Abstract
A stable and bio-compatible w/o nano-emulsion was developed as adjuvant for foot and mouth disease (FMD) vaccine with isopropyl myristate as oil phase, polyglycerol polyricinoleate, and Tween 80 as surfactants. Dissolving Tween 80 in aqueous phase decreased the droplet size and improved stability of the emulsion through narrowing size distribution, increasing viscosity, and reducing the interfacial tension between oil and water. The nano-emulsion was then formulated with inactivated FMD virus and ovalbumin to investigate its potential application as an adjuvant. Compared with commercial ISA-206 adjuvant, the novel w/o nano-emulsion was able to induce both humoral- and cell-mediated immune response.
Introduction
Foot and mouth disease (FMD) is a highly contagious disease that affects cloven-hoofed animals causing great economic losses in livestock field. Vaccination with inactivated FMD virus is the most effective way to control and eradicate FMD during an outbreak. However, failure to elicit effective immune responses by vaccination has been frequently reported (He et al. Citation2007, Wei Citation2007). There is a need to improve the available FMD vaccines in order to effectively protect animals from infection.
Using an adjuvant is an effective strategy to improve the immune response. Furthermore, the adjuvant may reduce the required amount of antigen or the multiple immunization. Mineral oil-based adjuvant is a traditional selection and has been used with the antigen in emulsion formulation. For FMD vaccine, emulsification of inactivated FMD with incomplete Freund adjuvant (IFA) has been found to protect pigs against the infection well (Cunliffe and Graves Citation1963, McKercher and Farris Citation1967, Michelsen Citation1961). Currently, the MontanideTM ISA series of oil-adjuvants (SEPPIC, France) is used for formulation of FMD vaccines in Asian and South American countries. Montanide ISA-206 renders a water-in-oil-in-water double emulsion, and Montanide ISA-201 is another newly developed oil-based adjuvant retaining the advantage of ISA-206 and adding some chemical components on the basis of ISA-206 to improve the cellular response, producing higher immunity responses (Dar et al. Citation2013).
However, all these adjuvants are based on mineral oils. There has been a concern about the mineral oil which is left in the animal body whose meat is for human consumption. Furthermore, studies have found that some mineral oil also has a bad effect to the animals, including swelling or necrosis at the injection site, or even systemic reaction affecting mainly the liver (Salk et al. Citation1953). The mineral oils comprise a mix of several hydrocarbons with different length of carbon chain. Study on relationship between carbon chain length and safety of adjuvants showed that shorter chains are efficient but induce local reactions, whereas longer chains are safer but less efficient (Stewart-Tull et al. Citation1976). Therefore, bio-compatible oils with intermediate carbon chain length are needed to make an ideal candidate for emulsions as adjuvant. Besides the quality of oil, properties of surfactants have also some effects on safety of the emulsion adjuvants (Gupta et al. Citation1993, Murray et al. Citation1972, Potter and Boyce Citation1962). Therefore, development of safer or better bio-compatible adjuvant is very important.
In this study, we aimed at developing a novel emulsion for FMD vaccine using a bio-compatible oil. Isopropyl myristate, an ester of fatty acid derived from plant oil such as coconut oil, is bio-compatible with chain length of 14 carbons that has been widely used in cosmetic and transdermal drug delivery due to its excellence in enhancing skin permeability and biosafety (Arellano et al. Citation1999, Goldberg-Cettina et al. Citation1995). A multiple microemulsion formulation based on isopropyl myristate with polyglycerol polyricinoleate (PGPR) as the surfactant has been tested as a potential adjuvant for rabies antigen. It was found that the rabies antigen with the micro-emulsion possessed a good potency against the virus, and there was no apparent local reaction (Leclercq et al. Citation2011).
The surfactant PGPR is considered to be GRAS (Substance Generally Recognized as Safe) by the FDA and has good biocompatibility and biodegradability (Wilson et al. Citation1998). Considering above-mentioned good properties of isopropyl myristate and PGPR, we should have a reason to believe that the system would be a novel candidate for FMD virus vaccine adjuvant.
However, initial experiments in this study discovered that the micro-emulsion prepared by isopropyl myristate with PGPR was not stable over a period of time. We then searched the literature and found that a variety of w/o emulsions had been prepared employing PGPR as the emulsifier, but the stabilities of these emulsions are limited. For instance, emulsions prepared with PGPR alone usually constitute water droplets larger than 1 μm with poor uniformity, as a consequence, its long-term stability is not good enough, limiting its application (Bonnet et al. Citation2009, Cheng et al. Citation2006, Fechner et al. Citation2007, Márquez et al. Citation2010, Su et al. Citation2006). Combination of surfactants or addition of stabilizers is a strategy to improve the emulsion stability, such as blending different affinity surfactants or adding biopolymers including proteins or polysaccharides (Krstonošić et al. Citation2009, Matos et al. Citation2014, Porras et al. Citation2004). Unfortunately, these efforts require extra high cost and safety consideration, which is undesirable for animal vaccines.
In order to solve the stability issue, we turned to some pharmaceutically approved surfactants, one of which is Tween 80. It is usually used as hydrophilic surfactant for preparing o/w emulsion. Because PGPR is a lipophilic surfactant, combination of Tween 80 with PGPR might be effective in adjusting the hydrophilic lipophilic balance (HLB) value, thus improving the stability of w/o emulsion. With this in mind, we started systematic investigation on combination of PGPR with Tween 80 in isopropyl myristate to develop a stable and effective bio-compatible oil emulsion. Thereafter, the obtained stable w/o emulsion was formulated with inactivated foot-mouth disease virus (FMDV) and a model antigen ovalbumin (OVA) to evaluate its potential as vaccine adjuvant.
Materials and methods
Materials
Isopropyl myristate (Chemical grade) was purchased from Sinopharm Chemical Reagent Co. (Beijing, China). Polyoxyethylene sorbitan monooleate (Tween 80, HLB =15.0) was purchased from Xilong Chemical Co. (Beijing, China). PGPR (HLB ≈1.5 ± 0.5, Food Grade) were supplied by Palsgaard Co. (Denmark). OVA (grade V) was purchased from Sigma Aldrich Co. (USA). Montanide ISA-206 was provided by SEPPIC (Shanghai) Specialty Chemicals Co., Ltd. FMDV strains O China 1999 vaccine and enzyme linked immunosorbent assay (ELISA) test kit were kindly provided by Lanzhou Veterinary Research Institute (Chinese Academy of Agricultural, China).
Emulsion preparation
PGPR was used as the lipophilic emulsifier to obtain the w/o emulsions. The external oil phase was prepared by dispersing certain amount of PGPR in isopropyl myristate under magnetic stirring at 600 rpm for 10 min. The aqueous phases were prepared by dissolving different amount of Tween 80 (w/w: 0, 1, 2, 3, 4, and 5%) in pure water. The w/o emulsions were prepared by adding the aqueous phase containing varied concentration of Tween 80 drop-wise into the oil phase containing PGPR; thereafter, the mixture was homogenized by a homogenizer (IKA® T18 digital ULTRA TURRAX®, Staufen, Germany) using S18N-10G rotor at 24,000 rpm for 5 min. For all the emulsion systems (total weight, 30 g), the weight ratio of aqueous phase to oil phase were set as 1:2, and all preparations were operated at room temperature.
To prepare emulsions-containing antigens, certain amount of OVA or FMDV was added previously to water phase containing 5% Tween 80. Then the water phase containing antigens was added to the oil phase containing 5% PGPR at aqueous phase to oil phase weight ratio of 1:2. After stirring at the rate of 24 000 rpm for 5 min, w/o emulsion system was formed. For the preparation of ISA-206 adjuvant emulsion, the volumetric ratio of aqueous phase to ISA-206 was set as 1:1 according to its specifications. For both emulsions, the final content of OVA and FMDV in the emulsion system was about 1 mg/ml and 10 μg/ml, respectively. The FMDV antigen concentration was determined by size-exclusion HPLC method, which was developed in our lab (Yang et al. Citation2015).
Characterization of the emulsions
Micrographs of freshly prepared emulsions were obtained with a Leica DMLB optical microscope (Leica Microsystems, GmbH; Germany), using an adapted digital camera (Leica DC100, Leica Microsystems, GmbH) operating at 400 × magnification.
Size distribution of water droplets of w/o emulsions was measured using a dynamic light scattering instrument (Zetasizer Nano ZSP, Malvern Instruments, Malvern, UK). Measurements were performed at 25 °C with duplicate measurement on each sample. To avoid multiple scattering during measurements, sample was diluted with isopropyl myristate and measured immediately.
The viscosity of the emulsions was measured with a DV-1 rotational viscometer (Shanghai Yueping Scientific Instruments Inc., Shanghai, China) at room temperature.
Interfacial tension between aqueous in the presence of different amount of hydrophilic surfactants and oil phase containing 5% PGPR was obtained with a K100 Krüss tensiometer (Hamburg, Germany) using a Wilhelmy plate method. Measurements were performed at room temperature.
Global stability of emulsions
Global stability of the w/o emulsions was evaluated by light scattering measurements, using a Turbiscan apparatus vertical scan analyzer (Formulaction, France). Emulsions without dilution were placed in the test cells, the equipment scans the sample along the sample height, giving profiles of back-scattering (%BS) and transmission percentage (%TS) as a function of time at room temperature. The optical reading head scanned the sample in cells and providing %BS and TS data every 40 μm as a function of the sample height (mm), gave a group of profiles data of the emulsion samples every half an hour. These data build up a macroscopic fingerprint of the emulsions at a given time, and showed useful information of changes in droplet size distribution or appearance of a creaming layer or sedimentation about the emulsions as a function of time.
Immunization of animals
Female BABL/c mice (6–8 weeks of age) were purchased from Beijing Vital Laboratory Animal Technology Company Ltd., (Beijing, China) and maintained with pathogen-free water and food. Groups of mice (5 mice per group) were immunized by injected intramuscularly (100 μl) in the upper quadriceps muscles of mice on days 1 and 14, with vaccine alone or vaccine with different emulsion formulations. Local reactions were recorded within 24 h after immunizations, and sera were collected at 4 weeks after immunizations. Sera sample were stored at −20 °C before further analysis. All animals were immunized with the equal amount of antigens. All procedures related to the animals and their throughout this study conformed to the internationally accepted principles as found in the Guidelines for Keeping Experimental Animals issued by the government of China.
Assay for serum antibodies
The level of total OVA-specific IgG antibodies was determined by enzyme linked immunosorbent assay (ELISA) method. Microplates (96 wells, Corning Inc., NY) were coated with OVA at an assertive concentration with 0.1 mM sodium carbonate (pH 9.5) and incubated at 4 °C overnight. Plates were washed three times with phosphate-buffered saline (PBS) with 0.5% Tween 20 (PBST) and then blocked with PBS-2% bovine serum albumin (BSA) for 1 h at 37 °C. After washing five times with PBST, serially diluted sera samples were added. After 3-h incubation at 37 °C, the plates were washed five times with PBST and peroxidase-conjugated goat-anti-mouse IgG (ABGENT, San Diego, USA) was added at a 1:1000 dilution to detect total IgG antibodies and incubated at 37 °C for 1 h. Then washing five times and color reaction was developed with TMB substrate solution at 37 °C for 10 min. The color reaction was stopped by adding 0.05 ml/well of 2 M H2SO4 and the optical densities (OD) were read at 450 nm (SunriseTM, TECAN, Switzerland) within 5 min.
The FMDV-specific IgG antibodies were tested using liquid-phase blocking ELISA kit for detecting FMD virus type O antibodies according to protocols provided by the producers.
T-cell proliferation
Single-cell suspensions were prepared from the spleens of the immunized animals employing mechanical dissociation 2 weeks after booster immunization. Spleen collected from immunized mice under aseptic conditions was minced and passed through a fine steel mesh to obtain a homogeneous cell suspension. After centrifugation (500 × g at 4 °C for 5 min), the pelleted cells were washed three times in RPMI-1640 and re-suspended in complete medium (RPMI 1640 supplemented with 100 IU/ml penicillin, 100 μg/ml streptomycin and 10% heat inactivated fetal calf serum). Cell numbers were counted with a cell counter (Scepter, Merck Millipore, USA) equipped with one-off chips. Spelenocytes were plated at 5 × 105 cells per well. Concanavalin A (Con A, type IV) was used as the mitogen and each well was set at 7.5 μg/ml; medium only was used for wells serving as a negative control. The cell cultures were incubated at 37 °C with 5% CO2 for 48 h. The colorimetric reaction was performed according to the Cell Counting Kit-8 (CCK-8, Dojindo, Japan) protocol. Each well was added 10 μl CCK-8 solution and was further cultured for 4 h. The OD value was read at 450 nm. Stimulation index (SI) was calculated as the ratio of OD450 nm of Con A stimulated culture to that of the unstimulated culture.
Stability of nano-emulsion with antigens
The long-term stability of nano-emulsion formulated with antigens (FMDV and OVA) was evaluated by monitoring changes in the particle size using dynamic light scattering instrument over 2-month period. The emulsions were stored at 4 °C in refrigerator.
Effect of emulsification on FMDV antigen
The w/o emulsion formulated with FMDV was centrifuged at 12,000 rpm for 15 min to get phase separation. FMDV antigen content in the aqueous phase recovered from the emulsion was quantitatively determined by size-exclusion HPLC method developed in our lab (Yang et al. Citation2015). The antigen content in aqueous phase before being emulsified with oil phase was also determined for comparison.
Statistical analysis
Data analysis was performed with GraphPad Prism and Origin software. Values were expressed as the mean ± standard deviation (SD). Statistical significance of difference was determined by the unpaired Student’s t-test. P values of less than 0.05 were considered statistically significant.
Results and discussion
Effects of PGPR concentrations on the w/o emulsions
shows the size distribution of the w/o emulsions prepared at three different concentrations of PGPR in oil phase (isopropyl myristate) and with no additions in the aqueous phase. At low PGPR concentration of 1%, a very broad bi-modal size distribution was observed, with the major average droplet size about 3114.60 nm. As the increase of the PGPR content to 5%, the emulsion showed a monomodal distribution of sizes, with average diameter of 2266.87 nm, significantly smaller than that prepared at 1% PGPR. Further increase the PGPR concentration to 10%, droplet size decreased to 1959.52 nm, and the uniformity of the emulsion was also improved as evidenced from the narrower size distributions. The effect of PGPR concentration on droplet size of w/o emulsions was in agreement with results observed in some of other w/o emulsion systems (Márquez et al. Citation2010), where droplet size decreased from 2.94 μm to 1.27 μm with the PGPR concentration from 0.2% to 1.0%.
Figure 1. Droplet size distribution of w/o emulsions prepared with different concentrations of PGPR in oil phase.
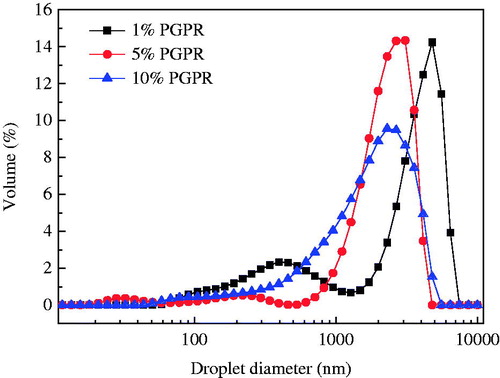
Despite of the fact that PGPR is considered to be GRAS by the FDA, its quantity in the emulsion should be as low as possible. Moreover, changes in average droplet diameter for PGPR between 1% and 5% are more intensive than the changes when PGPR concentration was from 5% to 10%. Overall, in consideration of safety and economic factors, 5% PGPR was used for emulsion in the following experiment.
The microstructure of the w/o emulsion prepared with 5% PGPR was observed by optical microscope as shown in . It can be seen that some aggregated water droplets were formed in the emulsion. After 3 months of storage at room temperature, serious phase separation was observed as shown in . It is obvious that PGPR alone could not stabilize the emulsion for long period.
Figure 2. Optical micrographs of w/o emulsions prepared with 5% PGPR in oil phase, immediately after preparation. Without adding Tween 80 in aqueous phase: (a) With different concentrations of Tween 80 in aqueous phase: (b) 1% Tween 80; (c) 2% Tween 80; (d) 3% Tween 80; (e) 4% Tween 80; (f) 5% Tween 80. Bar = 5 μm.
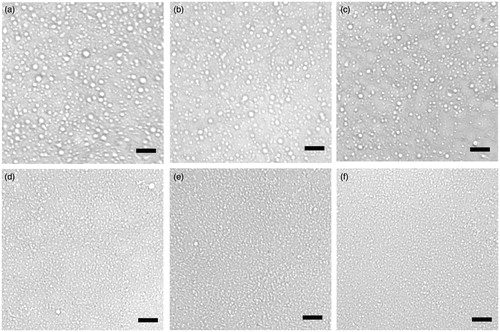
Effect of Tween 80 on w/o emulsions
Using PGPR alone could not obtain w/o nano-emulsion from the data we measured. To prepare a nano-scale w/o emulsion and improve its stability, we then tried to include different amount of Tween 80 in aqueous phase as co-emulsifier. are the optical micrographs of the emulsions prepared with 5% PGPR in oil phase and in collaboration with different concentrations of Tween 80 in aqueous phase. Compared with the emulsion prepared with PGPR alone (), the droplet size became smaller and the uniformity of the droplets was much improved with the increase of Tween 80 concentration from 1% to 5%, as manifested by a smaller mean droplet diameters listed in . The droplet size distribution of w/o emulsions with additional Tween 80 in aqueous phase was also presented in . The average droplet size of the emulsion prepared with PGPR was about 2266.88 nm, by adding 5% Tween 80 in aqueous phase, the average droplet size was reduced to about 129.85 nm. The addition of Tween 80 not only decreased the droplets size and improved the uniformity of the droplets, but also led to significant enhancement in long-term storage stability. As shown by the pictures in , the emulsion prepared with PGPR appeared obvious phase separation due to sedimentation of water droplets at the bottom during storage. By increasing the concentration of Tween 80 from 1% to 5%, the situation improved greatly. No apparent phase separation occurred, and the nano-emulsion remained rather stable after 3-month storage.
Figure 4. Appearance of w/o emulsions prepared with 5% PGPR in oil phase with different concentration of Tween 80 in aqueous phase after being stored at room temperature for 3 months.
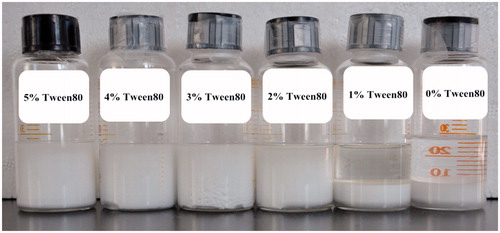
Table 1. Average droplets size of w/o emulsions with 5% PGPR in isopropyl myristate as oil phase and different amount of Tween 80 in water phase.
Global stability of w/o emulsions
shows the %BS profiles for the w/o emulsions prepared with 5% PGPR in oil phase, with and without addition of Tween 80 in the aqueous phase. From the obtained profiles, the mean values of initial %BS were calculated in the 10–30 mm zone (%BSin10–30) corresponding to the medium part of the tube. The %BSin10–30 values of emulsion without Tween 80 addition was about 70.5, significantly lower than the values measured with the emulsions containing different Tween 80 concentrations, which are in the ranges of 81.6 to 84.5. The absolute value of %BS is directly related to the water droplet size of a w/o emulsion, lower %BS implying an increase of droplet size (Márquez et al. Citation2007). Therefore, the observation of %BSin10–30 that increased along with the addition of Tween 80 was well in consistence with the results of optical micrographs of the emulsions shown in , also with their average water droplets size listed in . Analysis on the %BS kinetic profiles gave more useful information about the global stability of the w/o emulsions. During 24-h measurement, %BS of the emulsion without Tween 80 decreased gradually along the tube, evidencing an increase of water droplets size due to a coalescence process. On the other hand, the %BS at the bottom of the tube increased at the expense of %BS diminution on the top part, indicating a sedimentation process of the water droplets. In contrast to the simultaneous occurrence of coalescence and sedimentation which started at a fast speed in the emulsion without Tween 80 (), the %BS kinetic profiles of the emulsion with addition of 1% to 5% Tween 80 showed that both the coalescence and sedimentation were highly suppressed according to the observation until 24 h (). There were almost no changes in the %BS profiles during 24-h measurement, indicating that the water droplets size of those emulsions was not sufficiently increased.
Figure 5. Back-scattering (BS%) profiles of w/o emulsions with different concentrations of Tween 80 dispersed in water as a function of time and tube length. Concentration of Tween 80: (a) 0%, (b) 1%, (c) 2%, (d) 3%, (e) 4%, and (f) 5%.
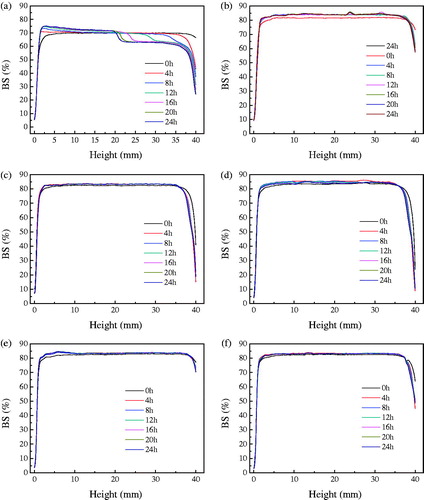
For better comparison of these emulsions stability, another parameter Turbiscan Stability Index, TSI, was used to gauge the stability of emulsions. The TSI was defined as follows:
The TSI values change in the range of 0–100 reflects the deviation of multiple scans of the light intensity received from the emulsions. Therefore, according to this rule, the higher the TSI value, the worse stability of the emulsions.
Plotting TSI as a function of time during 24-h measurements is shown in . It can be seen that TSI values of all w/o emulsions increased as a function of time, suggesting that there was particle migration (sedimentation) due to coalescence or particle aggregation (flocculation) (Mengual et al. Citation1999). Nevertheless, the increase in TSI of the emulsion without addition of Tween 80 was significantly higher than others with different concentration of Tween 80. Moreover, there was a tendency of decreasing TSI values when increasing the concentration of Tween 80 dispersed in water.
Figure 6. TSI values of w/o emulsions with different Tween 80 in aqueous phase as a function of time during 24 h.
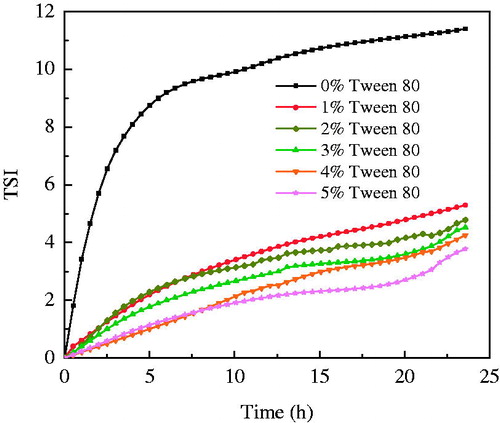
Based on above discussion on the destabilization mechanism of the w/o emulsion prepared in the absence of Tween 80, it can be concluded that the poor stability was mainly resulted from large size of the water droplets, and simultaneous occurrence of coalescence and sedimentation of the water droplets. On the other hand, the Tween 80 dispersed in aqueous phase was of prime importance to stabilize the w/o emulsions through cooperation with PGPR in oil phase. As demonstrated by results shown in and , such synergistic stabilization mechanism was manifested by the lower droplet size and higher uniformity in size distribution, which can contributes to the stability of emulsion by reducing the coalescence process as a consequence of the lower collision efficiency (McClements Citation2004).
Emulsion viscosity and interfacial property
Besides the above-mentioned stabilization mechanism afforded by Tween 80 added in aqueous phase, its influences on some other properties which are critical to the stability of emulsion, like viscosity and interfacial tension, might also be important. High viscosity was reported usually favorable to the stability of w/o emulsion by reducing rates of coalescence and sedimentation of water droplets (Su et al. Citation2006). presents the viscosities of the emulsions prepared with different concentrations of Tween 80 in aqueous phase. Clearly, addition of Tween 80 led to the increase in viscosity of emulsions from 25.5 mPa.s to 32.5 mPa.s, which could contribute to the stability of emulsions as reported by others (Zhu et al. Citation2015). In contrast, shows that the interfacial tension between aqueous phase and oil phase containing 5% PGPR decreased with the increase of Tween 80 concentration in aqueous phase. The interfacial tension at equilibrium decreased from 1.0 mN·m−1 in the absence of Tween 80 to 0.6 mN·m−1 when 5% Tween 80 was added in the aqueous phase. In one aspect, the decreased interfacial tension could contribute to the stability by lowering the droplet size, as demonstrated by the results listed . Second, the PGPR has a relative large hydrophilic portion constituted by polyglycerol moieties, which can bind to the water molecules by hydrogen bonds (Ushikubo and Cunha Citation2014). With the decrease of interfacial tension, more PGPR molecules could be adsorbed at the interface, and possible extra hydrogen bond would be formed between the hydrophilic portions in Tween 80 and PGPR, so that more complex interfacial film were formed to prevent coalescence of the water droplets. Such mechanism was considered similar to the synergistic stabilizing effects of dispersing proteins in aqueous phase for preparing w/o emulsions. Some proteins, like BSA (Gaiti et al. Citation1994) and β-lactoglobulin (Gülseren and Corredig Citation2012), have been proven to be able to increase the thickness of interfacial film in w/o emulsion, thus enhancing the stability.
Potential adjuvant effect of nano-emulsion formulation
The nano-emulsion was then formulated with FMDV antigen to determine its potential adjuvant efficacy. The total average antibody titer induced in sera collected on 28-d post-immunization was measured and results were shown in . The antibody titers in the groups vaccinated with FMDV formulated with our nano-emulsion and ISA-206 were significantly higher as compared to the control group immunized with antigen alone (P < 0.05). While there was no significant difference in the nano-emulsion group and the IS-206 group, demonstrated that our w/o nano-emulsion had approximate efficacy in inducing specific antibody response against FMDV with ISA-206 adjuvant. Since the protective efficacy of vaccination is strictly correlated to the immune response, and generation of strong specific antibody response play primary role in clinical protection from the external virus infection (Shi et al. Citation2007, Xiao et al. Citation2007), the increased serum specific IgG titers induced by w/o nano-emulsion would confer higher levels of protection against FMD virus.
Figure 8. Immune response in mice immunized with FMDV in w/o nano-emulsion and ISA-206 formulations. Statistical significance of difference was determined by the unpaired Student’s t-test. (A) IgG titers shown as means of IgG titer ± SD. *P < 0.05 compared to FMDV antigen alone group; P > 0.05 compared to ISA-206 group. (B) Splenocyte proliferative responses to Con A, shown as a mean stimulation index (SI). *P < 0.05 compared to FMDV antigen alone group and #P < 0.05 compared to ISA-206 group.
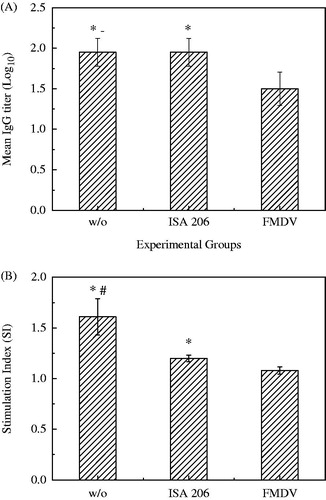
To better evaluate the potential efficacy of nano-emulsion as adjuvants, proliferation of splenocytes isolated from animals immunized with FMDV antigens was measured. presents the results of splenocytes proliferative responses to Con A in cultures established from animals immunized with FMDV. The mean SI in the group immunized with our nano-emulsion formulation was statistically higher than both groups immunized with ISA-206 formulation and antigen alone. Whereas the difference between groups vaccinated with ISA-206 formation and antigen alone was not sufficiently significant (P = 0.0413). This suggests that our w/o nano-emulsion is even more potent in enhancing the T cell response than the ISA-206.
The w/o nano-emulsion was also applied to a model antigen OVA to make further evaluation on its adjuvant effect. Again, higher humoral immune response and cellular response were observed with our nano-emulsion adjuvant compared to the ISA-206 adjuvant, as manifested by the higher level of specific IgG titer and proliferation of splenocytes presented in . Moreover, no any severe local secondary action was found in the immunization period.
Figure 9. Immune response in mice immunized with OVA in w/o nano-emulsion and ISA-206 formulations. Statistical significance of difference was determined by the unpaired Student’s t-test. (A) IgG titers shown as means of IgG titer ± SD. *P < 0.05 compared to OVA alone group and #P < 0.05 compared to ISA-206 group. (B) Splenocyte proliferative responses to Con A, shown as a mean stimulation index (SI). *P < 0.05 compared to OVA alone group and #P < 0.05 compared to ISA-206 group.
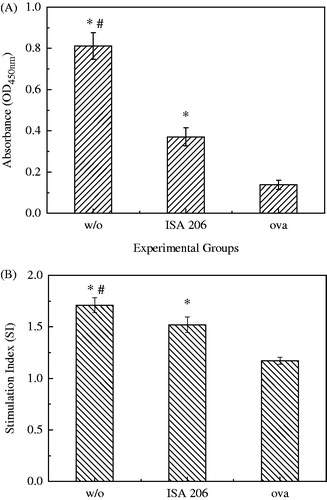
Figure 10. Changes in particle size of the nano-emulsions formulated with (A) OVA and (B) FMDV antigens during 2 months storing at 4 °C in refrigerator.
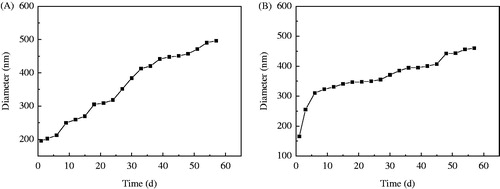
Based on results shown in and , the w/o nano-emulsion showed good potential as an adjuvant as validated by experiments involving two different antigen (FMDV and OVA). As regards FMDV vaccine, the w/o nano-emulsion exhibited similar capability in stimulating humoral-mediated immune response to the widely used ISA-206 oil adjuvant, while its capability in inducing cell-mediated immune response was even superior to the later one. As the most widely used commercial oil adjuvant, the ISA-206 is certainly more effective than conventional Al(OH)3/saponin-based adjuvant in terms of generating higher and longer lasting immune responses (Cao Citation2014). However, the less efficacy of ISA-206 in inducing cell-mediated immune response and consequent insufficient protective efficacy in cattle against FMD virus had been frequently reported in recent years (Cao Citation2014, Dar et al. Citation2013, Park et al. Citation2014, Terhuja et al. Citation2015). More recently, Terhuja et al. compared efficacy of recombinant FMD virus-like particles (VLPs) adjuvant with ISA-206 and several of other chemical adjuvants including CpG (Terhuja et al. Citation2015). The cell-mediated immune response in VLP + ISA-206 was weak and its protective efficacy was unsatisfactory. Therefore, developing new adjuvant that can induce high level of both specific antibody and cell-mediated immune response are desired and receiving wide attentions (Cao Citation2014, Jin et al. Citation2004, Park et al. Citation2014, Terhuja et al. Citation2015). The SEPPIC Inc. has developed a newly mineral oil-based with commercial name of Montanide ISA-201 by adding some chemical components on the basis of ISA-206 to improve the cellular responses. Results from comparative studies had proven that as compared to ISA-206, the inactivated FMD vaccines formulated with the ISA-201 adjuvant could induce earlier and higher neutralizing antibody responses, and higher cellular immunity and better protective efficacy in cattle (Dar et al. Citation2013). However, such improvement is based on addition of extra chemicals. In comparison, our nano-emulsion formulation is made from plant oil such as coconut oil, with much better biocompatibility than the mineral oil in ISA-206 and ISA-201. The emulsifiers PGPR and Tween 80 are pharmaceutically approved surfactants. And more importantly, our nano-emulsion formulation had shown good potent in inducing humoral- and cell-mediated immune response, therefore we believe it can be a good candidate of oil-based adjuvant for food animal by providing better biocompatibility and safety. Of course, more detailed research is needed to study the immunological aspects of the nano-emulsion adjuvant, such as the kinetics of specific antibody response, the cytokine secretion, and long-lasting protection efficacy, etc.
Stability of nano-emulsion with antigens and effect of emulsification on FMDV antigen
The nano-emulsion formulated with FMDV and OVA antigens also showed good stability. Over 60-d storage, the particle size was still in nano-scale (below 500 nm), and no creaming or phase separation was observed, though gradual increase in particle size was detected due to particle movement flocculation.
It has been known that the inactivated FMDV, which has a sedimentation coefficient of 146S, is crucial to the efficacy of vaccine preparations, but extremely unstable in vitro. It is prone to dissociate into smaller particles referred to as 12S with a concomitant decrease in immunogenicity (Rao et al. Citation1994). During preparation of the nano-emulsion, there was a high shear force that may cause dissociation of the 146S. Therefore, the 146S content in aquous phase before and after emulsification was determined with size exculsion HPLC analysis according to protocol developed in our lab (Yang et al. Citation2015). The HPLC chromtogaphs shown in indicated that the FMDV recoverd from nano-emulsion was eluted at the same time as that of before emulsification, indicating there was no occurrence of aggragation or dissociation in 146S during emulsification, thus its immunogenicity would be affected. Using the previouly established standard curve, the FMDV was quntatively detmermined and the recovery ratio was calulacated to be about 83.7% by comparing the total amount of FMDV found in aqeuous phase separated from the emulsion through centrifugation with its initial amount in the aqeuous phase before being emulsified with the oil phase. Since phase separation by centrifugation might not be complete, the FMDV revovery ratio of 83.7% was considered high enough. From this aspect, FMDV antigen was considered well retained during the emulsification process and in the nano-emulsion.
Conclusions
We developed a stable and bio-compatible w/o nano-emulsion to be used as adjuvant for FMD vaccine with isopropyl myristate as oil phase, PGPR and Tween 80 as surfactants, which were dissolved separately in oil and in water before mixing them together. It was found that addition of a second surfactant Tween 80 could significantly improve the emulsion stability, through decreasing the droplet size, narrowing size distribution, increasing viscosity of emulsions, and reducing the interfacial tension between oil and water. All these four aspects played important roles in inhibiting the occurrence of coalescence and sedimentation of the water droplets.
The stable w/o nano-emulsion showed good potential as a novel oil-based adjuvant. Compared to ISA-206, the w/o nano-emulsion formulated with two antigens (FMDV and OVA) induced comparable specific antibody titers, but statistically higher level of cellular-mediated immune response. Considering its good biocompatibility and safety of the natural lipid-derived oil, our w/o nano-emulsion might be a good alternative to conventional mineral oil based ISA-206 adjuvant. Therefore, this work would contribute not only to the preparation of a more stable w/o nano-emulsion by using a hydrophilic surfactant Tween 80 in aqueous phase, but also contribute to the knowledge on production of novel oil-based nano-emulsion adjuvants. Further researches will be carried to study the immunological aspects of the nano-emulsion adjuvant, such as the kinetics of specific antibody response, the cytokine secretion, and long-lasting protection efficacy, etc.
Funding information
We are grateful for the financial support from the National Natural Science Foundation of China (21336010), the National High Technology Research and Development Program of China (863 Program, 2014AA021005, 2014AA021006), and National Science and Technology Major Project (2013ZX10004606).
Acknowledgements
We gratefully acknowledge LDS Technology Limited Company (Beijing, China) for their assistance in the emulsions global stability measurements.
Disclosure statement
The authors report no conflicts of interest. The authors alone are responsible for the content and writing of this article.
References
- Arellano A, Santoyo S, Martın C, et al. 1999. Influence of propylene glycol and isopropyl myristate on the in vitro percutaneous penetration of diclofenac sodium from carbopol gels. Eur J Pharm Sci. 7:129–135.
- Bonnet M, Cansell M, Berkaoui A, et al. 2009. Release rate profiles of magnesium from multiple W/O/W emulsions. Food Hydrocolloids 23:92–101.
- Cao Y. 2014. Adjuvants for foot-and-mouth disease virus vaccines: recent progress. Exp Rev Vaccines 13:1377–1385.
- Cheng CJ, Chu LY, Xie R. 2006. Preparation of highly monodisperse W/O emulsions with hydrophobically modified SPG membranes. J Colloid Interface Sci. 300:375–382.
- Graves JH. 1963. Formalin-treated foot-and-mouth disease virus: comparison of two adjuvants in cattle. Can J Comp Med Vet Sci. 27:193–197.
- Dar P, Kalaivanan R, Sied N, et al. 2013. Montanide ISA™ 201 adjuvanted FMD vaccine induces improved immune responses and protection in cattle. Vaccine 31:3327–3332.
- Fechner A, Knoth A, Scherze I, et al. 2007. Stability and release properties of double-emulsions stabilised by caseinate–dextran conjugates. Food Hydrocolloids 21:943–952.
- Gaiti N, Aserin A, Cohen Y. 1994. Mechanistic considerations on the release of electrolytes from multiple emulsions stabilized by BSA and nonionic surfactants. J Control Release 29:41–51.
- Goldberg-Cettina M, Liu P, Nightingale J, et al. 1995. Enhanced transdermal delivery of estradiol in vitro using binary vehicles of isopropyl myristate and short-chain alkanols. Int J Pharmaceut. 114:237–245.
- Gülseren İ, Corredig M. 2012. Interactions at the interface between hydrophobic and hydrophilic emulsifiers: polyglycerol polyricinoleate (PGPR) and milk proteins, studied by drop shape tensiometry. Food Hydrocolloids 29:193–198.
- Gupta RK, Relyveld EH, Lindblad EB, et al. 1993. Adjuvants – a balance between toxicity and adjuvanticity. Vaccine 11:293–306.
- He QG, Chen HC, Wu B, et al. 2007. Detection of serum antibody against hog cholera, porcine parvovirus and the foot-and-mouth disease on 44 pig farms of six provinces. Chin J Pre Vet Med. 22:5–9.
- Jin H, Li Y, Ma Z, et al. 2004. Effect of chemical adjuvants on DNA vaccination. Vaccine 22:2925–2935.
- Krstonošić V, Dokić L, Dokić P, et al. 2009. Effects of xanthan gum on physicochemical properties and stability of corn oil-in-water emulsions stabilized by polyoxyethylene (20) sorbitan monooleate. Food Hydrocolloids 23:2212–2218.
- Leclercq SY, dos Santos RMM, Macedo LB, et al. 2011. Evaluation of water-in-oil-in-water multiple emulsion and microemulsion as potential adjuvants for immunization with rabies antigen. Eur J Pharm Sci. 43:378–385.
- Márquez AL, Palazolo GG, Wagner JR. 2007. Water in oil (w/o) and double (w/o/w) emulsions prepared with spans: microstructure, stability, and rheology. Colloid Polym Sci. 285:1119–1128.
- Márquez AL, Medrano A, Panizzolo LA, et al. 2010. Effect of calcium salts and surfactant concentration on the stability of water-in-oil (w/o) emulsions prepared with polyglycerol polyricinoleate. J Colloid Interface Sci. 341:101–108.
- Matos M, Gutiérrez G, Coca J, et al. 2014. Preparation of water-in-oil-in-water (W 1/O/W 2) double emulsions containing trans-resveratrol. Colloid Surface A 442:69–79.
- McClements DJ. 2004. Food Emulsions, Principles, Practices and Techniques. Boca Raton (FL): CRC Press Inc.
- McKercher PD, Farris Jr HE. 1967. Foot-and-mouth disease in swine: response to inactivated vaccines. Arch Gesamte Virusforsch. 22:451–461.
- Mengual O, Meunier G, Cayré I, et al. 1999. TURBISCAN MA 2000: multiple light scattering measurement for concentrated emulsion and suspension instability analysis. Talanta 50:445–456.
- Michelsen E. 1961. Experience with vaccination of pigs. Arch Exp Vet Med. 15:317–321.
- Murray R, Cohen P, Hardegree MC. 1972. Mineral oil adjuvants: biological and chemical studies. Ann Allergy 30:146–151.
- Park ME, Lee SY, Kim RH, et al. 2014. Enhanced immune responses of foot-and-mouth disease vaccine using new oil/gel adjuvant mixtures in pigs and goats. Vaccine 32:5221–5227.
- Porras M, Solans C, Gonzalez C, et al. 2004. Studies of formation of W/O nano-emulsions. Colloid Surface A 249:115–118.
- Potter M, Boyce CR. 1962. Induction of plasma-cell neoplasms in strain BALB/c mice with mineral oil and mineral oil adjuvants. Nature 193:1086–1087.
- Rao MG, Butchaiah G, Sen AK. 1994. Antibody response to 146S particle, 12S protein subunit and isolated VP1 polypeptide of foot-and-mouth disease virus type Asia-1. Vet Microbiol. 39:135–143.
- Salk JE, Contakos M, Laurent AM, et al. 1953. Use of adjuvants in studies on influenza immunization. III. Degree of persistence of antibody in human subjects two years after vaccination. J Am Med Assoc. 151:1169–1175.
- Shi XJ, Wang B, Wang M. 2007. Immune enhancing effects of recombinant bovine IL-18 on foot-and-mouth disease vaccination in mice model. Vaccine 25:1257–1264.
- Stewart-Tull DES, Shimono T, Kotani S, et al. 1976. Immunosuppressive effect in mycobacterial adjuvant emulsions of mineral oils containing low molecular weight hydrocarbons. Int Arch Allergy Appl Immunol. 52:118–128.
- Su J, Flanagan J, Hemar Y, et al. 2006. Synergistic effects of polyglycerol ester of polyricinoleic acid and sodium caseinate on the stabilisation of water–oil–water emulsions. Food Hydrocolloids 20:261–268.
- Terhuja M, Saravanan P, Tamilselvan RP. 2015. Comparative efficacy of virus like particle (VLP) vaccine of foot-and-mouth-disease virus (FMDV) type O adjuvanted with poly I:C or CpG in guinea pigs. Biologicals 43:437–443.
- Ushikubo FY, Cunha RL. 2014. Stability mechanisms of liquid water-in-oil emulsions. Food Hydrocolloids 34:145–153.
- Wei DS. 2007. Discussion on the failure of vaccination in animals. J Anim Sci Vet Med. 26:33–34.
- Wilson R, Van Schie BJ, Howes D. 1998. Overview of the preparation, use and biological studies on polyglycerol polyricinoleate (PGPR). Food Chem Toxicol. 36:711–718.
- Xiao C, Rajput ZI, Hu S. 2007. Improvement of a commercial foot-and-mouth disease vaccine by supplement of Quil A. Vaccine 25:4795–4800.
- Yang Y, Li H, Li Z, et al. 2015. Size-exclusion HPLC provides a simple, rapid, and versatile alternative method for quality control of vaccines by characterizing the assembly of antigens. Vaccine 33:1143–1150.
- Zhu Q, Li J, Liu H, et al. 2015. Development of stable water-in-oil emulsions using polyglycerol polyricinoleate and whey protein isolate and the impact on the quality of bittern-tofu. J Disperse Sci Technol. 36:1548–1555.