Catal B Enzym. 42:32–38.
- Toscano L, Montero G, Stotycheva M, Cervantes L, Gochev V. 2014. Comparison of the performances of four hydrophilic polymers as supports for lipase immobilisation. Biotechnol Biotechnol Equip. 28:52–60.
- Tumturk H, Demirel G, Altinok H, Aksoy S, Hasirci N. 2008. Immobilization of glucose isomerase in surface-modified alginate gel beads. J Food Biochem. 32:234–246.
- Villeneuve P, Muderhwa JM, Graille J, Haas MJ. 2000. Customizing lipases for biocatalysis: a survey of chemical, physical and molecular biological approaches. J Mol Catal B Enzym. 9:113–148.
- Won K, Kim S, Kim KJ, Park HW, Moon SJ. 2005. Optimization of lipase entrapment in Ca-alginate gel beads. Process Biochem. 40:2149–2154.
- Zhou GX, Chen GY, Yan BB. 2014. Biodiesel production in a magnetically-stabilized, fluidized bed reactor with an immobilized lipase in magnetic chitosan microspheres. Biotechnol Lett. 36:63–68.