Abstract
A study was conducted to compare the resistance to heme exposure between myoglobin, bovine hemoglobin, and OxyVita®Hb (zero-link-hemoglobin-polymer). The rate of release of heme-iron is related to the tissue oxidative stress that can elicit deleterious effects in clinical uses of blood substitutes. Experimental work has focused on the unfolding of hemoglobin molecules, resulting in heme loss, by using urea as a denaturant and analyzing the Soret spectral region. These unfolding studies provide evidence for both the structural integrity and redox stability of OxyVita®Hb and demonstrate that OxyVita®Hb does not readily unfold and its heme exposure/release is greatly reduced.
Summary
Revolutionizing blood science is a developing area in research today. OxyVita®Hb is a zero-linked, polymerized, stroma free hemoglobin, which is a promising clinical blood substitute due to its ability to deliver oxygen (Bucci et al. Citation1996, Harrington and Wollocko Citation2011, Song et al. Citation2013). A study was conducted to assess and compare the resistance to heme exposure between myoglobin (monomer), bovine hemoglobin (tetramer), and OxyVita®Hb (polymer – 2 forms: OxyVita®Hb and OxyVita®C HbCO) (Harrington et al. Citation2010, Harrington et al. Citation2009, Schaer et al. Citation2013). This information is significant due to the fact that the rate of the release of heme-iron is related to the cellular and tissue oxidative stress events that can elicit deleterious side effects in clinical uses of blood substitutes (Alayash and Cashon Citation1995, Harrington et al. Citation2010, Harrington et al. Citation2011, Jia and Alayash Citation2009).
Experimental work has focused on the unfolding of hemoglobin molecules with increasing concentrations of urea as a denaturant. It is known that hemoglobin denaturation with chaotropic agents such as urea leads to molecular unfolding and heme loss (Alayash and Cashon Citation1995, Harrington et al. Citation2011, Jia and Alayash Citation2009). Therefore, monitoring heme exposure is useful tool in determining protein unfolding. The extent of heme exposure was determined by measuring the absorbance of three types of heme proteins, bovine tetrameric hemoglobin, myoglobin, and zero-linked polymeric hemoglobin (OxyVita®Hb) manufactured by OXYVITA, Inc. Resistance to molecular unfolding was determined through analysis of the Soret spectral region (350–450 nm) due to its absorbance sensitivity to alterations within the heme environment. When heme proteins are exposed to a conformational solvent perturbant, such as urea, disruption of the many intramolecular interactions responsible for the maintenance of the native structure occurs (Harrington and Wollocko Citation2010, Song et al. Citation2013, Harrington et al. Citation2011, Harrington et al. Citation2010). Absorbances were recorded at increasing concentrations of urea while keeping the concentration of hemoglobin constant. The results provide data for comparison of the three different hemoglobin molecules examined. These unfolding studies provide evidence for both the structural integrity and redox stability of OxyVita®Hb that are required for normal hemoglobin functionality, i.e. maintenance of the heme-iron in the reduced state necessary for the reversible binding/release of molecular oxygen and minimization of methemoglobin (heme-Fe+3) formation. Further, we demonstrate that the zero-linked polymeric hemoglobin does not readily unfold and the extent of its heme exposure or release is greatly reduced.
Introduction
The physiological binding and release of molecular oxygen from any acellular hemoglobin acting as a Hemoglobin-Based-Oxygen-Carrier (HBOC) requires that the heme-iron be maintained in the reduced state (heme-Fe+2). Unfortunately, all acellular HBOCs are susceptible to methemoglobin formation to different degrees depending upon their inherent structure and redox potential (Alayash and Cashon Citation1995, Faivre et al. Citation1998, Harrington et al. Citation2011, Jia and Alayash Citation2009, Harrington et al. Citation2010). Methemoglobin (heme-Fe+3), which can be generated either through autoxidation and/or interaction with exogenous oxidizing compounds, is unable to bind oxygen. Lacking the intracellular, protective, antioxidative enzymes such as superoxide dismutase, catalase and low molecular weight endogenous reductants like ascorbate and gluthatione, acellular HBOCs undergo oxidation beginning with methemoglobin formation, slowly progressing to hemichrome formation, followed by progression to the ferryl heme-Fe+4 intermediate in the presence of H2O2, and ultimately free heme and release of iron. This sequence of reactions can initiate and/or propagate a wide range of oxidative events within the vascular system involving interactions with lipids, proteins and nucleic acids () (Alayash and Cashon Citation1995, Harrington et al. Citation2011, Jia and Alayash Citation2009).
Figure 1. Unfolding spectra in the Soret region of reduced, HbCO, and oxidized, metHb, forms of OxyVita®Hbs in presence of increasing concentrations of urea at T = 37 °C, pH 7.35.
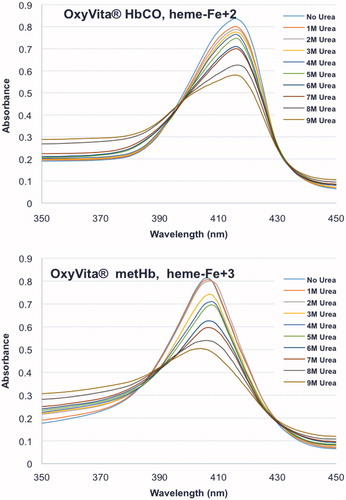
The unique structural array within the heme-iron pocket that is responsible for the affinity of the globins for the heme moiety is regulated by a network of covalent, hydrophophic, electrostatic, and steric effects between the bound heme and the globin. The covalent bond between the proximal His92 (Bovine Hb) and the fifth coordination site of the reduced iron is vital in binding the heme to the reduced iron. Autoxidation leads to the weakening of this bond, and sets in motion a series of unfolding events that result in increased heme exposure and ultimately dissociation from the methemoglobin species. Alterations of many non-covalent interactions come into play as well during the unfolding process. In particular, hydrophobic interactions associated with the methyl and vinyl groups on the tetrapyrrole ring and the apolar region of the globin also contribute to the retention of the heme moiety (Harrington and Wollocko Citation2013, Harrington et al. Citation2009, Schaer et al. Citation2013).
These molecular events, associated with initiation of oxidative stress, have been well documented through years of pre-clinical and clinical studies with HBOC’s (Alayash and Cashon Citation1995, Harrington et al. Citation2011, Jia and Alayash Citation2009). For many of the earlier generation HBOCs, significant evidence has linked exposure to these acellular Hbs to toxicity in the vasculature and major organs including the heart, kidney, lung, liver, and brain. The extent of this toxicity has been associated with the duration of exposure within the circulatory system, dosage, the presence and extent of endogenous antioxidant and detoxification systems, and pre-existing pathophysiology, such as inflammation and ischemia (Alayash and Cashon Citation1995, Harrington et al. Citation2011, Jia and Alayash Citation2009).
Unique among HBOCs being produced today is the large polymeric OxyVita®Hb which is synthesized through a series of chemical activators. This process leads to a homogeneous globular-like polymeric molecule (M.wt. ∼17MDa) that is structurally designed to have the neighboring bovine Hb tetramers linked by amide bonds (pseudopeptide bonds). Thus, no chemical linking agents are present in the final polymer (Bucci et al. Citation1996).
To determine the resistance of OxyVita®Hb to heme exposure during possible methemoglobin formation within the circulation, a series of unfolding studies were carried out in vitro in the presence of increasing concentrations of urea. We compared the met forms of the single-chain myoglobin, tetrameric bovine hemoglobin, and the polymeric OxyVita®Hb. Resistance to molecular unfolding, heme exposure and release was determined through analysis of the Soret spectral region (350–450 nm) wherein absorbance and wavelength maximum sensitivity are linked to alterations within the heme environment. When heme proteins are exposed to a conformational solvent perturbant, such as urea, specifically at large/increasing concentrations, disruption of the native protein structure occurs. These unfolding studies provide evidence for both the structural integrity and redox stability of OxyVita®Hb. Based upon the minimal heme exposure and release we suggest OxyVita®Hb has the potential to mitigate possible oxidative vascular stress and damage.
Experimental methods
Preparation of zero-linked polymeric hemoglobin (OxyVita®Hb)
Fresh bovine blood was obtained from a USDA-approved facility, with the appropriate documentation on the history of the herd. The purification of bovine hemoglobin was carried out through a process of red cell lysis using a dilute phosphate buffer, pH 7.4, followed by a series of low-speed and high-speed centrifugation to remove cellular debris. The isolated hemoglobin was used immediately for the production of the polymerized OxyVita®hemoglobin.
Initiation of the zero-linked polymerization process is governed by the use of chemical activators that facilitate intermolecular polymerization. In the production of OxyVita®Hb, the water soluble carbodiimide EDC [1-ethyl-3-(3-dimethylaminopropyl) carbodiimide] is used to activate side chain carboxylate groups on the hemoglobin surface, producing a highly reactive and short-lived O-acyliosurea derivative. The complex is formed from the C-terminal and Glu and Asp side chain carboxylate groups. These activated species react with the side chains of lysyl residues on adjacent hemoglobin molecules to form a stable amide bond (covalent), often referred to as a pseudopeptide bond. The isourea by-product is soluble in an aqueous solution and is removed directly by repeated dialysis. Some interference can occur during this activation process wherein the activated carboxylic groups may be hydrolyzed by water, reducing their reactivity with available lysyl amino groups. To improve on the efficiency of the polymerization process, a two-step approach to enhance the yield of the amide bond formation was introduced. Addition of N-hydroxysulfosuccinimide (sulfo-NHS) to the carbodiimide reaction resulted in the formation of an intermediate sulfo-NHS ester, which then reacts with the amino groups. One advantage of this approach is to achieve modulation of the extent of polymerization by altering the relative amounts of sulfo-NHS and EDC within the reaction mixture. Size exclusion chromatography (SEC) was used to remove lower molecular weight components and was coupled with dynamic light scattering (DLS) to improve and verify molecular weight homogeneity (∼17 MDa) (Schaer et al. Citation2013).
Structural stability: isothermal unfolding studies
The isothermal unfolding of each heme protein was obtained at 37 °C. Urea solutions (Sigma Ultrapure, – Sigma-Aldrich Corp St. Louis, MO) of 1.0–9.0 M were prepared by volumetric dilution using a 10 M stock solution. For absorbance determinations, hemoglobin solutions were prepared by dilution in 5 ml volumetric flasks. All protein solutions contained 100 mM Tris-HCl, 100 mM KCl, pH 7.35. Spectral measurements in the Soret region (450–350 nm) were recorded on a Shimadzu 160 recording spectrophotometer after 30 min of equilibration at 37 °C in an overlay mode (see and ). The unfolding of each heme protein as a function of increasing urea concentration was followed by changes in absorbance spectra within the Soret region (450–350 nm). Hemoglobin concentrations were ∼10−6 M in heme. The Soret region wavelength maximum for the native (absence of urea) heme proteins are: metmyoglobin (metMb), 410 nm; bovine metHb, 406 nm; and OxyVita®metHb, 406 nm.
Preparation of methemoglobins
Each heme protein was treated with a small molar excess of potassium ferricyanide, K3Fe(CN)6. Ferricyanide is a strong oxidizing agent that has been successfully used with a variety of hemoglobin’s. After adding K3Fe(CN)6 the sample was allowed to equilibrate for 10 min at room temperature before it was filtered through a Sephadex G-25 column to separate the unreactive ferricyanide from the met form of the proteins. Spectra (700 nm–490 nm) were run to confirm complete conversion to the met form for each heme protein.
Size exclusion chromatography (SEC)
Molecular weight homogeneity was determined with a Hewlett-Packard HPLC 1100 system (Agilent Technologies Hewlett-Packard ? Alpharetta, GA) using a 60 cm ×0.5 cm glass column packed with Fractogel (20–40 μm) at flow rate of 0.5 ml/min and wavelength of 280 nm. The mobile phase was 0.05 M phosphate buffer at pH 7.4. Agilent ChemStation software (Agilent Technologies Hewlett-Packard ? Alpharetta, GA) was used for collection of data and analysis.
Results
The unfolding spectra of OxyVita®C HbCO (heme-Fe + 2) and OxyVita®metHb (heme-Fe + 3) for the Soret (450–350 nm) region in the presence of increasing concentrations (0 M–9 M) of urea are shown in . The wavelength maxima of the native reduced form is 417 nm (top) and the oxidized form is 406 nm (bottom). As the concentration of urea increases, there is a decrease in the absorbance signal, but no alteration of the wavelength maxima for either the reduced or oxidized forms of the OxyVita®Hbs. This unfolding behavior clearly indicates that some perturbation of the secondary and tertiary structure of these polymeric Hbs does occur with increasing concentrations of urea. However, as one examines the wavelength maxima for each form of Hb, there is no corresponding blue shift of these wavelength maxima indicating no significant heme exposure or release from the globin chains even in the presence of high concentrations of urea (9 M). Heme exposure as determined by Soret spectral analysis is evident by a broad spectral band and wavelength maximum blue shift towards 395 nm comparable to that of free heme.
While the metHb form OxyVita®Hb does indicate some alterations of its secondary and tertiary structure compared to the native (no urea) form with increasing urea concentration (, bottom panel), the oxidized met form is not associated with any molecular dissociation of this oxidized form (). The symmetry of the SEC peak is preserved. Thus, the molecular size and structural integrity of the OxyVita®metHb is unchanged.
A comparison of the unfolding behavior of metmyoglobin (metMb), bovine tetrameric Hb (metBvHb) and OxyVita®metHb as presented in and . The large differences in the unfolding Soret spectra of metMb and metBvHb compared to polymeric Hb as the urea concentration increases shows not only a large decrease in absorbance, but a significant blue shift in the wavelength maxima of the metMb (410 nm→397 nm) and metBvHb (406 nm→396 nm). The blue shifts for metMb and metBvHb are directly related to an increase in the extent of heme exposure as the linkage between the heme moiety and the globin chains weaken due to the greater interaction of urea with the intact molecules. This is in marked contrast to the behavior of OxyVita®metHb which clearly demonstrates smaller absorbance changes and no significant blue shift in the Soret wavelength maxima (406 nm → 404 nm) with increasing urea concentration. These results strongly suggest that the structural nature of the zero-linked polymeric OxyVita®Hbs provides greater resistance to molecular unfolding and reduces the extent of heme exposure and heme release in the met form.
Figure 4. Unfolding transitions in the Soret wavelength maximum of the oxidized met forms of Mb, BvHb, OxyVita®Hb, and the reduced (CO) form of OxyVita®Hb in the presence of increasing concentrations of urea at T = 37 °C, pH 7.35.
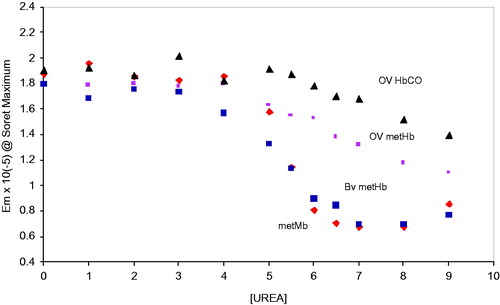
In the full unfolding profiles based on the decrease in the extinction coefficients of metMb, metBvHb, and the reduced and oxidized forms of OxyVita®Hbs in the presence of increasing concentrations of urea are presented. Assuming a simple two-state unfolding mechanism (N ↔ U), a comparison of the unfolding midpoints (D1/2) for each of these proteins is easily obtained from each of the transition profiles evident in . Both the monomeric metMb (D1/2 = 5.4 M urea) and the tetrameric metBvHb (D1/2 = 5.4 M urea) exhibit less resistance to unfolding than either forms of OxyVita®Hb (D1/2 = 6.3 M urea for the met form and D1/2 = 6.9 M urea for the oxy and CO forms). One also sees a greater resistance to unfolding for the reduced OxyVita®HbCO compared to its oxidized form. A summary of these results is presented in .
Figure 5. Comparison of the changes in the Soret wavelength maximum for the met forms of Mb, BvHb, and OxyVita®Hb in the presence of increasing concentrations of urea at T = 37 °C, pH 7.35.
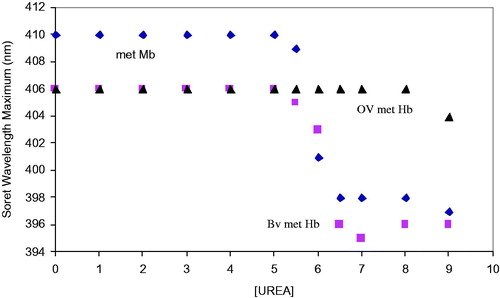
Table 1. Denaturation midpoint (D1/2) at T = 37 °C in presence of urea.
A related series of transition profiles based upon the changes in the Soret wavelength maxima associated with metMb, metBvHb, and OxyVita®metHb demonstrate a large resistance of the zero-linked polymeric OxyVita®Hb to molecular unfolding, reduced heme exposure and release of heme-iron (). The results of this Soret spectral analysis provide direct evidence that the unique structural construct of OxyVita®Hb (zero-linked polymeric hemoglobin) has the potential to reduce oxidative stress within the vascular system due to its greater resistance to molecular unfolding and reduced tendency to expose and release heme-iron into the circulatory system.
Discussion
Over the last several years much effort has gone into understanding the different intravascular mechanisms for reducing oxidative stress associated with the presence of acellular hemoglobin’s. These efforts have largely focused on the removal of hemoglobin that has been released from lysis of red blood cells due to natural aging process or various pathologic processes in an array of different diseases.
A similar problem exists in the therapeutic use of HBOCs, where the oxidative reactions at the heme group may be responsible for cellular, and tissue damage. The most commonly proposed remedy for this issue involves supplementation with scavengers of natural hemoglobin, namely haptoglobin. Research on this subject is more and more active (Harrington et al. Citation2009).
Here we propose a different approach for minimizing oxidative stress that may be associated with acellular HBOCs. Our approach involves fabrication of an HBOC with a structure that exhibits strong resistance to molecular unfolding and heme release. This increased HBOC stability will likely preclude the Fenton reactions that are associated with exposed heme and free iron, thereby limiting oxidative damage within the circulation.
The polymeric, globular structure of OxyVita®Hb, with intra-molecular and inter-molecular pseudo-peptide bonds, exhibits high resistance to molecular unfolding and heme release, even in the presence of very high (non-physiological) concentrations of unfolding compounds. In comparison studies, monomeric myoglobin (Mb) exhibits rapid molecular unfolding, which is expressed by its low denaturation midpoint (the lowest D1/2 amongst hemoglobins compared in this work) and significant heme exposure, shown as a change in the UV spectra in the Soret region from 406 nm to 397 nm. Similarly, bovine Hb (α2β2 tetramer) easily undergoes subunit dissociation with significant unfolding and heme exposure, expressed as a change in UV spectra in Soret region from 406 nm to 396 nm. The denaturation midpoint for bovine Hb is at the same value as myoglobin (D1/2 at 5.4M urea). Our data illustrates the dramatic chaotropic unfolding properties of both myoglobin and bovine Hb. The large differences in wavelength maxima and large decrease in absorbencies in the presence of increasing concentrations of urea demonstrate the extent of heme exposure due to unfolding. In contrast, OxyVita®Hb, exhibits smaller change in absorbencies and no significant blue shift in Soret wavelength maxima when treated with equivalent concentrations of urea. The minimal change in the Soret region from 406 nm to 404 nm is exhibited by zero-linked OxyVita®Hb (HbCO, HbO2, and MetHb) in either the reduced or oxidized forms. The denaturation midpoints for OxyVita®Hb at much higher concentration of urea (between 6.3 and 6.9 M), compared to myoglobin or bovine Hb demonstrate the increased stability of the zero-linked polymeric HBOC. It is also noteworthy that the SEC analysis () indicates that the change from OxyVita®Hb to its met form (reduced to oxidize) does not result in any molecular dissociation of the polymer. Again, this is reflecting the inherent stability of the OxyVita®Hb molecule.
Overall, OxyVita®Hb demonstrates strikingly different behavior when compared to myoglobin or bovine Hb, when exposed to the unfolding agents. This behavior is identical in both, O2 and CO forms of the product as well as for its metHb form. No or little change in the Soret Maxima, small decreases in absorbance signal and a high value of unfolding midpoints demonstrate the structural integrity and strong resistance to molecular unfolding. The stability of OxyVita®Hb can be attributed to the unique molecular structure achieved as a result of the zero-link polymerization production technology which involves a series of activation steps that leads to the formation of pseudopeptide bonds between each tetrameric molecule. This chemical linkage not only provides OxyVita®Hb with structural integrity, but also limits any conformational changes that might lead to rapid heme exposure or release, thus limiting its participation in oxidative events within the circulatory system. Based upon these data we suggest that OxyVita®Hb offers the potential to minimize oxidative damage during therapeutic employment of HBOCs.
References
- Alayash AI, Cashon RE. 1995. Hemoglobin and free radicals: implications for the development of a safe blood substitute. Mol Med Today. 1:122–127.
- Bucci E, Razynska A, Kwansa H, Mathenson-Urbaitis B, O’Hearne M, Ulatowski JA, Koehler RC. 1996. Production and characteristics of an infusible oxygen-carrying fluid based on hemoglobin intramolecularly cross-linked with sebacic acid. J Lab Clin Med. 128:146–153.
- Faivre B, Menu P, Labrude P, Vigernon C. 1998. Hemoglobin autooxidation/oxidation mechanisms and methemoglobin prevention or reduction processes in the bloodstream. Literature review and outline of autooxidation reaction. Artif Cells Blood Substit Immobil Biotechnol. 26:17–26.
- Harrington JP, Orlik K, Wollocko H. 2009. Hierarchical structures of natural acellular polymeric hemoglobins: model for design of a therapeutic hemoglobin-based-oxygen-carrier (HBOC). Biophys J. 96:p437a.
- Harrington JP, Orlik K, Zito SL, Wollocko J, Wollocko H. 2010. Structural and redox behavior of OxyVita®, a zero-linked polymeric hemoglobin: comparison with natural acellular polymeric hemoglobins. Artif Cells Blood Substit Immobil Biotechnol. 38:64–68.
- Harrington JP, Wollocko H. 2010. Pre-clinical studies using OxyVita hemoglobin, a zero-linked polymeric hemoglobin: a review. J Artif Organs. 13:183–188.
- Harrington JP, Wollocko H. 2011. Molecular design properties of OxyVita hemoglobin, a new generation therapeutic oxygen carrier: a review. J Funct Biomaterial. 2:414–424.
- Harrington JP, Wollocko H. 2013. Zero-Link Hemoglobin (OxyVita®): impact of molecular design characteristics on pre-clinical studies, Chapter 15. In Hemoglobin-based oxygen carriers as red cell substitutes and oxygen therapeutics. New York: Springer.
- Harrington JP, Wollocko J, Kostecki E, Wollocko H. 2011. Physicochemical characteristics of OxyVita hemoglobin, a zero-linked polymer: liquid and powder preparations. Artif Cells Blood Substit Immobil Biotechnol. 39:12–18.
- Jia Y, Alayash A.I. 2009. Effects of cross-linking and zero-link polymerization on oxygen transport and redox chemistry of bovine hemoglobin. Biochim Biophys Acta. 1794:1234–1242.
- Schaer DJ, Buehler PW, Alayash AI, Belcher JD, Vercellotti GM. 2013. Hemolysis and free hemoglobin revisited: exploring hemoglobin and hemin scavengers as a novel class of therapeutic proteins. Blood. 121:1276–1284.
- Song BK, Nugent WH, Moon-Massat PF, Auker CR, McCarron R, Pittman RN. 2013. Effects of top-loading a zero-link bovine hemoglobin (ZL-HbBv), OxyVitaTM, on systemic and microcirculatory variables. Military Med. 178:570–577.