Abstract
Recent evidence suggests that mesenchymal stem cells (MSCs) have promising therapeutic potential for a broad range of diseases. Because the percentage of MSCs obtained from tissues is very low for cell therapy applications, ex vivo expansion of MSCs is necessary, but aging, loss of stemness and undesired differentiation of them during in vitro cultivation reduces their effectiveness. For achieving ideal therapeutic potential of MSCs in tissue regenerative purposes, it is necessary to retain their stemness properties in vitro. This review emphasis on the last updates in preserving the self-renewal capability of stem cells through in vitro expansion with different parameters.
Introduction
Stem cells have two significant properties: self-renewal and multipotential differentiation ability that define their stemness. They are essential factors in both developmental biology and regenerative medicine (Potten and Loeffler Citation1990). In the latest decade, an enhancing attention in stem cells researches and their medical applications has become obvious. The most commonly studied stem cells are embryonic stem cells (ESCs), induced pluripotent stem cells (iPSCs), bone marrow mesenchymal stem cells (BMSCs), and adipose tissue-derived stem cells (ASCs). ESCs, derived from the early-stage fetus, are one of the most broadly studied cell origins for regenerative medicine (Schwartz et al. Citation2012, Zare et al. Citation2015, Zare et al. Citation2016). ESCs show the capacity to differentiate in to a diversity of particular cell kinds and therefore show a distinctive chance for tissue engineering and regenerative medicine, but the ethical issues and tumorigenic potential of them are two limitations of their use in regenerative medicine. In comparison, MSCs are moderately harmless and have been isolated from a diversity of tissues, e.g. bone marrow, adipose tissue, hair follicles, dermis, heart, liver, and spleen. MSCs have become one of the most widespread stem cell residents in stem cell-based regeneration research, from adipose tissue can be reaped in higher amounts with fewer aggressive approaches (Kolli et al. Citation2010, Orford and Scadden Citation2008); major reductions in the proliferation and differentiation ability of murine and hBMSCs were detected through in vitro expansion; the expression of stemness biomarkers in hMSCs diminished meaningfully through long-term handling, together with the reduction of differentiation capacity (Bonab et al. Citation2006, Kretlow et al. Citation2008) .So, the key question is in what way to preserve the stemness of stem cells. As MSCs are defined by their capability to self-renew and produce cells with multi-lineage potential, identification of the in vivo and in vitro controlling factors that maintain their stemness properties is necessary to recognize MSCs biology in vivo and also for producing enough amounts of MSCs in laboratories for therapeutic purposes. MSCs gene and protein expression profiles vary between the undifferentiated stem and differentiated states (Tormin et al. Citation2009). In this review, we focus on factors which affect stemness of MSCs and categorize them into 4 groups include, intrinsic parameters, extrinsic parameters, both intrinsic and extrinsic parameters, and donor-related parameters (, ) (Leychkis et al. Citation2009).
Figure 1. Effective parameters on the stemness of MSCs: in general parameters that influence the stemness of MSCs categorize in 4 groups, intrinsic parameters, extrinsic parameters, parameter in both groups and donor-related parameters.
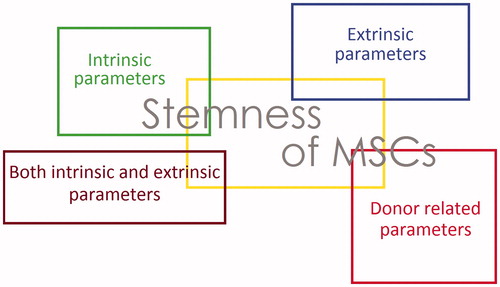
Table 1. Parameters affecting the stemness of MSCs.
Intrinsic parameters
Genetic regulation
Nuclear receptors (NRs)
Genetic regulation containing diverse transcription factors results in the self-renewal and differentiation properties of stem cells. The NR superfamily consist of 48 ligand-activated transcription factors involved in various physiological functions for example metabolism, development, and reproduction. Evidence displays that special NRs function in controlling stemness or differentiation of ESCs and ASCs. Studies provide visions into the therapeutic ability of the NR superfamily in stem cell therapy and in treating stem cell-related disease (e. g., cancer stem cell) (Monaghan et al. Citation1995). NRs are not only included in the pathway of tissue-specific stem cell control but specific NRs also play critical roles in preserving ESCs in collaboration with Oct3/4 and Nanog. A complete overview of the NR superfamily in stem cell biology is missing. Studies focused on the roles of NRs that are included in ASCs and ESCs biology. NRs have special influence on stem cells. For example, some NRs controlling pluripotency of ESCs like octamer-binding transcription factor 4 (oct4), sox2, nanog, c-myc, Lin 28. GCNF and RARs are included in prompting ESCs differentiations. DAX-1, ERRβ, and LRH-1 preserve stemness of ESCs by prompting Oct3/4 expression. Some NRs like RARγ, AR are included in lineage-specification of ASCs. Subsets of NRs are involved in regulating MSCs differentiation into various physiological lineage of cell kinds (Jeong and Mangelsdorf Citation2009). Practical studies have demonstrated a set of pluripotency transcription factors that influence the pluripotent ability. Inside this collection, the transcription factors Sox2, Nanog, and Oct4 or Pou5f are essential for the effective conservation of pluripotent cell identity. These factors control stem cell pluripotency and differentiation via co-localization and the collaboration with each other. Pluripotency transcription factors are limited to pluripotent cells and are reduced during differentiation (Kashyap et al. Citation2009).
The action of Oct4 in ESCs is crucial for the self-renewal and differentiation capacities of mammalian ESCs. The exact expression patterns and functions of oct4 in ASCs are completely debatable. In summary, these reports propose that OCT4A gene acts functionally preserved roles in human umbilical cord blood-derived multipotent stem cells (hUCB-MSCs), making its expression biologically essential for ex vivo culture of hUCB-MSCs (Lengner et al. Citation2008).
Sox2, as a transcriptional factor, is essential for preserving self-renewal, or pluripotency of undifferentiated ESCs. Sox2 has an important role in preservation of embryonic and neural stem cells (Rizzino Citation2009).
Nanog is a transcription factor that involved with self-renewal of undifferentiated ESCs. In humans, this protein is encoded by the NANOG gene. Nanog in connection with other factors such as POU5F1 (Oct-4) and sox2 form ESCs identity (Mitsui et al. Citation2003). Therefore, any extrinsic factor which affects the stemness marker genes expression is important in preserving stemness of stem cells.
Nuclear receptor subfamily 2, group F, member 2 (NR2F2), is a nuclear receptor that has been recognized in the mesenchymal section of developing organs. Reports have displayed that the inhibition of NR2F2 by shRNA-mediated knockdown, reduced self-renewal ability, and pluripotency of BM-MSCs. Also, the suppression of colony-forming capacity may be ascribed to the increase of senescence rate via up-regulation of P21 and P16. So, NR2F2 plays a significant role in the stemness maintenance of BM-MSCs (Zhu et al. Citation2016).
MicroRNAs
MicroRNAs (miRNAs) as a class of small noncoding RNAs are involved in post-transcriptional controlling of gene expression and different biological events. Previously, Aminoacyl-tRNA synthetase-interacting multifunctional protein-3 (AIMP3) was shown to be included in the macromolecular tRNA synthetase complex (Alizadeh et al. Citation2015, Hoseinzadeh et al. Citation2016). AIMP3/p18 controls cellular senescence in hMSCs probably by miR-543 and miR-590-3p (Lee et al. Citation2014). Clarification of miRNA-targeted genes in future studies will absolutely essential for superior comprehending of MSCs biology and easing expansion of novel MSC-based therapeutic methods for several illnesses (Alizadeh et al. Citation2016).
p21Cip1/Waf1 (p21)
The late-passage MSCs showed an increased expression of p21, a suppressor of the cyclin-dependent kinase (CDK). Cyclin-dependent kinase inhibitors (CKIs) stimulate cell cycle arresting by binding to CDK and impede cell cycle progression. The p21, a CKI, regulates pass into the cell cycle and is a molecular mediator of quiescence in HSCs and NSCs. Knockdown of p21 by Lentiviral-mediated shRNAs against p21 in late-passage MSCs enhanced the proliferation ability, the expression of sox2 and Nanog, and osteogenic potential in comparison with cells transduced with control shRNA (Yew et al. Citation2011).
Retinoblastoma (RB)
RB gene codes the RB protein, which controls cell-cycle development from G1 in to S phase by binding to E2F and preventing its action. RB expressed in early-passage of MSCs increases DNA methyltransferase 1 (DNMT1) expression and inhibits senescence in MSCs. The senescent process is reverse of stemness. So RB enhances the stemness of MSCs (Lin et al. Citation2014).
CD49f
CD49f, a cell-surface molecule has important roles in MSC sphere creation and in the determination of the differentiation ability of hMSCs. Also, reports showed cross-talk between the pluripotency genes OCT4 and SOX2 and CD49f in hMSCs and hESCs. OCT4 and SOX2 bound straight to the CD49f promoter, showing that OCT4 and SOX2 have positive roles in the expression of CD49f. CD49f have essential roles in preserving the stemness of hMSCs and hESCs. Specially, CD49f controls proliferation and differentiation capabilities of hMSCs and hESCs through the phosphatidylinositol-3-kinase pathway (PI3K/AKT/p53) (Yu et al. Citation2012).
Methyl-CpG-binding protein (MBD6)
Argonaute 2 (Ago2) is a key controller of cell fate in ASCs. Its expression is meaningfully down-regulated in late passages of cells. Nuclear localization of Ago2 is essential for the survival, proliferation, and differentiation of human adipose tissue-derived stem cells (hATSCs), mediated by the specific binding of the regulatory areas of functional genes, which positively or negatively changed gene expression. Ago2 targets genes that regulate stemness, reactive oxygen species (ROS) scavenging, and microRNA expression, all of which are critical for hATSCs survival and self-renewal. Ago2 stimulates cell proliferation and self-renewal by stimulating the expression of Oct4. MBD6 was identified as an Oct4 controlling gene. Knockdown of MBD6 expression decreased cell proliferation and finally prompted cell death. Besides, MBD6 stimulated cell transdifferentiation into neural and endodermal B-cells though meaningfully reducing differentiation into the mesodermal lineage. MBD6 is controlled by Ago2 via a collaboration with Oct4, that changes selfrenewal and gene expression in hATSCs (Jung et al. Citation2013).
Promyelocytic leukemia zinc-finger (PLZF)
Lack of PLZF expression is essential for stemness preservation and a primary occurrence at the beginning of MSCs commitment toward the differentiation processes of the three main lineages is the expression of PLZF (Kelly and Daniel Citation2006). Therefore, PLZF can be an important controller for the stemness maintenance of stem cells and act as an inducer of MSCs commitment (Djouad et al. Citation2014).
Signaling pathways
The appropriate use of stem cells for clinical applications needs a combined understanding of numerous signal inputs that regulate conservation of stemness, proliferation, commitment, and differentiation. Numerous signaling pathways have been involved in the control of MSC differentiation; though, complexities of pathway interactions, as well as apparently contradictory results in the literature, produce frequently confusing and disorganized knowledge base.
Fibroblast growth factor (FGF) is one of the greatest mutual growth factors used to expand stem cells, containing HESCs and numerous tissue type-specific stem cells. The molecular mechanisms underlying the regulation of stemness by FGF have remained obscure for a long time. The key signal transduction pathway initiated at the FGF receptors leads to the activation of Ras/ERK pathways through the control center FRS2α. New developing evidence proposes that the FGF-ERK axis regulates stemness by numerous types of action. There is a prevailing concept that FGF signaling is connected with stemness of a diversity of stem cells in an in vitro culture and in vivo. The FGF-FRS2α-ERK axis is commonly activated in cells by FGF stimulation. Then, FGF signaling prompts transcription and production of significant molecules such as master transcription factors and cytokines.
The master transcription factors prompt major variations in the transcriptome in cells. The cytokines regulate adjacent cells in a paracrine way. There is still a gap in information with regard to the relation between the FGF-ERK axis and gene sets that are ready to be stimulated or repressed. It is tempting to guess that epigenetical regulation is involved. Extra work is necessary to comprehend the molecular mechanisms underlying the control of stemness by FGF; and the information acquired by these efforts would surely help to the application of stem cells in regenerative medicine and novel cancer treatment in the near future (Gotoh Citation2009).
There are 22 members in the FGF family and 4 members in FGF receptor family.
They display a diversity of expression patterns in a spatial and temporal manner through development from the embryo to adult stage. FGF receptors belong to the receptor type tyrosine kinase family and are tyrosine-phosphorylated upon ligand stimulation. Evolving evidence shows that FRS2α, one of the docking/scaffold adaptor proteins, acts as a “control center” for intracellular signaling in response to FGF (Gotoh Citation2008, Reuss and und Halbach Citation2003).
Researchers have recognized pathways that MSCs use to differentiate into adipogenic, chondrogenic, and osteogenic lineages. Studies recognized activin-mediated transforming growth factor (TGF)-β signaling, platelet-derived growth factor (PDGF) signaling, and FGF signaling as the main pathways involved in MSC differentiation. The differentiation of MSCs into these lineages is influenced when these pathways are perturbed by inhibitors of cell surface receptor function. As growth and differentiation are closely connected processes, researchers furthermore studied the significance of these 3 pathways in MSC growth. These 3 pathways were essential and sufficient for MSC growth. Preventing any of these pathways reduced MSC growth; however, a combination of TGF-β, β-PDGF, and β-FGF was appropriate to grow MSCs in a serum-free medium up to 5 passages. Therefore, this study shows it is possible to forecast signaling pathways active in cellular differentiation and growth (Ng et al. Citation2008).
Genes of the PDGF pathway are expressed powerfully in undifferentiated MSCs. Fresh frozen pooled plasma (FFPP), which is rich in PDGF, has been used to substitute serum for MSC culture (Muller et al. Citation2006). Results give more indication for the significance of PDGF signaling in MSC growth.
Activin receptor was very powerfully expressed on undifferentiated MSCs. The activin/nodal pathway, that signals over the TGF-β pathway, cooperates with FGF signaling in preserving the pluripotency of ESCs. FGF is an essential growth factor for MSC expansion. Addition of β-FGF has been displayed to rises the growth rate and life span of MSCs from diverse species (Tsutsumi et al. Citation2001). The FGFR1 transcript was very weakly expressed in undifferentiated MSCs and was not up-regulated through differentiation (Benavente et al. Citation2003).
So these 3 pathways are necessary for expanding MSCs in serum-free media (SFM). It will be exciting to determine whether we can attain vigorous MSC and targeted differentiation over stimulating one pathway whereas hindering another one. Such a method can then be used to recognize combinations of growth factors and small molecules for clinical use of MSCs, where healthy expansion and targeted differentiation is required.
Signals mediated by TGF-β family members have been participated in the maintenance and differentiation of several kinds of somatic stem cells. Wnt signaling and TGF-β1 prompt the proliferation of hMSCs. TGF-β1 persuades Smad3-dependent nuclear accumulation of β-catenin in MSCs, needed for the stimulation of MSCs proliferation (Watabe and Miyazono Citation2009). In contrast, BMP-2 irritates Wnt3a signaling and prevents the proliferation of BM-MSCs over the interaction of a BMP-specific R-Smad with Dishevelled-1, an element of the Wnt signaling pathway. TGF-β family signaling might therefore differentially control MSCs proliferation through cross-talk with Wnt signaling (Liu et al. Citation2006). Wnts might also control MSCs maintenance, equally they do in the self-renewal of hematopoietic, intestinal, and NSCs. Wnt3a treatment rises ADMSC proliferation whereas preventing their osteogenic differentiation. Though discriminating the precise participation of Wnts is indicated by their pleiotropic effects. Examples of recognized Wnt actions contain the promotion of long-term culture expansion of stem cells, increased in vivo reconstruction of hematopoietic lines, and Wnt3a-specific conservation of skin and intestinal stem cell populations. Since stem cells might share signaling mechanisms with cancer cells that come from deregulated differentiation programs, the sustained B-catenin expression detected in some colon carcinomas proposes a downstream participation of B-catenin in Wnt control of MSCs self-renewal () (Kolf et al. Citation2007).
Figure 2. The canonical Wnt pathway (or Wnt/β-catenin pathway): an endogenous canonical Wnt pathway is active in stem cells: Wnt ligands are secreted and bind to their cognate receptor complex on the surface of receiving cells. The receptor complex consists of Frizzled, a 7-transmembrane domain receptor, and a low-density lipoprotein (LDL) receptor-related protein (LRP) coreceptor. The Drosophila homolog of this LRP coreceptor is called Arrow. The signal is transmitted through the intracellular protein Disheveled (Dsh/Dvl), which, in the presence of Wnt ligand binding, promotes inhibition of a destruction complex consisting of Axin, APC, and GSK3 (glycogen synthase kinase 3; Zeste-white 3/Shaggy in Drosophila). In the absence of a Wnt signal, the destruction complex phosphorylates B-catenin, targeting it for degradation by the proteasome. Inhibition of the of the destruction complex leads to an accumulation of cytoplasmic B-catenin and the subsequent translocation of B-catenin in to the nucleus. In the nucleus, B-catenin interacts with TCF/LEF transcription factors to regulate transcription of Wnt target genes. Destruction complex leads to an accumulation of cytoplasmic B-catenin and the subsequent translocation of B-catenin in to the nucleus. In the nucleus, B-catenin interacts with TCF/LEF transcription factors to regulate transcription of Wnt target genes.
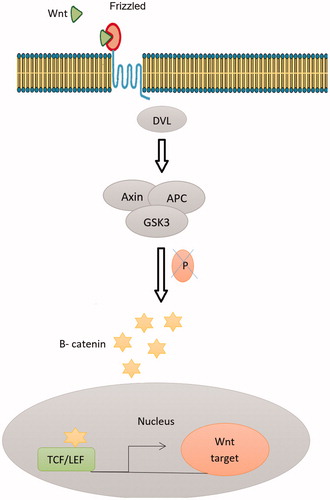
Organelles
The latest years, numerous factors were described to be straightly associated with the acts of stem cells, and mitochondrial activity has been regarded as key organelle in maintaining cell viability. Actually, while pluripotent cells comprise less mitochondria and depend mainly on glycolysis to gain their energy demand, differentiated cells show a more developed mitochondrial network and depend on oxidative phosphorylation for most of their energy production (Xu et al. Citation2013). Both the mitochondrial mass and mitochondrial DNA (mtDNA) content change during hESC differentiation. These observations powerfully propose the existence of an interaction among mitochondrial biogenesis and stem cell differentiation. Studies performed on three stem cell groups including ESCs, iPSCs, and somatic stem cells showed changes in mitochondrial morphology, dynamics and/or functions during stem cell differentiation and remarkably, proposed a relationship between stemness, stem cell differentiation, and mitochondrial biogenesis (Shyh-Chang et al. Citation2013).
Irradiation can prompt ROS gathering that causes DNA injury in MSCs and harms the stemness of MSCs. It decreases the expression of three positive markers (CD105, CD90, CD29), CFU-F competency and self-renewal ability of MSCs. Autophagy is described by the creation of a double-membrane bladders, called autophagosome, which surrounds cytoplasmic constituents and carries them to lysosomes for degradation. Autophagy prevents irradiation injury and preserves stemness through decreasing ROS generation in MSCs and reducing DNA injury in irradiated MSCs. Taken together, it has been concluded that the autophagy works as a defensive mechanism for MSCs stemness when they are unprotected to irradiation (Hou et al. Citation2013).
Extrinsic parameters
Anatomical origin and cell passage number
Canine adipose tissue-derived stem cells (cASCs) indicate a gradually reduced expression of the MSCs markers during passages and a decreased osteogenic differentiation potential. Moreover, records show that the anatomical origin of the adipose tissue has an obvious influence in the differentiation potential of the ASCs. HASC have been isolated from diverse fat depots, being reported that subcutaneous adipose tissue has an upper proliferation rate whereas visceral fat has a higher osteogenic differentiation capability (Requicha et al. Citation2012). Aksu and coworkers discovered that the ASCs from superficial subcutaneous layers had upper osteogenic potential, in males, comparing to deeper subcutaneous depots, so the ASCs from male donors had better proliferative performance (Aksu et al. Citation2008). Furthermore, Prunet-Marcassus et al. detected that the density of osteogenic clusters was meaningfully lower in cultures from epidydimal fat pads as compared to inguinal depot (Prunet-Marcassus et al. Citation2006).
Cellular microenvironment (niche)
The literature review shows that niche plays a significant role in determining stemness properties. Compared with frequently used monolayer cultures (2D), three-dimensional (3D) culture approaches suggest a cellular niche that is further similar to the in vivo microenvironment. Several 3D cell culture procedures, comprising the use of hydrogels, porous scaffolds or polymers, as well as cellular collections, have been established to keep the stemness properties of stem cells (Zhang et al. Citation2015). In addition to gene transduction, the exterior signals that regulate stem cell destiny together form the stem cell niche. The niche protects stem cells from reduction, although defending the host from over-exuberant stem-cell propagation. So, in vitro recapitulation of the in vivo stem cell niche, which establishes secreted factors, cell–cell contacts and ECM prepares a hopeful method in reprogramming stem cells for healing aims (Yan et al. Citation2014).
Oxygen pressure is as low as 1–2% in MSCs niche while it has been known that oxygen has a significant effect on the cells, hMSCs are frequently cultured at 21% O2. Cell proliferation, colony-forming potential, and stemness are enhanced through hypoxia (Zachar et al. Citation2011). There are merely suggestions that the stemness of MSCs is enhanced by hypoxic cell culture and increased expression of pluripotency marker genes (Rex-1, Oct-4) has been detected (Saller et al. Citation2012).
In normal culture situations, most animal cells are cultivated at 37 °C. Though, a number of studies have showed that decreasing the culture temperature less than 37 °C declines growth ratio, stress-induced senescence, and apoptosis levels and increased viability. The cultivation of BM-MSCs to differentiate in to Schwann cells was verified to be more effective at low temperature (Yoon et al. Citation2015).
A study showed the significance of cell shape in maintaining stemness and provided a new approach to maintaining MSCs multipotency via the use of microengineered substrates (Zhang and Kilian Citation2013). The cell shape and cytoskeletal tension are essential factors in stimulating multipotency. Prockop and coworkers have showed that subpopulations of small and quickly self-renewing MSCs have greater multipotentiality than their greater and further spread equivalents. Similarly, studies on hESCs have displayed that compact, rounded hESCs show higher expression of pluripotent transcription factors than flattened ESCs. Restricting cell shape can be a crucial element in preserving multipotency and culture situations limiting cell distribution either through substrate toughness, chemistry, or topography may be used to prolong the MSCs phenotype during ex vivo culture (Tsimbouri et al. Citation2012).
Sub-confluent culture is critically significant to preserve the stemness phenotype of placenta-derived MSCs (PD-MSCs) through expansion. The phenotypic profile of PD-MSCs when subcultured at 50–70% cell density stayed unaffected while PD-MSCs grown in confluent or near confluent culture missed MSCs markers almost totally. PD-MSCs passaged in subconfluent culture sustained their early marker profile and their ability to differentiate (Loges et al. Citation2004).
Extracellular matrix (ECM) which exists as a blend of numerous diverse proteins provides informative signs or cell fate choices, mainly via the integrin family of cell surface adhesion receptors. These informative signs can be classified as biochemical indicators (e.g. ECM elements) and biophysical signs (e.g. firmness and topography). ECM is a significant element of the cellular niche in a tissue, providing essential biochemical and physical signals to start and tolerate cellular actions (He et al. Citation2009). ECM regulates the activities of growth factors and cytokines, such as TGF-β, tumor necrosis factor (TNF), and platelet-derived growth factor (PDGF) by triggering inactive growth factors via proteolytic processing, by sequestering GFs and inhibiting them from binding to their receptors, or by straight affecting receptor activity. Cells exist in the ECM not only obtain ECM signals but besides impact ECM signaling by secreting ECM constituents and by making enzymes that cause proteolytic alteration of proteins and GFs in the ECM. The consequence is connection among cells and the ECM that describes cell performance. Regardless of tissue kinds, the ECM comprises of collagen fibers, laminin polymers, cell adhesion proteins such as fibronectin, high-molecular-weight proteoglycans, various GFs that often are in a hidden or covered form, and members of the small leucine-rich proteoglycan (SLRP) family. Researchers arranged an ECM prepared by BM-MSCs to rebuild the MSCs niche in vitro. This mesenchymal stem cell-derived ECM (MSCD-ECM) stimulates the duplication of MSCs and facilities the conservation of their plasticity (Chen Citation2010).
Stem cells can “sense” and act in response to the rigidity of the substance. Tissue culture dishes, commonly used for culturing mono layered cells, are firmer than the cell niche in vivo. Substrate rigidity has been known as a vigorous factor to define stem cell fate. For example, firm matrices that imitate collagenous bone are osteogenic, whereas soft substrates that match the intrinsic rigidity of ESCs stimulate self-renewal and pluripotency of ESCs (Engler et al. Citation2006). Substrates imitating muscle stiffness control self-renewal, increase survival, inhibit differentiation, and stimulate stemness of muscle stem cells. Polyacrylamide gels that mimic the elasticity of bone marrow can preserve MSCs in an inert state. Accordingly, it is safe to theorize that stem cells cultivated on substrates that imitate the rigidity of their own microenvironments can be a hopeful system to stimulate their self-renewal ability and preserve their stemness. In addition to rigidity, exterior mechanical induction plays important roles in stem cell fate (Guilak et al. Citation2009).
The activities of in vitro cultured cells might also be influenced by bioelectrical signal through application of electrical or electromagnetic currents to stimulate substrate and/or cell construct. For this aim, Chang et al. developed a biphasic current stimulator chip for stem cell culture. This stimulator chip can create both positive and negative currents in the same culture chamber. Additional investigation showed that the culture system can double NSCs proliferation by applying constant and clear levels of electric current. For hMSCs, pulsed electromagnetic field contact could increase cell proliferation through the log phase and it perhaps resulted from the shortening of the lag phase (Chang et al. Citation2011).
Some biochemical factors have also been described for the effective in vitro expansion of stem cells. For example, treatment with short-wave UV/ozone radiation on typical tissue culture dishes produces chemically defined surface with elevated intensities of some secondary ions (e.g. C2H5O+) which are promising to hESC colony formation (Saha et al. Citation2011). Other instance is that hMSCs preserve phenotype of stem cells when cultured on –CH3-modified glass surfaces, but processed osteogenic differentiation when cultured on –SH- and–NH2-modified glass surfaces 144 (Curran et al. Citation2006).
The communications among stem cells, as well as connections between stem cells and nearby differentiated cells, can control stem cell fate. The special mechanisms by which feeder cells support the undifferentiated growth of stem cells has not been described. This supporting effect is probable not caused by straight cell–cell connection but by special growth factors secreted by feeder cells. The hESCs might not be preserved using human feeder cells missing the capability to create bFGF, as indicated by the important reduced expressions of Oct-4 and Nanog in hESCs cultured in bFGF-knockout set compared with those in the control (Park et al. Citation2011). MSCs are used as feeder cells for ESCs and hiPSCs. Feeder layers have been applied broadly to support the growth and stemness potential of stem cells for in vitro cultures. Mouse embryonic fibroblast and mouse fibroblast cell line (SNL) are mutual feeder cells for hiPSCs culture. Owing to some difficulties in the use of these animal feeders and in order to shorten the therapeutic application of hiPSCs, researchers tested hMSCs as a powerful feeder system. This approach benefits from inhibition of possible contamination of animal origin feeder systems. Morphological, biochemical, cytochemical, and molecular analyses of iPSCs cultivated on MSCs feeder in comparison to those cultivated on SNL feeder verified the suitable features of MSCs and proposed it as feeder layer for expanded iPSC in vitro culture (Havasi et al. Citation2013).
Transduction of numerous genes
Transduction of numerous genes could regulate the fate of tissue-specific ASCs and ESCs. In spite of hopeful consequences, some challenges are important. First, genetically altered cells lack the long-term expression of the transgene which will meaningfully restrict the clinical and research applications. Second, most existing gene delivery approaches are founded on viral vectors in order to attain the constant transport of genetic information into eukaryotic genomes, which could have a major danger to the patients’ immune system (McMahon et al. Citation2007). Third, gene delivery approaches have the hazard of interfering inherent genes by chance insert of vector sequences. Furthermore, the methods of gene transduction are moderately intricate. Retroviral vectors can be effectively used to present therapeutic genes in to MSCs, because they allow maintainable expression of therapeutic genes while proliferation and differentiation potentials, chromosomal stability, and surface antigenicity of MSCs were not altered by retroviral transduction .Retroviral vectors can be securely used for transport of suicide genes to MSCs for ex vivo therapy without change in proliferation and differentiation abilities, chromosomal firmness and surface antigenicity of MSCs (Horwitz et al. Citation2005).
Cell culture methods
Researchers theorized that coculture of hNSCs with hMSCs might increase the stemness of hNSCs via Notch-1 signaling. This hypothesis was tested by evaluating functions of hNSCs and hMSCs under analytically planned coculture circumstances compared with monocultures, with or without Notch-1 handling in vitro. Statistics showed that expression levels of Notch-1 and HES1 were importantly upper in hNSCs cocultured with hMSCs than those of controls. Also, coculturing notably enhance immunoreactivity of CD15, a neural stemness marker, but reduces CD24, a marker of neural/neuronal commitment in hNSCs. The influence is independent from the physical state of cell growth since coculture and notch signaling in fact stimulates hNSC adhesion. Notably, coculture with hMSCs clearly increase hNSC proliferation rate without declining their lineage differentiation abilities. The consequences propose that coculture of hNSCs with hMSCs increases stemness of hNSCs incompletely via activation of Notch-1 signal transduction (Haragopal et al. Citation2015).
Floating cultivation can sustain the stemness MSCs derived from Wharton’s Jelly (WJCs) of the umbilical cord. In floating culture, MSCs derived from WJCs can sustain stemness, and differentiate to osteogenic, chondrogenic, and adipogenic lineages. Floating culture planned to preserve the stemness on ultra-low-attachment plates. Seeded WJCs made spheres and were cultivated for 21 days. After aspirating with a pipette, WJCs spheres were put on slides by the cytospin method. With immunostaining, the spheres of WJCs expressed stem cell marker proteins, such as Oct3/4, Sox2, Klf4, and c-myc, while cultured adherent WJCs did not. So, WJCs can preserve their stemness in a floating culture (Higuchi et al. Citation2012).
A novel cell culture technique with innovative extremely flexible culture plate whose diameter is uniformly extended using a motorized mechanical tool was developed recently. This method is appropriate for all adherent cells that are chosen to grow quickly in huge amounts for regenerative medicine. Dynamic expansion of culture surfaces is now applied to expanse cells on membranes made of poly-dimethylsiloxane (PDMS); the material properties of PDMS limit surface extension to 130% (Majd et al. Citation2009). To attain upper culture surface expansion, an obvious and bio-compatible high-extension silicone rubber (HESR) was identified that permits isotropic surface expansion up to 1000%. Dynamic HESR culture conserves an undifferentiated and nonfibrogenic hMSCs phenotype. This method has two main benefits over typical cell culture: (a) elongated conservation of the cell phenotype since cell surface protein degradation is decreased by enzymatic passaging, and (b) higher cell yield because dynamic culture surface expansion removes contact inhibition of growth and preserves cell growth in the log phase (Goffin et al. Citation2006). Using this culture method may reduce our need for scaffolding of MSCs for supplying enough amounts of cells for tissue engineering.
3D culture systems
Cells developed in a 3D environment mimic in vivo cells in morphology and molecular control. The 3D culture provides additional dimension for cell adhesion, which could affect integrin ligation, cell contraction, and connected intracellular signaling. In 3D cell culture models, cells are fixed in biomaterials, cultured as cellular spheroids, or developed on 3D scaffold materials. Limitations in the distribution of soluble elements might be responsible for some of the variances detected between 2D and 3D culture systems (Han et al. Citation2012). Several materials, such as poly(lactic-co-glycolic acid) (PLGA), alginate, collagen and chitosan have been used to establish 3D culture system, which could affect the migration, proliferation and differentiation of MSCs. Amongst them, collagen is a best 3D biomaterial| for scaffolds because it is the key element of the ECM in connective tissues and also it has little antigenicity and proper biocompatibility (Cheng et al. Citation2013).
Producing a third dimension for cell culture is obviously more relevant, but needs multidisciplinary multidisciplinary approach and multidisciplinary expertise when entering the third dimension, researchers need to design scaffolds for supporting the organisation of cells or the use of bioreactors for regulating nutrient and discarded product exchange. As 3D culture systems become more advanced and relevant to human and animal physiology, the capability to design and develop co-cultures become probable as does the ability to integrate stem cells. The primary goals for developing 3D cell culture system differ broadly and sort from engineering tissues for clinical delivery through to the improvement of models for drug screening (Haycock Citation2011).
To comprehend completely how tissues form and function, as well as their pathophysiology, it is critical to study how cells and tissues act as parts of whole living organs that are composed of several, tightly opposed tissue types that are extremely dynamic and variable in terms of their 3D structure, biochemical microenvironment, and mechanical properties.
Unfortunately, most studies on cell and tissue regulation have depend on analysis of cells grown in 2D cell-culture models that fail to rebuilding the in vivo cellular microenvironment; consequently, these cultures normally do not preserve their differentiated functions. Efforts to address these restrictions led to the development of 3D cell-culture models in that cells are grown in ECM gels. This approach enhances expression of differentiated functions and progresses tissue organization (Pampaloni et al. Citation2007).
3D cell culture advantages
3D cultures are in vitro cultures where immortalized cell lines, stem cells or explants are located inside hydrogel matrices that imitate in vivo cell environments. Cells in 3D, as compared to 2D, indicate enhancements in numerous studies of basic biological mechanisms, such as: cell number checking, morphology, viability, proliferation, differentiation, reply to stimuli, cell–cell communication, migration and invasion of tumor cells into nearby tissues, drug metabolism, angiogenesis stimulation and immune system evasion, gene expression and protein synthesis, general cell function and in vivo relevance.
3D cell cultures provide additional precise depiction of cell polarization meanwhile in 2D the cells can only be moderately polarized. Furthermore, 3D cell cultures have more stability and longer lifespans than cell cultures in 2D. Also, 3D collections can be cultured for longer, at least up to 4 weeks, as compared to almost 1 week with 2D monolayer culture because of cell confluency. So, they might be more suitable for long-term studies and surviving cells and for representing long-term effects of the drug. Through the 3D environment, the cells are allowed to grow uninterrupted compared to the 2D models, where consistent trypsinization is essential in order to provide them with adequate nutrients for normal cell growth.
Compared to 3D, 2D surfaces result in a uniform cell population, which leads to an overestimation of the effects determined from in vitro cytotoxic screens, resulting in an incompetence of the candidate agents in vivo. Hence, 2D culture does not let testing the efficacy of special candidate compounds such as antiangiogenic and vasculogenic agents previous to in vivo approaches and permits almost exclusively to recognize antiproliferative candidate agents. The proliferation of tumor cells grown in monolayer cultures is quicker than that of 3D spheroids, shows diverse metabolic profiles and naturally shows enhanced sensitivity in treatment such as chemotherapy or radiation therapy.
Finally, over analysis of microRNA, metabolic profiles and gene expression it has been displayed that 3D generating collections show a genotype meaningfully more relevant to in vivo, as compared to 2D monolayer cultures (Antoni et al. Citation2015).
Principles of 3D cell culture
Important standards contain select of material for the scaffold, the source of cells, and the definite methods of culture, which differs noticeably according to the tissue of study. Numerous culture models can be distinguished: the study of complete animals and organotypic explant cultures, microcarrier cultures, cell spheroids, and tissues-engineered models. Whole animal and organotypic explants are mainly used in studies where an entire requirement for tissue-specific information is necessary (Toda et al. Citation2002).
Many cell kinds have the natural affinity to collection. Cells can re-establish common contacts and particular microenvironments that let them to express a tissue-like phenotype. Cellular spheroids show the most common use of ex vivo 3D cultures. They are simple 3D models that can be created from an extensive range of cell types and procedure because of the affinity of adherent cells to aggregate.
For microcarrier culture, beads derive from glycosaminoglycans, dextran, gelatin, and other porous polymers can be used as a 3D support for the culture of anchorage-dependent animal cell lines. Directional culture is highly appropriate for tissue regeneration applications like muscular repair and uses native extracellular proteins, such as laminin or collagen, with other matrices such bio-mimetics.
Organotypic cultures comprise both an epithelial layer and a mixture of ECM protein, such as fibroblasts and collagen. They can besides use synthetic matrices or artificial scaffolds. Cellular aggregates need the careful exchange of nutrients and gases besides to spatial control, and problems with cell death rise when aggregate thickness of 1–2 mm arise through an absence of mass transfer, principally through a limited exchange of nutrients and waste metabolites (Griffith and Swartz Citation2006).
So, the need of scaffolds with a design reflecting the tissue of interest becomes obvious. 3D matrices are accessible in a large diversity of materials with diverse porosities, permeabilities, pore sizes, and mechanical characteristics designed to reflect the in vivo ECM of the special tissues being modeled: glasses, ceramics, metals, natural and synthetic polymers, and also composites.
Polymers are the most common materials used, since the control of their chemical and structural properties, important for dictating the adhesion and diffusion of living cells and protein adsorption and in turn cellular attachment are easier to control (Allen et al. Citation2006).
Other possessions to consider include biocompatibility, wettability, mechanism, and methods for fabrication. Because of the variety of material and structural choices for scaffolds, they are widely used in many applications.
As the last goal of a scaffold is to produce features found in the ECM necessary for native cell function, the macro-, micro-, and nano-scale elements of 3D scaffolds must be debated.
Macroscale structures are significant for determining the total size and shape of a scaffold, dependent on application. Nano-scale features are important for nutrient supply and functional effects because of the size of several cell-signaling molecules.
Microscale must be considered for permeability, pore geometry, pore interconnectivity, pore size, distribution and elements of surface topography. Micro-scale features eases mass transport, diffusion of nutrients, metabolic wastes, and soluble molecules and can activate special genes and moderate cellular behavior in differentiation and proliferation. They also affect the overall robustness of the scaffolds and therefore the desired application, such as use in a bioreactor or multi-wells plate.
Bioreactors enable the exact and reproducible control over many environmental situations necessary for cell culture, comprising temperature, medium flow rate, pH, oxygen, nutrient supply, and waste metabolite removal. Bioreactors have been adjusted for 3D cell culture and are able to preserve and check the environment through growth (Martin et al. Citation2004).
Four groups can be recognized: rotating wall vessels, straight perfusion systems containing spinner flasks hollow fibers and mechanical force systems.
Rotating wall vessels were initially designed by the National Aeronautics and Space Administration to simulate microgravity. They provide constantly moving culture situations where cell constructs are grown under low shear stress forces and enable high amounts of mass transfer (Unsworth and Lelkes Citation1998).
Mechanical force systems use the mechanism by which tissues answer to force through growth. Cells such as osteoblasts are recognized to be mechanoreceptive and reply to force with the activation of intracellular signal transduction pathways (Rubin et al. Citation2006).
When joined with scaffolds, bioreactors can be used for specialty goals that use mechanical load or electrical. 3D cell culture in gels can be joined with other models like scaffolds, spheroid cultures and microchips. Gels uses as substrates for 3D cell culture. They have a soft tissue-like rigidity and aim to imitate the ECM. They could be made from ECM combinations of natural origin, such as collagen and alginate. The most commonly used is a reconstituted basement membrane preparation derived from mouse sarcoma (Matrigel™, Corning Life Sciences, Tewksbury, MA), rich in ECM proteins, such as laminin and collagen, enzymes, and growth factors. Gels made from animal sourced natural ECM may differ and change in structure over time so synthetic gels, such as the polyethylene glycol-based hydrogels, have been developed. Cells for 3D cultures and tissue engineering can be assembled according the source of them into stem cells, allogenic cells, autologous cells, xenogenic cells, animal-derived primary cells, cell lines, and genetically altered variants of all the above cell kinds (Antoni et al. Citation2015).
Types of 3D culture systems
Scaffolds
MSCs cultured inside hydrogels showed a major raise in the relative expression of transcription factors linked with self-renewal and pluripotency in comparison with MSCs in standard cell culture. Studies have confirmed the ability of hydrogel systems to keep the self-renewal of ESCs and to preserve these cells in their undifferentiated states. Although these artificial matrices have provided a means of circumventing feeder layers for ESCs culture, ADMSCs do not need culture on feeder cells. Additional modifications of the hydrogel matrix to boost its niche-like properties can give more regenerative profit to this MSC-based beneficial construct (Gerecht et al. Citation2007; Rustad et al. Citation2012).
The collagen scaffolds are appropriate for rat MSCs adherence and proliferation (Kador and Goldberg Citation2012). Compared to MSCs under 2D culture, 3D MSCs importantly preserve upper expression levels of stemness genes, generate high incidences of CFU-F and present increased osteogenic and adipogenic differentiation competency after stimulation. Therefore, 3D collagen scaffolds may be useful for MSCs expansion whereas keeping the stem cell properties in vitro (Han et al. Citation2012).
Collecting evidence revealed that substrate nanotopography is the important parameter in stemness maintenance of stem cells. TiO2 nanofibrous surfaces increased the expression of stemness markers. TiO2 nanofibrous surfaces can be used to improve cell adhesion and proliferation whereas concurrently preserving the stemness of ADSCs (Chen et al. Citation2012).
ADSCs and GMs gathered in to cell–microsphere constructs (ADSC-GMs), with enhanced cell–cell and cell–matrix interactions can maintain the stemness properties and increase the differentiation capabilities of ADSCs into adipogenic, osteogenic, and hepatic lineages when compared to the ordinary 2D cultures. Furthermore, the ADSC-GMs increase the tubular network creation of human umbilical vein endothelial cells (HUVECs) on matrigel and therefore seem to increase the proangiogenic properties of ADSCs. This would cause better vascularization of the regenerating tissue. Using these ADSC-GMs with such improved regenerative capabilities can accelerate tissue regeneration and so increases the therapeutic potential of ADSCs (Ahn et al. Citation2015).
Alumina is one of the greatest prevalent implanting substances used in orthopedics, but limited records are accessible about the cellular response of MSCs grown on nanoporous construction. The cellular behavior is influenced by difference in pore size, supplying new vision in to the possible application of this new bioadaptable material for the evolving field of tissue engineering .The helpful influence of the surface topography of nanoporous alumina on cell behavior propose that this novel bioadaptable material, when used in mixture with stem cells, can function as an active tissue-engineering scaffold in regenerative applications (Song et al. Citation2013).
Cellular spheroids
Cellular spheroids which mimic the in vivo conditions, supply a 3D culture conditions more than a cell encapsulation system. Cellular spheroids are collective cells and free of external materials (Baraniak Citation2012). Spheroids can connect with culture materials, but no extraneous material is existing inside them. The methods for providing cellular spheroids by ASCs include, microwells, hanging drops, non-adherent surface, and rotary wall vessel bioreactors. The gene expression of self-renewal markers in MSCs and ASCs are meaningfully upper for the cells in spheroid in contrast to the monolayer cells, showing that the cells in spheroid maybe are in an uncommitted state. Short-term spheroid formation of ASCs before monolayer expansion can enhance their regenerative capacity by stimulating stemness, angiogenesis, and chemotaxis, thus providing enhanced therapeutic potential for tissue regeneration (Cheng et al. Citation2013). The studies showed that hASCs suddenly made 3D spheroids on chitosan films. A large number of ASCs inside the spheroid were viable, and the cells formed additional extracellular molecules, like laminin and fibronectin. The creation of adhered spheroids on chitosan and chitosan-HA membranes could support the expression of stemness marker genes of MSCs and rise their chondrogenic differentiation ability. The Rho/Rho-related kinase (ROCK) signaling pathway might be participate in spheroid formation (Huang et al. Citation2011).
Researchers use a microgravity bioreactor (MB) to progress a technique for biomaterial-free-mediated spheroid formation to preserve the stemness properties of ADSCs. ADSCs instinctively formed 3D spheroids in the MB. Spheroid-derived ADSCs presented increased levels of pluripotent marker, proliferative capacity, colony-forming efficiency, stemness properties, and therapeutic potential. So, spheroid culture in an MB can be an appropriate efficient technique to sustain stemness, without the participation of biomaterials that is use for clinical applications of in vitro cultured ADSCs (Zhang et al. Citation2015).
Cell sheet formation
Cell sheet technology has introduced as an important tissue engineering approach. In a study, researchers discovered that L-ascorbic acid 2-phosphate (A2-P)-induced cell sheet, a steady form of ascorbic acid, significantly increased ASCs proliferation and stimulated ASCs sheet production in 7 days with plentiful ECM removal. These data showed that A2-P increased stemness of ASCs by collagen production and cell sheet creation. A2-P-stimulated collagen synthesis in ASCs can be facilitated over ERK1/2 pathway (Yu et al. Citation2014).
Biomaterials and culture additives
Biomaterials, from both natural and synthetic basis use to progress 2D and 3D cell culture methods for the expansion of ASCs. The modifications of biomaterials in terms of topography/construction, mechanical characteristic, and biochemical issues are done (Yan et al. Citation2014).
In vitro cell culture is typically done on flat substrates, which hold the benefits as an easy approach to recognizing the effect of unique niche constituents on stem cell fate. The mutual method of rising cells on tissue culture polystyrene (TCPS) is regularly being substituted by culturing cells on substrates with a more suitable composition. Stem cells can feel and response to ECM elements over the communication between integrins on the cell surface and similar ligand-binding motifs on ECM molecules (Griffith and Swartz Citation2006). Designing biomaterials imitating ECM is a moderately useful and harmless method to control the fate of stem cells. Regarding that most cells need adhesion to an ECM for survival and development through ECM-integrin contact, natural biomaterials containing ECM constituents have been verified for stem cell expansion. Some of these materials are laminin, collagen, matrigel, and vitronectin (Loeser Citation2002). Also other materials could be added to cell culture as a supplement to improve MSCs properties and maintain their stemness such as, antioxidants, ascorbic acid, fatty acids, etc.
Antioxidants have the potential to increase the proliferation of ADMSCs in vitro, while the full mechanisms and probable side effects are not completely surveyed. Throughout logarithmic phase growth, contact to ImF-A medium supplemented with antioxidants (N-acetyl-L-cysteine and ascorbic acid-2-phosphate) resulted in a higher ratio of ADMSCs in the S phase of the cell cycle and a reduced percentage in G0/G1 phase. This resulted in an importantly reduced cell-doubling time and enhanced number of cells in the antioxidant-complemented cultures. In the existence of antioxidants and fibroblast growth factor 2 (FGF2), ADMSCs quickly proliferate and preserve their stem cell properties, also their osteogenic and adipogenic abilities are increased. The mechanisms by which antioxidants prompt cell proliferation are like those participate in hypoxia, but the in vitro culture environment of antioxidant complemented medium under normoxic situations may be further steady than hypoxic situations. Therefore, a mixture of antioxidants and FGF-2 under normoxic conditions may supply a simple and quick technique for in vitro expansion of both ADMSCs and BMMSCs (Sun et al. Citation2013).
Vitamin C (ascorbic acid) treatment stimulates MSCs sheet creation and tissue regeneration by rising telomerase activity. Cell sheet engineering has been advanced as an alternative method to expand MSC-mediated tissue regeneration. Ascorbic acid is able of stimulating telomerase activity in periodontal ligament stem cells (PDLSCs), consequently, up-regulated expression of ECM type I collagen, fibronectin, and integrin β1, stem cell markers as well as osteogenic markers. Antioxidants such as ascorbic acid can be used to expand MSCs whereas maintaining their differentiation capability (Wei et al. Citation2012; Wei et al. Citation2014).
Crucial fatty acids, specifically x-6 (n-6) and x-3 (n-3) polyunsaturated fatty acids (PUFA), and their metabolites are crucial constituents of cell arrangement and action, and can so affect stem cell fate. The accessible evidences show that n-6 and n-3 PUFA and their metabolites can stimulate the proliferation and differentiation of several stem cell types via numerous mechanisms (Kang et al. Citation2014).
Extensive clinical applications of stem cells need chemically described materials that are cheap, with manageable physical characteristics and long-term firmness. Because of that, defined synthetic materials (synthetic peptides like synthetic peptide acrylate surfaces (PAS), connecting segment-1 and synthetic polymers like poly[2-(methacryloyloxy)ethyl dimethyl-(3-sulfopropyl)ammonium hydroxide] (PMEDSAH)) have been established to substitute the complex natural materials owing to simplicity of scale-up and reduced risks of illness diffusion from unidentified pathogens (Yan et al. Citation2014).
Effects of platelet-rich plasma (PRP) on proliferation, migration, stemness, maintenance of MSCs immune-modulatory properties, and appearance of senescence phenotype have been discovered. PRP can be quickly and simply gained by centrifugal segregation from entire blood. PRP (as platelet lysate or releasate) maintains the immune-privileged potential of MSCs and may delay the appearance of the senescent phenotype (Soomekh Citation2011).
Exogenous glucocorticoids (GC) have a role in the control of BM-MSCs proliferation and differentiation but the role of endogenous GC is not well understood. Researches have studied the influence of the obstruction of endogenous GC using RU486, an antagonist of the GC receptor, on the in vitro proliferation and differentiation abilities of hMSCs (Yu et al. Citation2012). It was revealed that RU486 treatment importantly improves the proliferation of hMSCs, while the ideal dose of RU486 for this increase in proliferation varies according to the gender of the MSC donor. This enhancement in MSC proliferation with RU486 treatment was upper in MSCs from male donors than that from females.RU486 pretreatment meaningfully increased the expression of mRNA for alkaline phosphatase in hMSCs and the mRNA expression of osteocalcin of these cells increased earlier after their contact to osteogenic differentiation medium. RU486 treatment increased the expressions of FGF-2 and Sox-11 in hMSCs. These outcomes show that obstruction of endogenous GCs might be established as a novel approach to efficiently advance the proliferation and osteochondral differentiation abilities of hMSCs for potential clinical applications. The up-regulation of FGF-2 and Sox-11 shows that impeding endogenous GC may function to preserve the stemness of MSCs and avoid aging (Crisostomo et al. Citation2007, Deasy et al. Citation2008, Larson et al. Citation2010, van Staa et al. Citation2005). Extra studies will be necessary to determine the potential long-term effects of RU486 treatment on these bone marrow cells.
Researchers studied whether histone deacetylase inhibitor trichostatin A (TSA) might repress the histone H3 deacetylation so preserving the basic property of MSCs (Alizadeh et al. Citation2013, Hassan and Nosratollah Citation2014). Administration of low concentrations of TSA in culture meaningfully inhibit the morphological variations in MSCs that happen through culture expansion, increase their propagation while retaining their cell interaction growth inhibition property and multipotent differentiation capability. Furthermore, TSA stabilized the expression of the overhead pluripotent genes and histone H3 acetylation levels, so help as an active culture additive to sustain the basic stemness of MSCs through culture expansion (Alizadeh et al. Citation2013, Han et al. Citation2013).
Polymers for preserving the stemness of MSCs
A polymer is a big molecule, or macromolecule, composed of numerous repeated subunits.
Owing to their wide range of properties, both synthetic and natural polymers show an important and pervasive role in everyday life (Heller, Citation1987).
Polymers array from familiar synthetic plastics such as polystyrene to natural biopolymers like DNA and proteins that are essential to biological construction and function. Polymers, both natural and synthetic, are produced via polymerization of several small molecules, identified as monomers. So their large molecular mass relative to small molecule composites produces distinctive physical properties, containing stiffness, viscoelasticity, and an affinity to produce glasses and semicrystalline structures instead of crystals (McCrum et al. Citation1997).
Polymers are of two types: natural polymeric materials such as silk, amber, shellac, wool, and natural rubber also, other natural polymers exist, like cellulose, that is the key component of wood and paper. The list of synthetic polymers contains synthetic rubber, phenol formaldehyde resin, nylon, neoprene, polyvinyl chloride (PVC or vinyl), polystyrene, polypropylene, polyethylene, polyacrylonitrile, PVB, silicone, and many more.
Natural polymers such as collagens, fibrinogen, and elastin work like the body’s native ECM. This ECM provides structure and mechanical integrity to tissues, in addition to connecting with the cellular constituents it supports to help simplify and control daily cellular processes and wound healing (Farach-Carson et al. Citation2007). A perfect tissue engineering scaffold would not merely replicate the construction of this ECM, however, would also replicate the several functions that the ECM performs.
Collagen fibrils and their networks create the ECM for most hard and soft tissues (bone, cartilage, tendon) in the human body. Collagen has a key role in preserving the biologic and structural integrity of the ECM architecture, and shows diverse morphologies in various tissues. Furthermore, it is extremely dynamic, suffering continuous alteration for appropriate physiological purposes (Barnes et al. Citation2007).
Joining collagen with other natural or synthetic polymers has enabled tissue engineers to fine tune the desired properties of the electrospun scaffolds. Collagen performs as an adhesion protein in the native ECM, increasing cell attachment and proliferation over special interactions between domains in collagen molecules and integrin receptors in the cell membrane (i.e. RGD (Arg-Gly-Asp)). Therefore, the existence of collagen in a synthetic polymer scaffold is intended to convey biocompatibility and bioactivity to the scaffolds, with the synthetic polymer providing mechanical integrity to the structure.
Other methods for presenting proteins into nanofibrous structures, such as covering or grafting, result in slow mass transfer of the proteins into the 3D porous materials. Electrospinning of a combined collagen blend is not only simpler, but also avoids the slow mass transfer process and uses lesser quantities of chemical reagents. Furthermore, the existence of collagen on the surface and inside the structure provides sustained cell recognition signals with polymer degradation, which is critical for cell function and development (He et al. Citation2005, Pilehvar-Soltanahmadi et al. Citation2015).
In the ancient decade, the process of electrospinning has confirmed effective in creating non-woven ECM analogue scaffolds of micro to nanoscale diameter fibers from a range of synthetic and natural polymers. The capability of this fabrication method to use the above-mentioned natural polymers to form tissue engineering scaffolds has generated hopeful results, both in vitro and in vivo, owing in part to the boosted bioactivity afforded by materials normally found inside the human body (Sell et al. Citation2010).
The connection of stem cells with their culture substrates is essential in regulating their fate and function. Diminishing stemness of hMSCs through in vitro expansion on TCPS strictly confines their therapeutic effectiveness before cell transplantation into injured tissues. So, several kind of natural and synthetic materials have been manipulated to reproduce in vivo matrix environments. Researchers developed a series of patterned polymer matrices for cell culture by hot-pressing poly(ɛ-caprolactone) (PCL) films in femtosecond laser-ablated nanopore molds, creating nanofibers on flat PCL substrates. HMSCs cultured on these PCL fiber matrices considerably increased expression of critical self-renewal factors, OCT4A and Nanog, along with markers of cell–cell interaction PECAM and ITGA2. The outcomes propose the patterned polymer fiber matrix is a favorable model to preserve the stemness of hMSCs (Hofmeister et al. Citation2015).
Poly(ɛ-caprolactone) (PCL), a mutual synthetic polymer used in biomedical applications, has been revealed to help hMSCs toward neural lines when the material’s hydrophobic nature is combined with soluble factor neural-induction media, Synthetic copolymers, like poly(vinylalcohol)-PCL, produce 3D structures reminiscent of native tissues and when loaded with growth factors have stimulated hMSC differentiation into chondrocytes, thus creating structurally healthy cartilage tissue (Jahani et al. Citation2015). Joining polymers with minerals and metals have also revealed ability in encouraging MSCs to produce complex coated tissue structures such as bone-cartilage interfaces. Furthermore to providing templates to examine MSC answers to high-throughput chemical screening protocols. In conclusion, natural polymers like collagen have also been altered with peptides to encourage better tissue regeneration by jump-starting growth answers in slow proliferating cells like dorsal root ganglion cells (Hosseinkhani et al. Citation2013).This method meets the need for scalable, extremely repeatable, and tuneable models that mimic ECM features that signal for maintenance of hMSC stemness.
Chitosan is a chitin derivative that is broadly found in nature. It is composed of D-glucosamine and N-acetyl-glucosamine, that are joined by β (1→4) glycosidic bands. Because of its biocompatibility, nontoxicity, and non-immunogenicity, chitosan is compatible and cross-linked with collagen. The diverse kinds of chitosan and its deacylation level have been found to influence cell adhesion and proliferation (Yang and Young Citation2009).
MSCs cultured using chitosan membranes create spheroids. Furthermore, the expression of stemness marker genes, containing Oct4, Sox2, and Nanog, increased meaningfully when MSCs were cultured with chitosan membranes compared with 2D culture systems. In conclusion, MSCs cultured using chitosan membranes were found to have an enhanced ability to differentiate into nerve cells and chrondrocytes. In summary, MSCs cultured on chitosan membranes preserve their stemness and homing capabilities. This outcome may be further examined for the improvement of novel cell-based therapies for diseases including neuron-like cells and chondrogenesis (Li et al. Citation2013).
Both intrinsic and extrinsic parameters
Wnt proteins, bFGF, TGF-β, and their family followers, and IL-6 have showed their influences on stem cell conservation. Since the influences of GFs on stem cells are dose dependent so to make the most cell numbers for their clinical, the quantity should be optimized. For instance, low doses of bone morphogenetic proteins (BMP-4) meaningfully rise the existence of hASCs and preserve their stemness as well as multipotency, while high doses increase apoptosis and decrease cell proliferation. Furthermore, great production price of GFs still is existing and harmless replacements to costly GFs are necessary for clinical usage (Yi et al. Citation2011; Zeng and Nusse Citation2010). Researches indicated that GFs secreted by MSCs control the maintenance of stemness. GFs were separated in to two groups, those which play roles in proliferation or those which direct differentiation of BM-MSCs. In general, throughout culture, the expression of GFs was declined slowly and prompted the delay of proliferation or differentiation according to the types of GFs. Finding data recommend that the types, amounts, and mixture of GFs must be considered to obtain a high value of BM-MSCs maintaining the stemness needed for stem cell therapy. In summary, reduction of GF could persuade autophagy, aging, and growth obstruction of BM-MSCs (Eom et al. Citation2014). A recent study showed that IL-6 gene expression is meaningfully up-regulated in undifferentiated MSCs as compared to their differentiated derivatives. Totally, these informations display that IL-6 preserves the proliferative and undifferentiated state of BM-MSCs, a significant parameter for the optimization of both in vitro and in vivo handling of MSCs. Leukemia inhibitory factor (LIF), a pleiotropic cytokine, preserves the stem state of ESCs and MSCs (Kolf et al. Citation2007). The recombinant human erythropoietin (rhEPO) can not only keep features of stem cells but also preserve its multi-lineage differentiation ability after suitable EPO treatment. But extra investigation on the involved mechanism can lead to a better understanding between EPO and MSC stemness maintenance (Ye et al. Citation2010).
Parameters related to donor of MSCs
Phenotype, gender, and donor age
BM-MSCs from younger donors indicated increased expression of MCAM, VCAM-1, ALCAM, PDGFRβ, PDL-1, Thy1, and CD71, and led to lesser IL-6 production when co-cultured with activated T cells. Female BM-MSCs indicated increased expression of IFN-γR1 and IL-6β. High-clonogenic BM-MSCs were smaller, divided more quickly and were more common in BM-MSC preparations from younger female donors. The mesodermal differentiation capability of BM-MSCs was unaffected by donor age or gender but was affected by phenotype. The expression of Oct4, Nanog mRNA in BM-MSCs was much lower compared to pluripotent stem cells and was not connected with donor age or gender (Siegel et al. Citation2013).
Woman’s reproductive status
There was no important difference in the differentiation capability among the pregnancy, premenopause, or menopause, while the proliferation level of ASCs from pregnant women was significantly greater. The proliferation rate of ASCs after estrogen treatment stayed unaffected, regardless of the upper proliferation rate in pregnant women, ASCs presented uniformity in cell differentiation capability and were unaffected by donor status. This proposes that factors except estrogen are accountable for the variance in proliferation in pregnant women (Ng et al. Citation2009).
Sex-specific hormones: estrogen
Since estrogen receptor is present on stem cells, proposing that estrogen might alter the action of those cells (Zarghami et al. Citation2005). Estradiol, or 17β-estradiol, is a steroid and estrogen sex hormone, and the primary female sex hormone that increases proliferation and migrations of some stem cells. Therefore, amendment of the function of stem cells by estrogenic inducement can rise the function of stem cells. Moreover 17β-estradiol motivates the expression of osteogenic genes for exmple ALP, collagen I, and TGF-β1 in MSCs (Hong et al. Citation2011).
Obesity
Studies were done to estimate how the adipogenic environment affects the stemness of the resident multipotent stem cells. To attain this aim, human omental multipotent stem cells (hO-MSCs) were isolated, expanded, and characterized in both healthy thin and morbidly obese individuals. Researchers detected variations in telomerase activity and DNA telomere length, proposing a reduced cell proliferation, early senescence, and enhanced cytokine secretion connected with increasing body mass index of the patients. Along with the latter finding, the hO-MSCs derived from patients with morbid obesity miss their multilineage differentiation capability associated with a dysregulation in the Wnt, Notch, and Sonic Hedgehog signaling pathways. Furthermore, microRNAs involved in the control of stemness, cell differentiation, and senescence were also up-regulated in fat individuals. Obesity causes a general short circuit in the stemness gene network of hO-MSCs (Roldan et al. Citation2011). Consequences propose that obesity leads to a common failure in the homeostasis regulatory network of ASCs. These data support that adipose tissue is an appropriate source of ASCs, but obesity has to be considered when using these cells for regenerative medicine applications (Mohammadzadeh and Zarghami Citation2009; Perez et al. Citation2015).
Conclusion
It must be noted that cell fate is affected by a diversity of factors in mixture. Vigorous, harmless, and economical approaches by described biomaterials can recreate the in vivo niche of stem cells, which will eventually profit in cooperation research and ordinary clinical aim. The progress of 3D culture methods by using biomaterials reviewing the in vivo stem cell niche will possibly offer better micro-environments for stem cells in comparison with the old 2D monolayer culture methods. Novel visions should be employed in to the change of biomaterials such as the surface features, rigidity, and surface interaction. However, advancement has been made to conserve the self-renewal capability of stem cells, we are yet distant from the large-scale extension of undifferentiated stem cells for therapeutic aim. In the future, biomaterials should be planned to prepare a mixture of physical and biochemical parameters to expand stem cells. Furthermore, the final purpose of prolonged cells needs an in vivo assessment to verify their regenerative capability, for instance, by replacement of extended cells into animal models. This requests association between several fields, comprising cell and molecular biology, nanotechnology, pharmacology, biomaterials, and medicine.
Acknowledgements
The authors gratefully acknowledge the research center for their sincere support offered during the project. The authors are so thankful to their colleague at Tabriz University of Medical Sciences, for her kind help in performing experiments.
Disclosure statement
The authors disclose no conflicts.
Funding
This work was supported by grant from the Stem Cell Research Center, Tabriz University of Medical Sciences, Tabriz, Iran (Project Number: 94/2-1/2).
References
- Ahn SH, Lee HJ, Lee J-S, Yoon H, Chun W, Kim GH. 2015. A novel cell-printing method and its application to hepatogenic differentiation of human adipose stem cell-embedded mesh structures. Sci Rep 5:13427.
- Aksu AE, Rubin JP, Dudas JR, Marra KG. 2008. Role of gender and anatomical region on induction of osteogenic differentiation of human adipose-derived stem cells. Ann Plast Surg 60:306–322.
- Alizadeh E, Akbarzadeh A, Eslaminejad MB, Barzegar A, Hashemzadeh S, Nejati‐Koshki K, et al. 2015. Up-regulation of liver-enriched transcription factors HNF4a and HNF6 and liver-specific microRNA (miR-122) by inhibition of let-7b in mesenchymal stem cells. Chem Biol Drug Des 85:268–279.
- Alizadeh E, Eslaminejad MB, Akbarzadeh A, Sadeghi Z, Abasi M, Herizchi R, et al. 2016. Upregulation of MiR-122 via trichostatin A treatments in hepatocyte-like cells derived from mesenchymal stem cells. Chem Biol Drug Des 87:296–305.
- Alizadeh E, Zarghami N, Eslaminejad M, Barzegar A, Jahangi S, Hashemzadeh S. 2013. Epigenetic alteration of Mir-122 and Let-7b expression in adipose tissue-derived mesenchymal stem cells by trichostatin A. Cell J 44:157–164.
- Alizadeh E, Zarghami N, Eslaminejad MB, Hashemzadeh S, Barzegar A, editors. Trichostatin A induced Mir-122 expression in adipose tissue-derived mesenchymal stem cells. 1st Tabriz International Life Science Conference and 12th Iran Biophysical Chemistry Conference; Tabriz University of Medical Sciences, Tabriz, Iran; 2013.
- Allen LT, Tosetto M, Miller IS, O’Connor DP, Penney SC, Lynch I, et al. 2006. Surface-induced changes in protein adsorption and implications for cellular phenotypic responses to surface interaction. Biomaterials 27:3096–3108.
- Antoni D, Burckel H, Josset E, Noel G. 2015. Three-dimensional cell culture: a breakthrough in vivo. Int J Mol Sci 16:5517–5527.
- Baraniak PR. 2012. Scaffold-free culture of mesenchymal stem cell spheroids in suspension preserves multilineage potential. Cell Tissue Res 347:701–711.
- Barnes CP, Sell SA, Boland ED, Simpson DG, Bowlin GL. 2007. Nanofiber technology: designing the next generation of tissue engineering scaffolds. Adv Drug Deliv Rev 59:1413–1433.
- Benavente CA, Sierralta WD, Conget PA, Minguell JJ. 2003. Subcellular distribution and mitogenic effect of basic fibroblast growth factor in mesenchymal uncommitted stem cells. Growth Fact 21:87–94.
- Bonab MM, Alimoghaddam K, Talebian F, Ghaffari SH, Ghavamzadeh A, Nikbin B. 2006. Aging of mesenchymal stem cell in vitro. BMC Cell Biol 7:14.
- Chang KA, Kim JW, Kim JA, Lee SE, Kim S, Suh WH, et al. 2011. Biphasic electrical currents stimulation promotes both proliferation and differentiation of fetal neural stem cells. PLoS One 6:e18738.
- Chen W, Villa-Diaz LG, Sun Y, Weng S, Kim JK, Lam RHW, et al. 2012. Nanotopography influences adhesion, spreading, and self-renewal of human embryonic stem cells. ACS Nano 6:4094–4103.
- Chen XD. 2010. Extracellular matrix provides an optimal niche for the maintenance and propagation of mesenchymal stem cells. Birth Def Res C Embryo Today Rev 90:45–54.
- Cheng N-C, Chen S-Y, Li J-R, Young T-H. 2013. Short-term spheroid formation enhances the regenerative capacity of adipose-derived stem cells by promoting stemness, angiogenesis, and chemotaxis. Stem Cells Transl Med 2:584.
- Crisostomo PR, Markel TA, Wang M, Lahm T, Lillemoe KD, Meldrum DR. 2007. In the adult mesenchymal stem cell population, source gender is a biologically relevant aspect of protective power. Surgery 142:215–221.
- Curran JM, Chen R, Hunt JA. 2006. The guidance of human mesenchymal stem cell differentiation in vitro by controlled modifications to the cell substrate. Biomaterials 27:4783–4793.
- Deasy BM, Schugar RC, Huard J. 2008. Sex differences in muscle-derived stem cells and skeletal muscle. Crit Rev Eukaryotic Gene Express 18:173–188.
- Djouad F, Tejedor G, Toupet K, Maumus M, Bony C, Blangy A, et al. 2014. Promyelocytic leukemia zinc-finger induction signs mesenchymal stem cell commitment: identification of a key marker for stemness maintenance? Stem Cell Res Ther 5:27.
- Engler AJ, Sen S, Sweeney HL, Discher DE. 2006. Matrix elasticity directs stem cell lineage specification. Cell 126:677–689.
- Eom YW, Oh J-E, Lee JI, Baik SK, Rhee K-J, Shin HC, et al. 2014. The role of growth factors in maintenance of stemness in bone marrow-derived mesenchymal stem cells. Biochem Biophys Res Commun 445:16–22.
- Farach-Carson M, Wagner R, Kiick K. 2007. Extracellular Matrix: Structure, Function, and Applications to Tissue Engineering. Boca Raton (FL): CRC Press, pp. 3-1–3-22.
- Gerecht S, Burdick JA, Ferreira LS, Townsend SA, Langer R, Vunjak-Novakovic G. 2007. Hyaluronic acid hydrogel for controlled self-renewal and differentiation of human embryonic stem cells. Proc Natl Acad Sci USA 104:11298–11303.
- Goffin JM, Pittet P, Csucs G, Lussi JW, Meister JJ, Hinz B. 2006. Focal adhesion size controls tension-dependent recruitment of alpha-smooth muscle actin to stress fibers. J Cell Biol 172:259–268.
- Gotoh N. 2008. Regulation of growth factor signaling by FRS2 family docking/scaffold adaptor proteins. Cancer Sci 99:1319–1325.
- Gotoh N. 2009. Control of stemness by fibroblast growth factor signaling in stem cells and cancer stem cells. Curr Stem Cell Res Ther 4:9–15.
- Griffith LG, Swartz MA. 2006. Capturing complex 3D tissue physiology in vitro. Nat Rev Mol Cell Biol 7:211–224.
- Guilak F, Cohen DM, Estes BT, Gimble JM, Liedtke W, Chen CS. 2009. Control of stem cell fate by physical interactions with the extracellular matrix. Cell Stem Cell 5:17–26.
- Han B, Li J, Li Z, Guo L, Wang S, Liu P, et al. 2013. Trichostatin A stabilizes the expression of pluripotent genes in human mesenchymal stem cells during ex vivo expansion. PLoS One 8:e81781
- Han S, Zhao Y, Xiao Z, Han J, Chen B, Chen L, et al. 2012. The three-dimensional collagen scaffold improves the stemness of rat bone marrow mesenchymal stem cells. J Genet Genomics 39:633–641.
- Haragopal H, Yu D, Zeng X, Kim S-W, Han I-B, Ropper AE, et al. 2015. Stemness enhancement of human neural stem cells following bone marrow MSC coculture. Cell Transplant 24:645–659.
- Hassan D, Nosratollah Z. 2014. Trichostatin A-induced Apoptosis is mediated by Kruppel-like factor 4 in ovarian and lung cancer. Asian Pac J Cancer Prev 15:6581–6586.
- Havasi P, Nabioni M, Soleimani M, Bakhshandeh B, Parivar K. 2013. Mesenchymal stem cells as an appropriate feeder layer for prolonged in vitro culture of human induced pluripotent stem cells. Mol Biol Rep 40:3023–3031.
- Haycock JW. 2011. 3D cell culture: a review of current approaches and techniques. 3D Cell Cult Meth Prot 2011:1–15.
- He F, Chen X, Pei M. 2009. Reconstruction of an in vitro tissue-specific microenvironment to rejuvenate synovium-derived stem cells for cartilage tissue engineering. Tissue Eng A 15:3809–3821.
- He W, Yong T, Teo WE, Ma Z, Ramakrishna S. 2005. Fabrication and endothelialization of collagen-blended biodegradable polymer nanofibers: potential vascular graft for blood vessel tissue engineering. Tissue Eng 11:1574–1588.
- Higuchi O, Okabe M, Yoshida T, Fathy M, Saito S, Miyawaki T, et al. 2012. Stemness of human Wharton’s jelly mesenchymal cells is maintained by floating cultivation. Cell Reprogram 14:448–455.
- Hofmeister LH, Costa L, Balikov DA, Crowder SW, Terekhov A, Sung H-J, et al. 2015. Patterned polymer matrix promotes stemness and cell-cell interaction of adult stem cells. J Biol Eng 9:1.
- Hong L, Zhang G, Sultana H, Yu Y, Wei Z. 2011. The effects of 17-β estradiol on enhancing proliferation of human bone marrow mesenchymal stromal cells in vitro. Stem Cells Dev 20:925–931.
- Horwitz EM, Le Blanc K, Dominici M, Mueller I, Slaper-Cortenbach I, Marini FC, et al. 2005. Clarification of the nomenclature for MSC: The International Society for Cellular Therapy position statement. Cytotherapy 7:393–395.
- Hoseinzadeh S, Atashi A, Soleimani M, Alizadeh E, Zarghami N. 2016. MiR-221-inhibited adipose tissue-derived mesenchymal stem cells bioengineered in a nano-hydroxy apatite scaffold. In Vitro Cell Develop Biol Anim 52:479–487.
- Hosseinkhani H, Hiraoka Y, Li C-H, Chen Y-R, Yu D-S, Hong P-D, et al. 2013. Engineering three-dimensional collagen-IKVAV matrix to mimic neural microenvironment. ACS Chem Neurosci 4:1229–1235.
- Hou J, Han ZP, Jing YY, Yang X, Zhang SS, Sun K, et al. 2013. Autophagy prevents irradiation injury and maintains stemness through decreasing ROS generation in mesenchymal stem cells. Cell Death Dis 4:e844.
- Huang G-S, Dai L-G, Yen BL, Hsu S-h. 2011. Spheroid formation of mesenchymal stem cells on chitosan and chitosan-hyaluronan membranes. Biomaterials 32:6929–6945.
- Jahani H, Jalilian FA, Wu CY, Kaviani S, Soleimani M, Abassi N, et al. 2015. Controlled surface morphology and hydrophilicity of polycaprolactone toward selective differentiation of mesenchymal stem cells to neural like cells. J Biomed Mater Res A 103:1875–1881.
- Jeong Y, Mangelsdorf DJ. 2009. Nuclear receptor regulation of stemness and stem cell differentiation. Exp Mol Med 41:525–537.
- Jung JS, Jee MK, Cho HT, Choi JI, Im YB, Kwon OH, et al. 2013. MBD6 is a direct target of Oct4 and controls the stemness and differentiation of adipose tissue-derived stem cells. Cell Mol Life Sci 70:711–728.
- Kador KE, Goldberg JL. 2012. Scaffolds and stem cells: delivery of cell transplants for retinal degenerations. Exp Rev Ophthalmol 7:459–470.
- Kang JX, Wan JB, He C. 2014. Concise review: regulation of stem cell proliferation and differentiation by essential fatty acids and their metabolites. Stem Cells 32:1092–1098.
- Kashyap V, Rezende NC, Scotland KB, Shaffer SM, Persson JL, Gudas LJ, et al. 2009. Regulation of stem cell pluripotency and differentiation involves a mutual regulatory circuit of the NANOG, OCT4, and SOX2 pluripotency transcription factors with polycomb repressive complexes and stem cell microRNAs. Stem Cells Dev 18:1093–1108.
- Kelly KF, Daniel JM. 2006. POZ for effect-POZ-ZF transcription factors in cancer and development. Trends Cell Biol 16:578–587.
- Kolf CM, Cho E, Tuan RS. 2007. Mesenchymal stromal cells. Biology of adult mesenchymal stem cells: regulation of niche, self-renewal and differentiation. Arthritis Res Ther 9:204.
- Kolli S, Ahmad S, Lako M, Figueiredo F. 2010. Successful clinical implementation of corneal epithelial stem cell therapy for treatment of unilateral limbal stem cell deficiency. Stem Cells (Dayton, Ohio) 28:597–610.
- Kretlow JD, Jin Y-Q, Liu W, Zhang WJ, Hong T-H, Zhou G, et al. 2008. Donor age and cell passage affects differentiation potential of murine bone marrow-derived stem cells. BMC Cell Biol 9:60.
- Larson BL, Ylostalo J, Lee RH, Gregory C, Prockop DJ. 2010. Sox11 is expressed in early progenitor human multipotent stromal cells and decreases with extensive expansion of the cells. Tissue Eng A 16:3385–3394.
- Lee S, Yu KR, Ryu YS, Oh YS, Hong IS, Kim HS, et al. 2014. miR-543 and miR-590-3p regulate human mesenchymal stem cell aging via direct targeting of AIMP3/p18. Age (Dordr) 36:9724.
- Lengner CJ, Welstead GG, Jaenisch R. 2008. The pluripotency regulator Oct4: a role in somatic stem cells? Cell Cycle 7:725–728.
- Leychkis Y, Munzer SR, Richardson JL. 2009. What is stemness? Stud Hist Philos Biol Biomed Sci 40:312–320.
- Li Z, Tian X, Yuan Y, Song Z, Zhang L, Wang X, et al. 2013. Effect of cell culture using chitosan membranes on stemness marker genes in mesenchymal stem cells. Mol Med Rep 7:1945–1949.
- Lin SP, Chiu FY, Wang Y, Yen ML, Kao SY, Hung SC. 2014. RB maintains quiescence and prevents premature senescence through upregulation of DNMT1 in mesenchymal stromal cells. Stem Cell Rep 3:975–986.
- Liu Z, Tang Y, Qiu T, Cao X, Clemens TL. 2006. A dishevelled-1/Smad1 interaction couples WNT and bone morphogenetic protein signaling pathways in uncommitted bone marrow stromal cells. J Biol Chem 281:17156–17163.
- Loeser RF. 2002. Integrins and cell signaling in chondrocytes. Biorheology 39:119–124.
- Loges S, Fehse B, Brockmann MA, Lamszus K, Butzal M, Guckenbiehl M, et al. 2004. Identification of the adult human hemangioblast. Stem Cells Dev 13:229–242.
- Majd H, Wipff PJ, Buscemi L, Bueno M, Vonwil D, Quinn TM, et al. 2009. A novel method of dynamic culture surface expansion improves mesenchymal stem cell proliferation and phenotype. Stem Cells 27:200–209.
- Martin I, Wendt D, Heberer M. 2004. The role of bioreactors in tissue engineering. Trends Biotechnol 22:80–86.
- McCrum NG, Buckley C, Bucknall CB. 1997. Principles of Polymer Engineering. Oxford: Oxford University Press.
- McMahon KA, Hiew SY, Hadjur S, Veiga-Fernandes H, Menzel U, Price AJ, et al. 2007. MLL has a critical role in fetal and adult hematopoietic stem cell self-renewal. Cell Stem Cell 1:338–345.
- Mitsui K, Tokuzawa Y, Itoh H, Segawa K, Murakami M, Takahashi K, et al. 2003. The homeoprotein Nanog is required for maintenance of pluripotency in mouse epiblast and ES cells. Cell 113:631–642.
- Mohammadzadeh G, Zarghami N. 2009. Associations between single-nucleotide polymorphisms of the adiponectin gene, serum adiponectin levels and increased risk of type 2 diabetes mellitus in Iranian obese individuals. Scand J Clin Lab Invest 69:764–771.
- Monaghan AP, Grau E, Bock D, Schutz G. 1995. The mouse homolog of the orphan nuclear receptor tailless is expressed in the developing forebrain. Development 121:839–853.
- Muller I, Kordowich S, Holzwarth C, Spano C, Isensee G, Staiber A, et al. 2006. Animal serum-free culture conditions for isolation and expansion of multipotent mesenchymal stromal cells from human BM. Cytotherapy 8:437–444.
- Ng F, Boucher S, Koh S, Sastry KS, Chase L, Lakshmipathy U, et al. 2008. PDGF, TGF-beta, and FGF signaling is important for differentiation and growth of mesenchymal stem cells (MSCs): transcriptional profiling can identify markers and signaling pathways important in differentiation of MSCs into adipogenic, chondrogenic, and osteogenic lineages. Blood 112:295–307.
- Ng LW, Yip SK, Wong HK, Yam GH, Liu YM, Lui WT, et al. 2009. Adipose-derived stem cells from pregnant women show higher proliferation rate unrelated to estrogen. Human Reprod 24:1164–1170.
- Orford KW, Scadden DT. 2008. Deconstructing stem cell self-renewal: genetic insights into cell-cycle regulation. Nat Rev Genet 9:115–128.
- Pampaloni F, Reynaud EG, Stelzer EHK. 2007. The third dimension bridges the gap between cell culture and live tissue. Nat Rev Mol Cell Biol 8:839–845.
- Park Y, Kim JH, Lee SJ, Choi IY, Park SJ, Lee SR, et al. 2011. Human feeder cells can support the undifferentiated growth of human and mouse embryonic stem cells using their own basic fibroblast growth factors. Stem Cells Dev 20:1901–1910.
- Perez LM, Bernal A, de Lucas B, San Martin N, Mastrangelo A, Garcia A, et al. 2015. Altered metabolic and stemness capacity of adipose tissue-derived stem cells from obese mouse and human. PLoS One 10:e0123397.
- Pilehvar-Soltanahmadi Y, Akbarzadeh A, Moazzez-Lalaklo N, Zarghami N. 2015. An update on clinical applications of electrospun nanofibers for skin bioengineering. Artif Cells Nanomed Biotechnol 44:1–15.
- Heller J. 1987. Fundamentals of polymer science, chapter 3. 139–177.
- Potten CS, Loeffler M. 1990. Stem cells: attributes, cycles, spirals, pitfalls and uncertainties. Lessons for and from the crypt. Development 110:1001–1020.
- Prunet-Marcassus B, Cousin B, Caton D, Andre M, Penicaud L, Casteilla L. 2006. From heterogeneity to plasticity in adipose tissues: site-specific differences. Exp Cell Res 312:727–736.
- Requicha JF, Viegas CA, Albuquerque CM, Azevedo JM, Reis RL, Gomes ME. 2012. Effect of anatomical origin and cell passage number on the stemness and osteogenic differentiation potential of canine adipose-derived stem cells. Stem Cell Rev 8:1211–1222.
- Reuss B, und Halbach OB. 2003. Fibroblast growth factors and their receptors in the central nervous system. Cell Tissue Res 313:139–157.
- Rizzino A. 2009. Sox2 and Oct-3/4: a versatile pair of master regulators that orchestrate the self-renewal and pluripotency of embryonic stem cells. Wiley Interdiscip Rev Syst Biol Med 1:228–236.
- Roldan M, Macias-Gonzalez M, Garcia R, Tinahones FJ, Martin M. 2011. Obesity short-circuits stemness gene network in human adipose multipotent stem cells. FASEB J 25:4111–4126.
- Rubin J, Rubin C, Jacobs CR. 2006. Molecular pathways mediating mechanical signaling in bone. Gene 367:1–16.
- Rustad KC, Wong VW, Sorkin M, Glotzbach JP, Major MR, Rajadas J, et al. 2012. Enhancement of mesenchymal stem cell angiogenic capacity and stemness by a biomimetic hydrogel scaffold. Biomaterials 33:80–90.
- Saha K, Mei Y, Reisterer CM, Pyzocha NK, Yang J, Muffat J, et al. 2011. Surface-engineered substrates for improved human pluripotent stem cell culture under fully defined conditions. Proc Natl Acad Sci USA 108:18714–18719.
- Saller MM, Prall WC, Docheva D, Schönitzer V, Popov T, Anz D, et al. 2012. Increased stemness and migration of human mesenchymal stem cells in hypoxia is associated with altered integrin expression. Biochem Biophys Res Commun 423:379–385.
- Schwartz SD, Hubschman JP, Heilwell G, Franco-Cardenas V, Pan CK, Ostrick RM, et al. 2012. Embryonic stem cell trials for macular degeneration: a preliminary report. Lancet (London, England) 379:713–720.
- Sell SA, Wolfe PS, Garg K, McCool JM, Rodriguez IA, Bowlin GL. 2010. The use of natural polymers in tissue engineering: a focus on electrospun extracellular matrix analogues. Polymers 2:522–553.
- Shyh-Chang N, Daley GQ, Cantley LC. 2013. Stem cell metabolism in tissue development and aging. Development 140:2535–2547.
- Siegel G, Kluba T, Hermanutz-Klein U, Bieback K, Northoff H, Schäfer R. 2013. Phenotype, donor age and gender affect function of human bone marrow-derived mesenchymal stromal cells. BMC Med 11:1–20.
- Song Y, Ju Y, Song G, Morita Y. 2013. In vitro proliferation and osteogenic differentiation of mesenchymal stem cells on nanoporous alumina. Int J Nanomedicine 8:2745–2756.
- Soomekh DJ. 2011. Current concepts for the use of platelet-rich plasma in the foot and ankle. Clin Podiatr Med Surg 28:155–170.
- Sun LY, Pang CY, Li DK, Liao CH, Huang WC, Wu CC, et al. 2013. Antioxidants cause rapid expansion of human adipose-derived mesenchymal stem cells via CDK and CDK inhibitor regulation. J Biomed Sci 20:53
- Toda S, Watanabe K, Yokoi F, Matsumura S, Suzuki K, Ootani A, et al. 2002. A new organotypic culture of thyroid tissue maintains three-dimensional follicles with C cells for a long term. Biochem Biophys Res Commun 294:906–911.
- Tormin A, Brune JC, Olsson E, Valcich J, Neuman U, Olofsson T, et al. 2009. Characterization of bone marrow-derived mesenchymal stromal cells (MSC) based on gene expression profiling of functionally defined MSC subsets. Cytotherapy 11:114–128.
- Tsimbouri PM, McMurray RJ, Burgess KV, Alakpa EV, Reynolds PM, Murawski K, et al. 2012. Using nanotopography and metabolomics to identify biochemical effectors of multipotency. ACS Nano 6:10239–10249.
- Tsutsumi S, Shimazu A, Miyazaki K, Pan H, Koike C, Yoshida E, et al. 2001. Retention of multilineage differentiation potential of mesenchymal cells during proliferation in response to FGF. Biochem Biophys Res Commun 288:413–419.
- Unsworth BR, Lelkes PI. 1998. Growing tissues in microgravity. Nat Med 4:901–907.
- van Staa TP, Geusens P, Pols HA, de Laet C, Leufkens HG, Cooper C. 2005. A simple score for estimating the long-term risk of fracture in patients using oral glucocorticoids. QJM 98:191–198.
- Watabe T, Miyazono K. 2009. Roles of TGF-beta family signaling in stem cell renewal and differentiation. Cell Res 19:103–115.
- Wei C, Liu X, Tao J, Wu R, Zhang P, Bian Y, et al. 2014. Effects of vitamin C on characteristics retaining of in vitro-cultured mouse adipose-derived stem cells. In Vitro Cell Dev Biol Anim 50:75–86.
- Wei F, Qu C, Song T, Ding G, Fan Z, Liu D, et al. 2012. Vitamin C treatment promotes mesenchymal stem cell sheet formation and tissue regeneration by elevating telomerase activity. J Cell Physiol 227:3216–3224.
- Xu X, Duan S, Yi F, Ocampo A, Liu GH, Izpisua Belmonte JC. 2013. Mitochondrial regulation in pluripotent stem cells. Cell Metab 18:325–332.
- Yan XZ, van den Beucken JJ, Both SK, Yang PS, Jansen JA, Yang F. 2014. Biomaterial strategies for stem cell maintenance during in vitro expansion. Tissue Eng B Rev 20:340–354.
- Yang T-L, Young T-H. 2009. The specificity of chitosan in promoting branching morphogenesis of progenitor salivary tissue. Biochem Biophys Res Commun 381:466–470.
- Ye L, Chen L, Yu Q, Cheng F. 2010. Effect of recombinant human erythropoietin on the stemness of bone marrow-derived mesenchymal stem cells in vitro. Int J Stem Cells 3:175–182.
- Yew TL, Chiu FY, Tsai CC, Chen HL, Lee WP, Chen YJ, et al. 2011. Knockdown of p21(Cip1/Waf1) enhances proliferation, the expression of stemness markers, and osteogenic potential in human mesenchymal stem cells. Aging Cell 10:349–361.
- Yi F, Pereira L, Hoffman JA, Shy BR, Yuen CM, Liu DR, et al. 2011. Opposing effects of Tcf3 and Tcf1 control Wnt stimulation of embryonic stem cell self-renewal. Nat Cell Biol 13:762–770.
- Yoon H-H, Han M-J, Park J-K, Lee J-H, Seo Y-K. 2015. Effect of low temperature on Schwann-like cell differentiation of bone marrow mesenchymal stem cells. Tissue Eng Regen Med 12:259–267.
- Yu J, Tu Y-K, Tang Y-B, Cheng N-C. 2014. Stemness and transdifferentiation of adipose-derived stem cells using l-ascorbic acid 2-phosphate-induced cell sheet formation. Biomaterials 35:3516–3526.
- Yu KR, Yang SR, Jung JW, Kim H, Ko K, Han DW, et al. 2012. CD49f enhances multipotency and maintains stemness through the direct regulation of OCT4 and SOX2. Stem Cells 30:876–887.
- Yu Y, Wei N, Stanford C, Schmidt T, Hong L. 2012. In vitro effects of RU486 on proliferation and differentiation capabilities of human bone marrow mesenchymal stromal cells. Steroids 77:132–137.
- Zachar V, Duroux M, Emmersen J, Rasmussen JG, Pennisi CP, Yang S, et al. 2011. Hypoxia and adipose-derived stem cell-based tissue regeneration and engineering. Exp Opin Biol Ther 11:775–786.
- Zare M, Soleimani M, Akbarzadeh A, Bakhshandeh B, Aghaee‐Bakhtiari SH, Zarghami N. 2015. A novel protocol to differentiate induced pluripotent stem cells by neuronal microRNAs to provide a suitable cellular model. Chem Biol Drug Des 86:232–238.
- Zare M, Soleimani M, Mohammadian M, Akbarzadeh A, Havasi P, Zarghami N. 2016. Efficient biotechnological approach for lentiviral transduction of induced pluripotent stem cells. Artif Cells Nanomed Biotechnol 44:743–748.
- Zarghami N, Onsori H, Alani B. 2005. Association between steroid hormone receptors and PSA gene expression in breast cancer cell lines. Afr J Biotechnol 4:1415–1420.
- Zeng YA, Nusse R. 2010. Wnt proteins are self-renewal factors for mammary stem cells and promote their long-term expansion in culture. Cell Stem Cell 6:568–577.
- Zhang D, Kilian KA. 2013. The effect of mesenchymal stem cell shape on the maintenance of multipotency. Biomaterials 34:3962–3969.
- Zhang S, Liu P, Chen L, Wang Y, Wang Z, Zhang B. 2015. The effects of spheroid formation of adipose-derived stem cells in a microgravity bioreactor on stemness properties and therapeutic potential. Biomaterials 41:15–25.
- Zhu N, Wang H, Wang B, Wei J, Shan W, Feng J, et al. 2016. A member of the nuclear receptor superfamily, designated as NR2F2, supports the self-renewal capacity and pluripotency of human bone marrow-derived mesenchymal stem cells. Stem Cells Int 2016:5687589.