Abstract
Poor water solubility of hydrophobic drugs is one of the major problems in pharmaceutical sciences. Herein, in order to address the poor solubility of crocetin, the 30% of primary amines of PAMAM G4 and PPI G4 were alkylated and evaluated as drug delivery vectors. According to the results, this modification could improve the drug delivery efficiency in term of drug solubility, release pattern and cytotoxicity; however, the encapsulation yield was decreased. Accordingly, it can be concluded that the designed alkylated dendrimers are able to improve the drug delivery efficiency, may be through improving the solubility and cellular uptake of crocetin.
Introduction
Crocetin is an amphiphilic low molecular weight carotenoid found in stigmas of the saffron crocus (Crocus starus L.). Crocetin molecule consists of a C-20 carbon chain with altering double bonds, and a carboxylic acid group at each terminal parts of the molecule. Naturally, crocetin is believed to be synthesized through enzymatic cleavage of zeaxanthin followed by glycosylation to form crocins with different pharmacokinetic properties () (Gutheil et al. Citation2012, Kim et al. Citation2014, Zhang et al. Citation2009).
It was shown that crocetin possess various biological activities such as anti-inflammation, anti-oxidation, anti-VEGF-induced angiogenesis, anti-cancer, prevention of NMDA-induced retinal damage, hepatoprotection, and neuroprotection (Ahmad et al. Citation2005, Magesh et al. Citation2009, Nam et al. Citation2010, Ohno et al. Citation2012, Tseng et al. Citation1995, Umigai et al. Citation2012, Wang et al. Citation1991).
In spite of such beneficial activities of crocetin, its use is limited due to the poor water solubility in its free-acid form. In addition, crocetin in this form is insoluble in most organic solvents, but soluble in pyridine and dimethylsulfoxide (log p = 4.312 and pKa = 4.39) (Bathaie et al. Citation2014). Such low water solubility may result in some difficulties in formulation as well as the suboptimal performance and poor bioavailability. Hence, recent studies have been focused on different approaches to increase the solubility of such compounds. Using nanocarriers such as PLGA and dendrimers offer a promising strategy to improve the formulation and solubility of water-insoluble substances (Cai et al. Citation2010, Hafezi et al. Citation2016).
Dendrimers are novel tree-like, well-defined and three-dimensional hyperbranched macromolecules that are used as drug and gene delivery carriers. They can encapsulate different compounds through their amphiphillic nature, namely hydrophobic interior and hydrophilic exterior which allows their application as solubility enhancers and sustained release drug carrier. Dendrimers can improve the solubility of drugs possibly through hydrophobic, hydrogen bonds and/or electrostatic interactions. Several studies have reported the efficiency of dendrimers in solubility enhancement of various drugs and poor soluble compounds (Beezer et al. Citation2003, Chauhan Citation2015, Wang et al. Citation2013). Furthermore, dendrimers can be engineered and conjugated with different functional motifs such as targeting molecules (Majoros et al. Citation2006).
Poly (amidoamine) dendrimers (PAMAM) and Poly (propylene) imine dendrimers (PPI) have been the most investigated families of dendrimers as solubility enhancers (Baars et al. Citation2000, Jain et al. Citation2015, Murugan et al. Citation2013, Sideratou et al. Citation2001). So far, several modifications such as PEGylation and acetylation have been adopted to improve the drug delivery efficiency and reduce the cytotoxicity of these vectors. Such modifications may alter the host–guest interactions, and thereby increase the solubility. In our previous studies, it was shown that alkylation of PAMAM and PPI could reduce their cytotoxicity and enhance gene delivery efficiency (Ayatollahi et al. Citation2015, Hashemi et al. Citation2013, Citation2015). Accordingly, the aim of this study was to evaluate the performance of alkyl-modified PPI G4 and PAMAM G4 derivatives as enhancers of solubility and antitumor activity of crocetin. It can be expected that alkylation might improve the solubility of crocetin and change the drug release rate, resulting in a sustained release drug delivery system with low cytotoxicity.
Experimental
Chemicals and reagents
G4 PPI-dendrimer (Mw: 3513.9) or DAB-Am-32 (32 primary amine terminal groups) was obtained from Symo-Chem BV (Eindhoven, the Netherlands). G4 PAMAM-dendrimer (Mw: 14214.17) with ethylenediamine core and 64 primary amines on the surface (10 wt. % in methanol), 10-bromodecanoic acid and 3-(4, 5-dimethylthiazol-2-yl)-2, 5-diphenyl tetra zolium bromide (MTT) were purchased from Sigma-Aldrich (Schnelldor, Germany). Fetal bovine serum (FBS) and Dulbecco’s modified Eagle’s medium (DMEM) were obtained from Gibco (Darmstadt, Germany). Dialyses were carried out by using Spectra/Por dialysis membranes (Spectrum Laboratories, Houston, TX). Crocetin was extracted from Crocus sativus L. based on the method represented in the Iran Patent No. 84459.
Conjugation of 10-bromodecanoic acid to G4-PAMAM or G4-PPI
Conjugation of 10-bromodecanoate to 30% surface primary amines of either PAMAM G4 or PPI G4 was reported in our previous studies (Ayatollahi et al. Citation2015, Hashemi et al. Citation2013). Briefly, 10-bromodecanoic acid was dissolved in chloroform and added drop wise to a vigorously stirred solution of either PAMAM or PPI in chloroform. The reactions were allowed to proceed at room temperature for 24 h. The solvent was evaporated under reduced pressure and the residue was dissolved in distilled water. In order to remove any unreacted alkylcarboxylates and reagents, the resulting solutions were dialyzed (10 kDa and 2 kDa cut-off dialysis membranes for PAMAM and PPI derivatives, respectively) against distilled water for three days and then lyophilized for 24 h.
Determination of the primary amine content of PAMAM derivatives with 2, 4, 6-trinitrobenzenesulfonic acid (TNBS)
The degree of alkylcarboxylate grafting of PAMAM and PPI derivatives was determined by TNBS method as described previously (Ayatollahi et al. Citation2015, Hashemi et al. Citation2013). Briefly, freshly prepared aqueous TNBS solution (15 mg/mL) was simultaneously added to 96-well plate containing different concentration of either PAMAM or PPI derivatives diluted in borate buffer (0.1 M, pH 9.2). The UV absorbance of the samples was recorded by an ELISA plate reader at 410 nm and the percentage of grafting in the modified dendrimers was calculated.
Encapsulation of crocetin within modified and unmodified dendrimers
One milligram of crocetin was added into various amounts of dendrimer solutions in water to obtain crocetin:dendrimer ratios of 1:2, 1:4 and 1:8 (weight to weight). The crocetin/dendrimer mixtures were vigorously stirred for 24 h and then centrifuged (10,000 rpm for 5 min) to remove the precipitates related to non-complexed free crocetin, which is insoluble in water. In order to measure the encapsulation efficiency, a defined amount of lyophilized product was dissolved in DMSO and analyzed by UV-Vis spectrophotometer at 430 nm using the calibration curve of 0.25–10 μg/mL crocetin in the same solvent.
The encapsulation yield was calculated according to the following equation:
In vitro release of crocetin from alkylated dendrimers
About 500 μL of PBS 0.2 M (pH 7.4) was added to 500 μL solution of crocetin/dendrimers complex (containing 0.5 mg crocetin) in distilled water. The resulting solution was equally divided in different tubes (100 μL) and kept in a shaker incubator set at 90 rpm and 37 °C. These tubes were taken out at time intervals of 1, 2, 3, 4, 24 and 48 h, and centrifuged (10,000 rpm for 5 min). The insoluble part was dissolved in 500 μL DMSO and the absorbance was measured by UV-Vis spectrophotometer at 430 nm (Mukerjee and Vishwanatha Citation2009).
The percentage of release was calculated according to the following equation:
In order to measure the quantities of released crocetin, the calibration curve of different concentrations of crocetin vs. absorbance at 430 nm was prepared.
Biology assays
Cell culture and cytotoxicity assay
To assess the cytotoxic effects, MTT assay was conducted, which was based on the conversion of MTT dye to formazan by mitochondrial dehydrogenase in live cells (Mosmann Citation1983). In brief, MCF-7 cells were seeded in 96-well plates (1 × 104 cells/well) and incubated at 37 °C in 5% CO2 for 24 h.
After that, the cells were treated with 100 μL of different concentrations of crocetin encapsulated in either alkylated or unmodified dendrimers or equivalent amount of dendrimers in complexes. Then the cells were incubated for another 24 h. The medium was removed and 20 μL MTT solution (5 mg/ml) was added into each well, and the cells were incubated at 37 °C for 3 h. The medium was removed and insoluble formazan product was dissolved in DMSO. The absorbance was measured at 570 nm and 630 nm in an ELISA reader. The percentage of cell viability for each concentration was calculated.
Analysis of apoptotic cells by flow cytometry
MCF-7 cells were cultured in 48-well microplates for 24 h and then exposed to different ratios of crocetin/dendrimers and unmodified dendrimers prepared in RPMI 1640 and incubated for another 24 h at 37 °C in 5% CO2. After treatment, the cells were harvested, washed with PBS, centrifuged (5000 rpm for 15 minutes at 25 °C) and then 100 μL of propidium iodide (50 μg/ml PI in 0.1% sodium citrate, 0.1% Triton X-100, and 100 μg/ml RNase A) was added to the sedimented cells and incubated at 4 °C for 2 h. After that, the cells were centrifuged, and 1 mL cold PBS was added to the cell pellets. The Sub G1 peak (apoptotic cells) was determined using a flow cytometer (Paratec, Wallerfangen, Germany).
Statistical analyses
The data are presented as the mean ± SD value. Statistical significance was calculated using the t test for paired samples p < .05 was regarded as significant.
Results
Synthesis of modified PAMAMs and PPIs
In order to increase the lipophilicity of the vectors, the primary amines of PAMAM G4 and PPI G4 were alkylated by 10-bromodecanoic acid. The degree of substitution was determined by TNBS assay. Based on the results, the grafting percentage was 27% and 21% for PAMAM G4 and PPI G4, respectively.
Encapsulation of crocetin within modified and unmodified PAMAMs and PPIs
The encapsulation efficiency of modified vectors was determined by UV-Vis spectrophotometer at 430 nm, and the data are presented in . According to the data, the alkylation decreased the encapsulation yield in both PPI G4 and PAMAM G4 groups. The crocetin/dendrimers ratios having highest encapsulation yield were used in ensuing experiments.
Table 1. The encapsulation yield (%) of crocetin into PPI G4, PAMAM G4 and corresponding alkylated dendrimers.
In vitro release of crocetin from alkylated dendrimers
The in vitro release behavior of crocetin from the dendrimers was investigated using UV-Vis spectrophotometer at 430 nm. According to , after 48 h ∼20% of crocetin was found in outer phase of both PAMAM G4–10 Br-30% and PPI G4–10 Br-30% complexes; however, during the same time ∼60% and 70% of crocetin was released from PAMAM G4 and PPI G4, respectively. Moreover, the unmodified PPI G4 exhibited very rapid release of crocetin during the first 4 h (∼50%), while this phenomenon was not observed in PAMAM G4.
Cytotoxicity assay
MTT assay was conducted to evaluate the cytotoxicity of the designed structures at various concentrations of crocetin and equivalent amounts of polymers in each treatment to verify the origin of cytotoxicity in the experiment (. According to the results, the encapsulation of crocetin into PPI G4 dendrimer resulted in a complex that has almost similar cytotoxicity in comparison with empty PPI G4 (). However, such a complexation with alkylated PPI G4 enhanced the cytotoxicity of crocetin with 6.25 μM concentration while polymer alone showed lower cytotoxicity (). On the other hand, in PAMAM G4 groups, the complexed PAMAM G4 at concentrations of crocetin ≥12.5 μM exhibited lower toxicity compared to un-complexed PAMAM (). Surprisingly, after the encapsulation of various concentrations of crocetin (≥1.6 μM) into the alkylated PAMAM G4, the cytotoxicity was significantly increased (). The formulation using alkylated PAMAM could alter the IC50 of crocetin (after 24 h) from 598.65 to 2.176 μM. Concerning the PPI G4 groups, the alkylated PPI G4 could reduce the IC50 of crocetin from 598.51 to 5.947 μM (.
Figure 3. % Cell viability of MCF-7 cells treated with different concentrations of crocetin encapsulated in either alkylated or unmodified dendrimers (mean ± SD, n = 3). The tables below the graphs represent the concentrations of crocetin as well as equivalent amount of polymers used in the complexes.
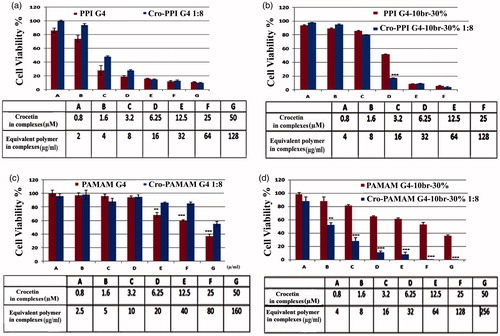
Analysis of apoptotic cells profile by flow cytometry
The percentage of apoptotic cells was analyzed using flow cytometry at concentrations of 0.8, 1. 6, 3.12, and 6.25 μM of crocetin encapsulated in either unmodified dendrimer or alkylated dendrimer 1:8 (. According to the data, the encapsulation of crocetin (≥1.6 μM) within either types of alkylated dendrimers (PPI G4–10br-30% and PAMAM G4–10br-30%) induced a sub-G1 peak and significantly enhanced the percentage of apoptotic cells. Overall, the percentage of sub G1 cells in complexed alkylated PAMAM dendrimers (20–70%) were higher than corresponding PPIs (18–42%), which can be related to the higher water solubility of crocetin entrapped in PAMAMs than in the PPI polymers.
Discussion
Poor water solubility of some drugs is one of the major issues in pharmaceutical applications of these compounds. Numerous attempts have been made to increase the solubility of hydrophobic drugs. One of these strategies is incorporation of these drugs into various nano-vectors such as liposomes and polymers. It was shown that nanocarriers have several advantages such as largely enhancing the concentration of crocetin in blood, stability under the physiological condition and controlled drug delivery at or around the cancer tissues (Zhao et al. Citation2011, Citation2012). Dendrimers as nano-based drug carriers have shown great potential in drug solubilization controlled release, and thereby enhancing the bioavailability of hydrophobic drugs. (Chauhan et al. Citation2004, Gu et al. Citation2013, Zhao et al. Citation2011, Zhou et al. Citation2013).
PAMAM and PPI are the two most investigated dendrimers as drug delivery vectors. The hydrodynamic diameter and internal cavity of PAMAM differ from those of PPI. In comparison with PAMAM, PPI dendrimers are smaller, more stable in higher temperature and less polar in their interior environment. Based on the structure of dendrimers, they have a high density of active groups including –NH2, –COOH, and –OH at their outer shell. Therefore, they are highly reactive molecules, which can be modified or conjugated with various molecules. A wide range of modifications such as PEGylation, acetylation, hydroxylation and methylation have been investigated to improve the drug/gene delivery efficiency as well as reducing the cytotoxicity (Murugan et al. Citation2013, Oskuee et al. Citation2009, Takahashi et al. Citation2007).
In our previous studies, different chain lengths of bromoalkylcarboxylates were conjugated with different percentage of primary amines of PAMAMs and PPIs. The results showed that 30% substitution of primary amines by 10-bromodecanoic group resulted in higher gene transfection efficiency and lower cytotoxicity compared to other formulations (Ayatollahi et al. Citation2015, Hashemi et al. Citation2013, Citation2015). In the current study, we modified the surface layer of PAMAM G4 and PPI G4 by 30% surface primary amines grafting with 10-bromodecanoic acid and explored their potential as solubility enhancers and drug delivery carriers. We used PPI (G4 and G5) and PAMAM (G3 and G4) for encapsulation of crocetin. Although, the encapsulation yield (%) of crocetin into PPI G4 and G5 was almost similar, we chose PPI G4 for our study due to its lower cytotoxicity. In regard to the PAMAM, since the encapsulation yield of PAMAM G3 was lower than PAMAM G4, this generation of PAMAM (G4) was considered for other modifications (data not shown).
According to the results, the alkylated vectors showed lower encapsulation yield at the same croectin/vectors ratio than the corresponding unmodified dendrimers. These dendrimers may improve the solubility through different mechanisms such as electrostatic interaction between surface amines and negative groups of guest molecules, hydrophobic and open cavities as well as hydrogen bond formations (Jain and Gupta Citation2008). Based on this, it was assumed that alkylation reduced the positive surface charge of polymers, and thereby the interaction between crocetin having negative charge with polymers on their surface might be decreased. Another assumption might be related to the steric hindrance of alkyl moieties, which prevents the efficient interaction of crocetin with surface amines.
depicts in vitro release of crocetin from both modified and unmodified dendrimers. Both alkylated vectors demonstrated a sustained release pattern of croectin within the time period, while during the same time PAMAM G4 and PPI G4 released higher percentage of encapsulated molecule. The reason may be because of more crocetin encapsulation into hydrophobic interior rather than surface of alkylated dendrimers compared to unmodified structure. Based on the results, crocetin loaded in PPI G4 displayed a burst release for the first 4 h and after that the release was almost linear. However, the PAMAM did not show such a rapid release. Such behavior might be explained by the larger interior cavity of PAMAM, which results in higher drug loading in comparison with its PPI counterpart.
In an in vivo condition, released crocetin has a weak interaction with serum albumin and is very well distributed to tissues of the body, so that it seems that it is unlikely to precipitate in plasma. On the other hand, the lipophilicity of crocetin facilitates its uptake from cell membranes. Moreover, inside the cell, like other insoluble drugs, the environmental conditions of cytoplasm, prevents the precipitation of crocetin (Kanakis et al. Citation2007).
In cytotoxicity assay, it is important that the observed toxicity is due to the crocetin complexed with dendrimers not carriers. The formulation composed of crocetin and alkylated PAMAM demonstrated significantly higher anti-proliferative activity than empty alkylated PAMAM, while complexation of ≥12.5 μM of crocetin with PAMAM G4 (≥ 40 μg/mL) even decreased the viability of the cells compared to unmodified PAMAM G4. Modification of PPI also resulted in a carrier which could increase the cytotoxicity of crocetin at a concentration of 6.25 μM encapsulated in 32 μg/mL polymer; however, there were no significant differences between viability of cells treated with unmodified PPI, either complexed or un-complexed. These findings are in agreement with our previous studies showing that alkylation of PAMAM and PPI could improve cytotoxicity especially in higher concentrations of dendrimer (Ayatollahi et al. Citation2015, Hashemi et al. Citation2015).
Based on our findings, the IC50 of crocetin after 24 h was reduced from 598 to 2.176 μM and 5.947 μM after complexation with PAMAM G4–10-Br 30% and PPI G4–10Br 30%, respectively. The free crocetin at the tested concentrations (0.8–6.25 μM) in flow cytometry experiment did not exhibit notable cytotoxicity effects. Accordingly, the reason of enhancement of crocetin cytotoxicity in the complexes may be due to the higher cellular uptake because of the interaction of hydrophobic moieties with membrane phospholipids, which enhances internalization of complexes. These cytotoxicity findings were further supported by flow cytometry data in which the encapsulation of crocetin into the alkylated dendrimers induced a sub-G1 peak and enhanced the percentage of apoptotic cells in a concentration-dependent manner. To sum up, the percentage of sub G1 cells in complexed alkylated PAMAM dendrimers (20–70%) were higher than the corresponding PPIs (18–42%) which can be related to the higher water solubility of crocetin entrapped in PAMAMs than in the PPI polymers.
The higher lethality of crocetin, encapsulated into alkylated dendrimers, on cancer cells may be due to better solubilization of crocetin, better passive targeting and/or better interaction with cell membranes, and thereby higher cellular uptake than free crocetin.
Conclusion
In summary, generation 4 of PAMAM and PPI, after 30% alkylation were used to encapsulate a model poor-water soluble compound, crocetin, to increase its solubility and cytotoxicity. Although the resulting formulations showed lower encapsulation yield, but they offered a sustained release drug delivery system.
The most obvious finding to emerge from this study is that it is possible to enhance the bioavailability of hydrophobic drugs by encapsulation of such poor water-soluble compounds into the engineered dendrimer with low cytotoxicity. In conclusion, the findings of this study suggest that these nano-vectors may be useful for efficient delivery of hydrophobic drugs; however, further investigations are needed to shed light on the usage of such vectors as solubility enhancers.
Disclosure statement
Authors report no competing financial interest.
Funding
This research project was funded by Mashhad University of Medical Sciences (Grant No. 920848), Mashhad, Iran.
References
- Ahmad AS, Ansari MA, Ahmad M, Saleem S, Yousuf S, Hoda MN, Islam F. 2005. Neuroprotection by crocetin in a hemi-Parkinsonian rat model. Pharmacol Biochem Behav. 81:805–813.
- Ayatollahi S, Hashemi M, Kazemi Oskuee R, Salmasi Z, Mokhtarzadeh A, Alibolandi M, Abnous K, Ramezani M. 2015. Synthesis of efficient gene delivery systems by grafting PEGylated alkylcarboxylate chains to PAMAM dendrimers: evaluation of transfection efficiency and cytotoxicity in cancerous and mesenchymal stem cells. J Biomater Appl. 30:632–648.
- Baars MW, Kleppinger R, Koch MH, Yeu SL, Meijer EW. 2000. The localization of guests in water-soluble oligoethyleneoxy-modified poly(propylene imine) dendrimers. Angew Chem Int Ed Engl. 39:1285–1288.
- Bathaie SZ, Farajzade A, Hoshyar R. 2014. A review of the chemistry and uses of crocins and crocetin, the carotenoid natural dyes in saffron, with particular emphasis on applications as colorants including their use as biological stains. Biotech Histochem. 89:401–411.
- Beezer AE, King ASH, Martin IK, Mitchel JC, Twyman LJ, Wain CF. 2003. Dendrimers as potential drug carriers; encapsulation of acidic hydrophobes within water soluble PAMAM derivatives. Tetrahedron. 59:3873–3880.
- Cai Z, Wang Y, Zhu LJ, Liu ZQ. 2010. Nanocarriers: a general strategy for enhancement of oral bioavailability of poorly absorbed or pre-systemically metabolized drugs. Curr Drug Metab. 11:197–207.
- Chauhan AS. 2015. Dendrimer nanotechnology for enhanced formulation and controlled delivery of resveratrol. Ann NY Acad Sci. 1348:134–140.
- Chauhan AS, Jain NK, Diwan PV, Khopade AJ. 2004. Solubility enhancement of indomethacin with poly(amidoamine) dendrimers and targeting to inflammatory regions of arthritic rats. J Drug Target. 12:575–583.
- Gu L, Wu Z, Qi X, He H, Ma X, Chou X, et al. 2013. Polyamidomine dendrimers: an excellent drug carrier for improving the solubility and bioavailability of puerarin. Pharm Dev Technol. 18:1051–1057.
- Gutheil WG, Reed G, Ray A, Anant S, Dhar A. 2012. Crocetin: an agent derived from saffron for prevention and therapy for cancer. Curr Pharm Biotechnol. 13:173–179.
- Hafezi GZ, Alebooye LF, Mokhtarzadeh A, Ramezani M, Hashemi M. 2016. Evaluation of anti-cancer activity of PLGA nanoparticles containing crocetin. Artif Cells Nanomed Biotechnol. 21:1–6.
- Hashemi M, Parhiz H, Mokhtarzadeh A, Tabatabai SM, Farzad SA, Shirvan HR, Ramezani M. 2015. Preparation of effective and safe gene carriers by grafting alkyl chains to generation 5 polypropyleneimine. AAPS PharmSciTech. 16:1002–1012.
- Hashemi M, Sahraie Fard H, Amel Farzad S, Parhiz H, Ramezani M. 2013. Gene Transfer Enhancement By Alkylcarboxylation of Poly(Propylenimine). Nanomed J. 1:55–62.
- Jain NK, Gupta U. 2008. Application of dendrimer–drug complexation in the enhancement of drug solubility and bioavailability. Expert Opin Drug Metab Toxicol. 4:1035–1052.
- Jain S, Kesharwani P, Tekade RK, Jain NK. 2015. One platform comparison of solubilization potential of dendrimer with some solubilizing agents. Drug Dev Ind Pharm. 41:722–727.
- Kanakis CD, Tarantilis PA, Tajmir-Riahi HA, Polissiou MG. 2007. Crocetin, dimethylcrocetin, and safranal bind human serum albumin: stability and antioxidative properties. J Agric Food Chem. 55:970–977.
- Kim SH, Lee JM, Kim SC, Park CB, Lee PC. 2014. Proposed cytotoxic mechanisms of the saffron carotenoids crocin and crocetin on cancer cell lines. Biochem Cell Biol. 92:105–111.
- Magesh V, DurgaBhavani K, Senthilnathan P, Rajendran P, Sakthisekaran D. 2009. In vivo protective effect of crocetin on benzo(a)pyrene-induced lung cancer in Swiss albino mice. Phytother Res. 23:533–539.
- Majoros IJ, Myc A, Thomas T, Mehta CB, Baker JR. Jr. 2006. PAMAM dendrimer-based multifunctional conjugate for cancer therapy: synthesis, characterization, and functionality. Biomacromolecules. 7:572–579.
- Mosmann T. 1983. Rapid colorimetric assay for cellular growth and survival: application to proliferation and cytotoxicity assays. J Immunol Methods. 65:55–63.
- Mukerjee A, Vishwanatha JK. 2009. Formulation, characterization and evaluation of curcumin-loaded PLGA nanospheres for cancer therapy. Anticancer Res. 29:3867–3875.
- Murugan E, Geetha Rani DP, Srinivasan K, Muthumary J. 2013. New surface hydroxylated and internally quaternised poly(propylene imine) dendrimers as efficient biocompatible drug carriers of norfloxacin. Expert Opin Drug Deliv. 10:1319–1334.
- Nam KN, Park YM, Jung HJ, Lee JY, Min BD, Park SU, et al. 2010. Anti-inflammatory effects of crocin and crocetin in rat brain microglial cells. Eur J Pharmacol. 648:110–116.
- Ohno Y, Nakanishi T, Umigai N, Tsuruma K, Shimazawa M, Hara H. 2012. Oral administration of crocetin prevents inner retinal damage induced by N-methyl-D-aspartate in mice. Eur J Pharmacol. 690:84–89.
- Oskuee RK, Dehshahri A, Shier WT, Ramezani M. 2009. Alkylcarboxylate grafting to polyethylenimine: a simple approach to producing a DNA nanocarrier with low toxicity. J Gene Med. 11:921–932.
- Sideratou Z, Tsiourvas D, Paleos CM. 2001. Solubilization and release properties of PEGylated diaminobutane poly(propylene imine) dendrimers. J Colloid Interface Sci. 242:272–276.
- Takahashi T, Hirose J, Kojima C, Harada A, Kono K. 2007. Synthesis of poly(amidoamine) dendron-bearing lipids with poly(ethylene glycol) grafts and their use for stabilization of nonviral gene vectors. Bioconjug Chem. 18:1163–1169.
- Tseng TH, Chu CY, Huang JM, Shiow SJ, Wang CJ. 1995. Crocetin protects against oxidative damage in rat primary hepatocytes. Cancer Lett. 97:61–67.
- Umigai N, Tanaka J, Tsuruma K, Shimazawa M, Hara H. 2012. Crocetin, a carotenoid derivative, inhibits VEGF-induced angiogenesis via suppression of p38 phosphorylation. Curr Neurovasc Res. 9:102–109.
- Wang CJ, Shiow SJ, Lin JK. 1991. Effects of crocetin on the hepatotoxicity and hepatic DNA binding of aflatoxin B1 in rats. Carcinogenesis. 12:459–462.
- Wang L, Xu X, Zhang Y, Zhang Y, Zhu Y, Shi J, Sun Y, Huang Q. 2013. Encapsulation of curcumin within poly(amidoamine) dendrimers for delivery to cancer cells. J Mater Sci Mater Med. 24:2137–2144.
- Zhang R, Qian Z-Y, Han X-Y, Chen Z, Yan J-L, Hamid A. 2009. Comparison of the effects of crocetin and crocin on myocardial injury in rats. Chin J Nat Med. 7:223–227.
- Zhao C, Wang Y, Su Y, Zhang H, Ding L, Yan X, et al. 2011. Inclusion complexes of isoflavones with two commercially available dendrimers: solubility, stability, structures, release behaviors, cytotoxicity, and anti-oxidant activities. Int J Pharm. 421:301–309.
- Zhao L, Ye Y, Li J, Wei YM. 2011. Preparation and the in-vivo evaluation of paclitaxel liposomes for lung targeting delivery in dogs. J Pharm Pharmacol. 63:80–86.
- Zhao BX, Zhao Y, Huang Y, Luo LM, Song P, Wang X, et al. 2012. The efficiency of tumor-specific pH-responsive peptide-modified polymeric micelles containing paclitaxel. Biomaterials. 33:2508–2520.
- Zhou Z, D'Emanuele A, Attwood D. 2013. Solubility enhancement of paclitaxel using a linear-dendritic block copolymer. Int J Pharm. 452:173–179.