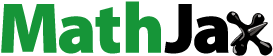
Abstract
The objective of this study was to investigate the recovery ability of polymerized porcine hemoglobin (pPolyHb) in a rat model of acute anemia caused by normovolemic hemodilution (ANH). After the ANH procedure, rats were infused with either pPolyHb or red blood cells. The results showed pPolyHb could carry a sufficient amount of oxygen to the tissues to maintain normal aerobic metabolism and hemodynamic stability, without any significant toxic effects on renal and liver function according to pathological, and biochemical analysis. The data suggest pPolyHb may be a good candidate for the treatment of acute anemia in future clinical trials.
Introduction
Acute normovolemic hemodilution (ANH) is a potential strategy to reduce the need for allogeneic blood transfusions during the perioperative period (Murray Citation2004). ANH consists of withdrawing a percentage of the patient’s blood volume and simultaneously infusing acellular fluids in order to maintain the original volume. During ANH, both hematocrit (Hct) and arterial O2 content decrease, and the patient becomes anemic. As anemia progresses, the patient’s tissue oxygenation is maintained over a large Hct range because of increasing cardiac output, redistribution of regional organ blood flows, and enhanced tissue O2 extraction (Ickx et al. Citation2000). When the hemoglobin concentration falls below a critical level, however, oxygen debt occurs with initiation of anaerobic metabolism and inefficient cell function (Vallet Citation2010).
Anemia resulting from clinical conditions or loss of blood is usually treated by blood transfusion to improve the supply of oxygen to hypoxic tissues and organs. When a patient’s Hct reaches a critically low level, the transfusion of allogeneic red blood cells (RBC) is warranted (Walsh et al. Citation2006). Retrospective analyses of hospital databases (Carson et al. Citation2002) and case reports (Wu et al. Citation2001) regarding mortality associated with severe acute anemia have suggested that the median hemoglobin value below, which death occurs is 5 g/dL. Although allogeneic blood transfusion can be life-saving, there are some risks associated with this procedure, which include accidental mistransfusion, transmission of infectious diseases, and transfusion-related lung injury (Harrington et al. Citation2007). Additionally, RBC are not always readily available, so RBC transfusion may not always be an option to treat severe anemia.
Hemoglobin based oxygen carriers (HBOCs), derived from either human or bovine hemoglobin, are able to transport O2, and therefore, have the potential to reduce the need for allogeneic blood transfusions (Bonegio et al. Citation2009). HBOC-201, which is purified bovine hemoglobin cross-linked and polymerized with glutaraldehyde, has been approved for the treatment of acute surgical anemia in South Africa since 2001 and in Russia since late 2010. Promising studies in swine with serial normovolemic exchange transfusion have demonstrated that the administration of HBOC-201 was capable of maintaining tissue oxygenation in a clinically relevant large animal model, in which the Hct was reduced by approximately 50% due to hemodilution (Muir et al. Citation2011). In humans, post hoc analysis suggested that administration of HBOC-201 to acutely bleeding and hemolyzing patients with life-threatening anemia, when RBC were not readily available or transfusion was not an option, was associated with improved chances of survival (Mackenzie et al. Citation2010). A multicenter phase III trial of human polymerized hemoglobin (PolyHeme, Northfield Laboratories), designed to assess survival of patients at the scene of injury when blood is needed but not available, documents that PolyHeme has life-sustaining capability (Moore et al. Citation2009) . The combination of PolyHeme’s oxygen-carrying capacity, its logistical benefits, and its acceptable benefit-to-risk profile, suggest its use as an alternative to transfusion for patients at high risk of death, when stored RBCs are not available. Polyethylene glycol maleimide-conjugated Hb (PEG-Hb) has been shown to be more effective than earlier formulations of blood substitute based on mimicking blood O2 transport properties (high Hb concentration and normal O2 affinity). PEG-Hb was demonstrated to decrease anaerobic metabolism in animal models of extreme hemodilution (11% Hct) and increase O2 delivery in conditions where native O2 -carrying capacity is severely decreased (Cabrales et al. Citation2010).
These promising early results from studies using HBOCs derived from out-dated human blood or fresh bovine blood suggest that HBOCs may be a viable option when safe blood products are not available. However, there are limitations to the usefulness of these products; because there is often an insufficient supply of outdated human banked blood (and thus human Hb) available and bovine hemoglobin may induce cross-species transmission of pathogens, such as prions. Polymerized porcine hemoglobin (pPolyHb), which is made from readily available porcine blood, potentially overcomes these limitations. pPolyHb has high homology with human Hb, and is free from contamination with human viruses and prions (Zhang et al. Citation2012). Our previous isovolumic exchange transfusion experiments in rats demonstrated that pPolyHb helps maintain hemodynamic stability, enables a suitable level of oxygen delivery to tissues, and effectively reverses anaerobic metabolism caused by extensive blood volume exchange (Zhu et al. Citation2011).
This study was designed to investigate the recovery ability of pPolyHb compared with RBC in a rat model of acute anemia caused by ANH. In particular, we investigate whether infusion of pPolyHb in response to critical normovolemic anemia provides adequate tissue oxygenation, and hence reverses anaerobic metabolism.
Materials and methods
Animals
A total of 32 male Sprague-Dawley rats weighing 240 ± 20 g were obtained from the Xi’an Jiaotong University, China. Animals were kept under standardized temperature conditions (22 ± 1 °C) with free access to food and water. The experiments described in this study were performed in adherence to National Institutes of Health guidelines on the use of experimental animals. The experimental protocol was approved by the Animal Care Committee of Northwest University, Xi’an, China.
Test solutions
pPolyHb (10.5 ± 0.5 g/dL, methemoglobin 5%, endotoxin 1.0 EU/mL, osmolality 300–330 mOsm, pH 7.4 ± 0.05, average molecular weight of pPolyHb 600 ± 50 kD, 64 kD tetramer 2%) was formulated in buffer consisting of Na+ 135–155 mmol/L, K+ 3.0–5.0 mmol/L, Ca2+ 1–3 mmol/L, Cl− 140–160 mmol/L, and stored at 4 °C under nitrogen gas until use.
Surgical procedure
Rats were food-restricted for 12 h. Anesthetic induction was carried out with sodium pentobarbital (45 mg/kg, intramuscularly). The left femoral artery was cannulated (PE 50 tubing) and connected to a MP150 Data Acquisition System (BIOPAC, Goleta, CA) for recording blood pressure, ECG, and heart rate. The right femoral artery was cannulated to induce controlled hemorrhage. Hetastarch (HES) or pPolyHb were administered via the left femoral artery.
Isovolemic hemodilution
After completion of the surgical procedure, a 30 min stabilization period was allowed to elapse to achieve stable baseline conditions. After recording the first data set (baseline), an isovolemic hemodilution protocol was initiated, consisting of three or four cycles of repeated hemorrhage of 20% of estimated total blood volume (70 mL/kg) via the femoral artery, followed by replacement with 6% HES solution via the femoral vein using a bidirectional precision pump. The initial Hb concentration of rats was 14.4 ± 0.8 g/dL. Exchange transfusions were performed at a rate of 0.3 mL/min. The target parameter for the hemodilution protocol was the animal’s Hb concentration. When the Hb concentration reached 4 ± 0.5 g/dL, the data set was collected and animals were randomized to receive a bolus infusion of either pPolyHb (n = 8) or RBC (n = 8). The target trigger for infusion was when the Hb concentration reached more than 7 g/dL. Eight rats that did not receive a bolus infusion of either PolyHb or RBC (i.e., received anesthesia and blood exchange with 6% HES) were used as the control group. In addition, eight rats with no experimental procedure (i.e., anesthesia and intubation only) were used as the sham group for standardization.
Monitoring of hemodynamic parameters
Mean arterial blood pressure (MAP) and heart rate (HR) were monitored every 10 min throughout the experiment. Arterial blood pH, as well as base excess (BE), bicarbonate, and lactate concentrations, was measured on an ABL 800 FLEX (Radiometer, Copenhagen, Denmark). Blood samples were withdrawn before the start of blood exchange (baseline), at the end of hemodilution, at the end of infusion, and 1 h after infusion.
Blood biochemical tests
After 7 d, 4 mL of venous blood was taken from eight rats in each group and centrifuged at 5000 rpm for 5 min on a standard centrifuge to separate the RBC and plasma phases. The plasma phase was frozen (20 °C) for the blood biochemical tests. The plasma activities of a total of eight analytes (total protein [TP], albumin [ALB], albumin/globulin ratio [A/G], aspartate aminotransferase [AST], alanine aminotransferase [ALT], alkaline phosphatase [ALP], creatinine [CREA], and blood urea nitrogen [BUN]) were measured using a fully automated clinical chemistry analyzer (HITACHI7170S).
Histopathologic observations
At 1 d and 7 d, tissue samples of isolated kidney were taken for histological examination. Samples were preserved in 10% formalin and embedded in paraffin, then sectioned into 5-μm intervals and stained with Hematoxylin and Eosin (H&E). A pathologist blinded to the treatment was assigned to assess the histopathological changes in the tissues.
Statistical analysis
All values in the text and figures are presented as mean ± SD. The blood pressure and HR were analyzed by the Student’s t test for two group comparisons. Other data were subjected to one-way ANOVA followed by LSD correction for post hoc tests (SPSS version 13.0 software; SPSS Inc., Chicago, IL). Results were considered statistically significantly different when p values were 0.05.
Results
Blood pressure and heart rate
shows the changes in blood pressure and HR with progressive hemodilution. There were no significant differences in MAP and HR between any of the groups at baseline. In response to hemodilution, MAP declined to 85 ± 5 mmHg in all groups. By the end of infusion, MAP returned to near baseline in the pPolyHb and RBC groups, whereas MAP in the control group remained significantly lower than baseline (). HR followed the same trend (). HR decreased to 420 ± 10 bpm in all groups during hemodilution, while infusion significantly restored HR in the pPolyHb and RBC groups. The control group maintained a significantly lower HR.
Acid–base response and metabolic status
shows the pH, , BE, and lactate (Lac) levels at each phase of the experiment for the three groups. Lactic acidosis developed equally in all groups during the hemodilution phase as evidenced by increased lactate levels and lower levels of BE and
(). No significant changes in pH were observed across groups after hemodilution, infusion, or at the end of the observation period (1 h).
Figure 2. pH, Lactate, , and base excess (BE) values in isovolemic hemodilution model rats. Blood samples were drawn before the start of hemodilution (baseline), at the end of hemodilution, at the end of infusion and after a 1 h observation period (1 h) to assess anaerobic metabolism. Blood gas analysis was performed on an ABL 800 (Radiometer, Copenhagen, Denmark). (A) pH values at different periods in rats in pPolyHb, RBC, and control groups. (B) HCO3− levels at different periods in rats in pPolyHb, RBC, and control groups. (C) Lactate levels at different periods in rats in pPolyHb, RBC, and control groups. (D) BE levels at different periods in rats in pPolyHb, RBC, and control groups.*p < .05 in comparison with baseline; #p < .05 in comparison with the control group; $p < .05 in comparison with the RBC group.
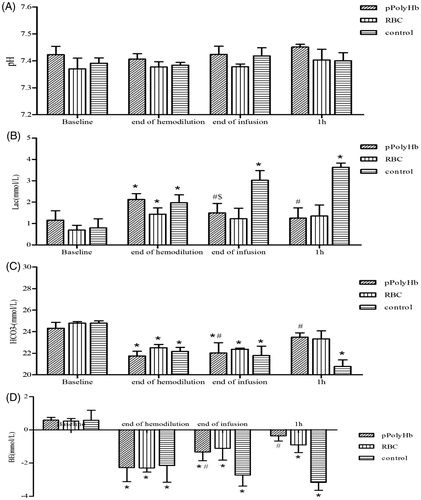
Lactate values increased from 1.16 ± 0.44 to 2.13 ± 0.27 mmol/L in the pPolyHb group and from 0.7 ± 0.22 to 1.44 ± 0.29 mmol/L in the RBC group immediately after hemodilution. These values returned to near baseline, 1.4 ± 0.34, and 1.23 ± 0.49 mmol/L, after infusion with pPolyHb and RBC, respectively. In both experimental groups the lactate levels remained unchanged at the end of the subsequent observation period (). However, in the control group, the lactate value increased from 0.8 ± 0.42 (baseline) to 1.98 ± 0.37 mmol/L after hemodilution, and to 3.63 ± 0.2 mmol/L at the end of the observation period.
In all three groups, the concentration decreased significantly after hemodilution and remained unchanged after infusion (). One hour after the infusion, the
levels in the pPolyHb group (23.49 ± 0.4 mmol/L), and RBC group (23.33 ± 0.75 mmol/L) nearly returned to baseline. For pPolyHb, the value at 1 h was significantly higher than for the control group (20.77 ± 0.62 mmol/L).
After hemodilution, the values for BE fell in all three groups (to −2.28 ± 0.85, −2.3 ± 0.25, and −2.15 ± 1.00 mmol/L for pPolyHb, RBC, and control, respectively) compared with baseline, but remained within the normal range (+3–−3 mmol/L). In the pPolyHb and RBC groups, BE values did not further deteriorate at the end of infusion or after observation for 1 h, and for pPolyHb significantly improved relative to the control group at both data points.
Blood biochemical tests
Rat plasma was obtained 7 d after the experimental procedure. Values for each of the eight plasma analytes that were tested are summarized in . In the pPolyHb and RBC groups, most of the parameters did not show any significant difference versus the sham group. The exceptions were that ALT decreased from 45.67 ± 4.16 (sham) to 35.3 ± 4.04 IU/L in the pPolyHb group, and ALP decreased from 169 ± 34.78 (sham) to 119.67 ± 56.37 IU/L in the RBC group. In the control group, the increase in AST (81.67 ± 11.02 IU/L in control versus 66.33 ± 8.5 IU/L in sham) and the decrease in ALP (105.3 ± 11.5 IU/L in control versus 169 ± 34.78 IU/L in sham) were both significant.
Table 1. Biochemical analysis of rat plasma obtained 7 d after infusion with pPolyHb or RBC.
Histopathologic observations
shows microscopic observations of kidney from rats that underwent isovolemic hemodilution and infusion with pPolyHb or RBC, at time points of 1 d and 7 d after the experimental protocol. Similar to the sham group, the glomerular capillary loops were clearly visible in both the pPolyHb and RBC groups and the structure of the renal tubules appeared normal after 1 d. However, a mild focal lesion in the kidney was evident in the pPolyHb group after 7 d, as manifested by glomerular balloon adhesions. In the RBC group, while there was no evidence of lesions after 1 d, mild inflammatory cell infiltration and glomerular balloon adhesions were seen after 7 d. In the control group, a slight abnormal enlargement of the glomerulus was visible after 1 and 7 d. Moreover, glomerular balloon adhesions, inflammatory cell accumulation, mesangial cell proliferation, and lobular accentuation of glomerulus were also observed in the control group.
Discussion
In this study, we demonstrated that infusion with pPolyHb significantly improved acute anemia caused by isovolemic hemodilution in experimental rats, as evidenced by restored baseline levels of MAP and HR, alleviated metabolic acidosis and reduced activities of markers of liver, and kidney injury. This improvement across all parameters tested was equivalent to that observed with RBC, but greater than that which occurred in a control group without infusion.
There was no statistically significant difference in recovery ability between pPolyHb and RBC. However, as a hemoglobin-based oxygen carrier, pPolyHb has the advantage over RBC that it could be made universally available without the need for blood typing. It is also free from many of the logistical constraints associated with blood product usage and could be readily available in an emergency, such as at disaster sites or on the battlefield (Bucci et al. Citation2009).
The hemodynamic effects of pPolyHb and RBC were along expected lines. With hemodilution, systemic vascular resistance is usually reduced because Hct influences blood viscosity, and viscosity is a component of resistance, and thus hemodilution leads to a decline in MAP. In this study, MAP and HR, which decreased after hemodilution, were promptly restored to near baseline values after infusion of pPolyHb, which is a response similar to that observed in the RBC group. These findings showed that the ability of pPolyHb to expand blood volume and maintain stable hemodynamics was equivalent to RBC.
During hemodilution, as long as normovolemia is maintained, tissue oxygenation is preserved during acute dilutional anemia until a so-called critical hemoglobin concentration (Hbcrit) is reached. When the hemoglobin concentration falls below this critical level, oxygen debt occurs with initiation of anaerobic metabolism, and inefficient cell function (Araújo and Garcia Citation2013). In general, blood oxygen-carrying capacity, determined by the hemoglobin content of blood, is directly related to the ability of the circulation to satisfy the metabolic demand for oxygen of the organism. However, in clinical extreme anemia in normovolemic conditions, or blood losses corrected with volume restoring plasma expanders, the critical factor is not the intrinsic oxygen-carrying capacity of blood but its oxygen-delivery capacity. Infusion of pPolyHb was able to alleviate metabolic acidosis more effectively than the control group without infusion, which can be attributed to the oxygen-carrying and off-loading properties of pPolyHb.
Hemospan (Sangart Inc, San Diego, CA) (MP4) is a polyethylene glycol (PEG)-conjugated human hemoglobin solution in development as an oxygen-carrying plasma expander for use in elective surgery. In addition, a previous study assessing the effect of this product on blood volume, hemodynamics, and metabolic stability showed that hemodilution with this product may also improve outcomes in surgical settings in a model of ANH and intraoperative blood loss (Young et al. Citation2009).
Safe and effective O2-carrying blood substitutes are expected to be at least as efficacious as RBC in maintaining acid-base status, and may cause fewer negative effects on plasma biochemical parameters, and histopathological features. The liver is a vital organ, as it performs a wide range of metabolic activities, including the synthesis and breakdown of both small and complex molecules, many of which are necessary for normal vital functions (Ezejindu et al. Citation2013). ALT and AST are markers of acute liver injury, rather than a reflection of hepatocellular function, because they do not reliably reflect the synthetic ability of the liver and they may come from tissues other than liver (such as muscle). In our study, the decrease in only ALT in the pPolyHb group likely does not reflect hepatic toxicity, since ALT is not a very sensitive index. In addition, ALP is widely distributed in almost every tissue in the body. Low levels are found in serious nephritis, dwarfism, hypothyroidism, and pernicious anemia. Thus, the low level of ALP seen in both the RBC and control groups appears to be consistent with the histological features of the kidney sections in our study that suggested evidence of kidney injury. However, other liver and kidney function markers remained unaltered after 7 d.
The kidneys serve essential regulatory roles in vertebrates. They regulate the balance of electrolytes in the blood, along with maintaining pH homeostasis. They also remove excess organic molecules and waste products of metabolism from the blood. The kidney is susceptible to reductions in oxygen delivery during acute ANH. In a recent dog study, reducing the Hct to 30% resulted in an initial decrease in oxygen delivery to the kidney to 80% of baseline. Subsequent reduction of the Hct to 8–10% (Hbcrit in this model) led to a further decrease in renal oxygen delivery to approximately 25% of baseline (George Citation2015). In our experimental rat model, hemodilution with HES until the Hb concentration reaches 4 ± 0.5 g/dL normally leads to anemia and kidney damage. Infusion with pPolyHb proved to be effective in avoiding kidney damage, as evidenced by the normal histological features of the kidney sections. Gould et al. (Citation1997) have suggested a greater oxygen extraction ratio from polymerized hemoglobin than from RBC. This difference in oxygen extraction at the tissue level could explain why there mild renal injury was seen in the RBC group in the rat anemia model but not in the pPolyHb group. Several factors may contribute to the enhancement of oxygen extraction from polymerized Hb solutions, such as increased plasma hemoglobin concentration, a rightward shift in the P50, and facilitated diffusion due to the lack of stromal components. However, a study in swine (Steven et al. Citation2001) indicates that these effects are only seen at Hct level less than 8%, meaning that when the RBC concentration reaches a critically low level, the extraction ratio from polymerized hemoglobin would exceed that from RBC hemoglobin. Thus, acellular hemoglobin solutions may be most useful in cases of severe, not moderate, anemia.
Conclusion
In conclusion, our results show that pPolyHb infusion does not yield any observable toxic side effects in an acute anemia experimental rat model, and that this blood substitute can carry a sufficient amount of oxygen to the tissues to maintain normal aerobic metabolism. Most biochemical and pathological markers also remained normal, except for the observed mild decrease in ALT. These data suggest that pPolyHb may be a good candidate to move forward into acute anemia clinical trials in the future.
Disclosure statement
The authors report no conflicts of interest. The authors alone are responsible for the content and writing of this article.
References
- Araújo LMTD, Garcia LV. 2013. Acute normovolemic hemodilution: a practical approach. Open J Anesthesiol. 3:38–43.
- Bonegio RG, Fuhro R, Ragno G, Valeri C, Lieberthal W. 2009. A comparison of the acute hemodynamic and delayed effects of 50% exchange transfusion with two different cross-linked hemoglobin based oxygen carrying solutions and pentastarch. Artif Cells Blood Substit Biotechnol. 34:145–157.
- Bucci E, Kwansa H, Koehler RC. 2009. Development of zero-link polymers of hemoglobin, which do not extravasate and do not induce pressure increases upon infusion. Artif Cells Blood Substit Biotechnol. 35:11–18.
- Cabrales P, Meng F, Acharya SA. 2010. Tissue oxidative metabolism after extreme hemodilution with PEG-conjugated hemoglobin. J Appl Physiol. 109:1852–1859.
- Carson JL, Noveck H, Berlin JA, Gould SA. 2002. Mortality and morbidity in patients with very low postoperative Hb levels who decline blood transfusion. Transfusion. 42:812–818.
- Ezejindu DN, Chinweife KC, Ihentuge CJ. 2013. The effects of moringa extract on liver enzymes of carbon tetrachloride induced hepatotoxicity in adult Wister rats. Int J Eng Sci. 2:54–59.
- George JC 2015. Regional tolerance to acute normovolemic hemodilution: evidence that the kidney may be at greatest risk. J Cardiothorac Vasc Anesth. 29:320–327.
- Gould SA, Moore EE, Moore FA, Haenel JB, Burch JM, Sehgal H, et al. 1997. Clinical utility of human polymerized hemoglobin as a blood substitute after acute trauma and urgent surgery. J Trauma. 43:325–332.
- Harrington JP, Kobayashi S, Dorman SC. 2007. Acellular invertebrate hemoglobins as model therapeutic oxygen carriers: unique redox potentials. Artif Cells Blood Substit Immobil Biotechnol. 35:53–67.
- Ickx BE, Rigolet M, Van Der Linden PJ. 2000. Cardiovascular and metabolic response to acute normovolemic anemia. Effects of anesthesia. Anesthesiology. 93:1011–1016.
- Mackenzie CF, Moon-Massat PF, Shander A, Javidroozi M, Greenburg AG. 2010. When blood is not an option: factors affecting survival after the use of a hemoglobin-based oxygen carrier in 54 patients with life-threatening anemia. Anesth Analg. 110:685–693.
- Moore EE, Moore FA, Fabian TC, Bernard AC, Fulda GJ, Hoyt DB, et al. 2009. Human polymerized hemoglobin for the treatment of hemorrhagic shock when blood is unavailable: the USA multicenter trial. J Am Coll Surg. 208:1–13.
- Muir WW, Ilangovan G, Zweier JL, oonmassat PF, Rentko VT. 2011. Vital organ tissue oxygenation after serial normovolemic exchange transfusion with HBOC-201 in anesthetized swine. Shock. 35:597–603.
- Murray D. 2004. Acute normovolemic hemodilution. Eur Spine J. 13:72–75.
- Steven NV, Brian JK, Talarico TL. 2001. Oxygen transport dynamics of acellular hemoglobin solutions in an isovolemic hemodilution model in swine. J Trauma. 51:1153–1160.
- Vallet B. 2010. Venous oxygen saturation as a physiologic transfusion trigger. Crit Care. 14:1–5.
- Walsh TS, Lee RJ, Maciver CR, Garrioch M, Mackirdy F, Binning AR, Cole S, McClelland DB. 2006. Anemia during and at discharge from intensive care: the impact of restrictive blood transfusion practice. Intensive Care Med. 32:100–109.
- Wu W, Rathore S, Wang Y, Radford M, Krumholz H. 2001. Blood transfusion in elderly patients with acute myocardial infarction. N Engl J Med. 345:1230–1236.
- Young MA, Lohman J, Malavalli A. 2009. Hemospan improves outcome in a model of perioperative hemodilution and blood loss in the rat: comparison with hydroxyethyl starch. J Cardiothorac Vasc Anesth. 23:339–347.
- Zhang W, Yan KP, Dai PG, Tian JJ, Zhu HL, Chen C. 2012. A novel hemoglobin-based oxygen carrier, polymerized porcine hemoglobin, inhibits H2O2-induced cytotoxicity of endothelial cells. Artif Organs. 36:151–160.
- Zhu HL, Dang XD, Yan KP, Dai PG, Ma J, Li Y, Chang TMS, Chen C. 2011. Pharmacodynamic study of polymerized porcine hemoglobin (pPolyHb) in a rat model of exchange transfusion. Artif Cells Blood Substit Immobil Biotechnol. 39:119–126.