Abstract
Issues associated with tissue transplantation and shortage of donors has always been a concern, whereas tissue engineering has provided the hopeful opportunities. The aim of this study was to investigate the chondrogenic differentiation potential of mesenchymal stem cells (MSCs) in the presence of embedded Dexamethasone into electrospun Poly(ethyleneoxide) nanofibers composited with Poly(ɛ-caprolactone) nanofibers. Electrospun-fabricated scaffolds were characterized by SEM, tensile, contact angle, release profile, MTT assay, and chonderogenic differentiation of stem cells. Results demonstrated Poly(ɛ-caprolactone) properties improved by composite with a Poly(ethyleneoxide). Overall, stem cells seeded on nanofibers content drug showed highest chondrogenic potential in comparison to the other groups.
Introduction
Cartilage atrophy and defects due to congenital abnormalities, trauma, or erosion in old age leads to many clinical results (Buckwalter Citation2003, Pirttiniemi et al. Citation2009). The limited improvement potential is reduced in cartilage tissue due to lack of blood vessels and eventually self-reconstruction possibility of cartilage tissues greatly reduced. There are several ways for the treatment of chondral lesions, including cartilage graft (Bhosale and Richardson Citation2008, Sgaglione et al. Citation2002). The tissue is transplanted from the donor to the recipient. The disadvantage of this type of transplantation is the lack of donors and also need to consume a large amount of immune suppressant drugs to prevent rejection of transplanted tissue. In auto-graft foundation treatment is at the same location that use the patient’s own tissue. Problems are treating the cartilage tissue auto-graft procedures, reshape, and define the edges of the cartilage because the shape of a piece of cartilage can cause releasing of imputes forces that balanced in normal cartilage and eventually leads to cartilage deformity.
Tissue engineering that is a recently growing field of medical research is another way for this kind of treatment (Temenoff and Mikos Citation2000). This procedure is fully in accordance with patient needs and it will not have the recipient’s immune system problem in the one tissue recipient. Tissue engineering is a process that creates three-dimensional tissue scaffolds using a combination of appropriate cells and these pay attention to facilitate cell growth and differentiation factors (Yang et al. Citation2001, Citation2002). Mesenchymal stem cells (MSCs) that derived from adipose tissue in appropriate culture conditions and the presence of differentiation factors have the ability to differentiate into cartilage (Shafiee et al. Citation2015). Dexamethasone (Dex) is a long-acting glucocorticoid, and is used widely for the induction of osteogenic, adipogenic, and chondrogenic differentiation but in different doses (Ng et al. Citation2008). While the concentration of Dex for chondrogenic differentiation induction is critical and very less than osteogenic and adipogenic differentiation and also chondrogenic differentiation of MSCs may be inhibited by high concentration of Dex. Therefore, the given and constant presence of Dex can increase the efficiency of chondrogenic differentiation and transplantation therapeutic value to improve the healing of cartilage defects. However, the design and fabrication of adapted Dex delivery construct capable of controlling its release represents a valuable strategy to enhance MSC chondrogenesis. One of the best constructs for this aim is a solid nanofibrous scaffold that can release Dex with good biocompatibility, biodegradability, and nanostructure for stimulating proliferation of stem cells by itself.
Poly (ɛ-caprolactone) (PCL) nanofibers was selected as a main substrate and Poly(ethylene oxide) (PEO) was used for dispersion of Dex to improve bioavailability of them and also increase biocompatibility of PCL. Several studies also showed that the use of conductive polymers including PEO in combination with other synthetic polymers such as PCL (Rath et al. Citation2008), PLLA (Cellesi Citation2012), and PLGA (Patel and Dunn Citation2015) can improve chondrogenic differentiation potential of stem cells as PEO is a water-soluble polymer and can be dissolved in culture media and release Dex. The aim of this study is to investigate the influence of Dex incorporation in nanofibrous PCL-PEO scaffold (PEO/Dex-PCL) fabricated by electrospinning on the chondrogenic differentiation of MSCs in comparison to the chondrogenic differentiation media content Dex.
Materials and methods
Electrospinning
For making scaffolds used in this study, 7% (w/w) solution of Polyethylene oxide (PEO) containing 6 M Dex, and 6% (w/w) solution of Poly-ɛ-caprolacton (PCL) were electrospun by different nozzles in electrospinning device (Nano-Model, Iran). Flow rate and voltage were adjusted at 0.5 ml/h and 23 kV for both polymers, respectively. Also, the distance between nozzle and collector was 24 and 15 cm and the nozzle angle were selected at 25° and 45° for PEO/Dex and PCL solutions, respectively. After mounting the scaffold with a diameter of 21 cm, scaffolds were punched and placed in 24-well plates. It was then followed by sterilization by incubating with 70% of alcohol for 2 h. Then, 4 × 104 MSCs in 24-well plates were counted.
Mechanical characterization
Mechanical characterization of the PEO/Dex-PCL hybrid nanofiber samples were done through tensile test on an Instron universal testing machine (Model STM-20, SANTAM, Iran), with sample sizes of 60 mm × 10 mm and a loading velocity of 50 mm/min. The results are based on four samples obtained from different electrospun sheets. The machine recording data were utilized to plot the tensile stress–strain curves.
Scanning electron microscopy (SEM)
Stem cell-seeded scaffolds were fixed in 2.5% glutaraldehyde for 2 h on days 7 and 21, then washed with PB and dehydrated through a graded series of ethanol (10 min for every section, 50%, 60%, 70%, 80%, 90%, and 100%), vacuum dried, mounted on aluminum stubs, and sputter coated with gold. The samples were examined using a scanning electron microscope (S-4500; Hitachi, Tokyo, Japan) at an accelerating voltage of 20 KV.
Contact angle measurement
To study the hydrophilicity of the nanofiber surface followed by surface treatment, the water contact angle was done by the sessile drop procedure with a G10 Kruss contact angle goniometer at room temperature. A water droplet was placed on the scaffold surface and the contact angle was evaluated after 10 s.
Dexamethasone release
PEO/Dex-PCL nanofibrous meshes (with 4 cm2) were weighted and incubated at 37 °C in 10 ml of phosphate buffer solution (PBS) stirred at 50 rpm. Aliquots of 0.5 ml were retrieved in specified time intervals and the same volume of fresh PBS was added to the medium. The samples were analyzed by spectroscopy at 242 nm (Shimazu UV 1601). The Dex concentration of each sample was calculated using a standard curve (concentrations ranging from 0.0 to 72 mg/ml). The results presented are an average of three replicates. Calculations of the amount of drug released took into account the replacement of medium with fresh medium. Unloaded control PEO-PCL nanofiber meshes were assumed as blanks in the analysis.
Cell culture
Abdominal fat tissues were collected during abdominoplasty procedures from three donors (mean age 30 years, Razi Clinic, Tehran, Iran) with informed consent, according to the Medical Ethics Committee guidelines, Ministry of Health I.R.Iran. MSCs were extracted from adipose tissue, according to the previously reported method (Ardeshirylajimi et al. Citation2014). In brief, fat samples were transferred and washed several times with phosphate-buffered saline (PBS) content antibiotics, then incubated by 0.2% collagenase for an hour at a temperature of 37 °C. After that samples were centrifuged at 1800 rpm for 10 min and then transferred to the flask. After the cell density reached 80%, it was then detached using trypsin and were subcultured. Specific MSC markers were investigated after two passages with flow cytometry. Four groups were used for MSCs culture on 24-well plates: PEO/Dex-PCL under differentiation media (DMEM high glucose supplemented with 10% fetal bovine serum (FBS), 50 μg/L ascorbic acid and 10 μg/ml TGFβ) (PEO/Dex-PCL-DM), PEO/Dex-PCL under basal media (DMEM high glucose supplemented with 10% FBS) (PEO/Dex-PCL-BM), PEO/PCL under differentiation media (PEO/PCL-DM) and cell pellet was also maintained in the tissue culture polystyrene (TCPS) under differentiation media (TCPS-DM) as a control group (for Alcian blue, real-time RT-PCR, and western blot assays and just for detect morphology of differentiated cells, MSCs was also cultured on the surface of cell culture flask).
MTT test
The survival of the cells was checked for 5 days of cell culture on a scaffold in the presence of basal medium. Sterilized PEO/PCL and PEO/Dex-PCL scaffolds were placed in 96-well culture plate, seeded with 5 × 103 cells per cm2, and incubated at 37 °C in 5% CO2. Over 1, 2, 3, 4, and 5 days cell seeding, 10 μl MTT solution (5 mg/ml in DMEM) was added to each well (n = 3). For conversion of MTT to formazan crystals by mitochondrial dehydrogenases of living cells, each plate was incubated at 37 °C for 3.5 h. For dissolution of dark blue intracellular formazan, the supernatant was removed and a constant amount of DMSO was added as solvent. Optical density was read in a micro-plate reader at 570 nm (BioTek Instruments, Winooski, VT. The same procedure was performed in cultured cells with TCPS as a control.
Proteoglycans staining
Alcian blue staining method was used to stain proteoglycans present in ECM of chondrocytes at days 7 and 21. The stem cell seeded scaffolds (BM and DM) were fixed in 4% paraformaldehyde at 4 °C for 30 min. After washing with PBS, scaffolds were incubated with 3% acetic acid. Proteoglycans were stained with Alcian blue in 3% acetic acid (pH 2.5) at room temperature for 30 min. After washing twice with PBS, scaffolds were observed under a light microscope (Nikon TE 2000).
Real-time RT-PCR
Real-time RT-PCR was used for relative quantification of four critical chondrogenic-related genes, including Sox-9, collagen type-1, collagen type-2, and aggrecan at days 7, 14, and 21 during stem cell differentiation. Total RNA was extracted using RNeasy kit (Qiagen, Germantown, MD) according to the manufacturer’s protocol. RevertAid First Strand cDNA synthesis kit (Fermentas, Burlington, Ontario, Canada) was also used to synthesize complementary strands. PCR parameters included denaturation at 95 °C for 4 min, then 35 cycles at 95 °C for 15 s, annealing at 60 °C for 30 s, and elongation at 72 °C for 30 s. For PCR amplification and real-time RT-PCR, primer sequences are illustrated in . Real-time RT-PCR was performed using SYBR Premix Ex Taq (TaKaRa Bio Inc., Otsu, Shiga, Japan). Relative quantification model was applied to calculate the expression of target genes in comparison to b-actin used as an endogenous control. Gene expression levels were quantified by Rotor Gene 6000 (Corbett, Concorde, New South Wales, Australia).
Table. 1 Primers used in real-time RT-PCR.
Western-blot analysis
Stem cells were seeded on different surfaces and differentiated to cartilage and after 21 days western blot was done to assay Col-2 and Aggrecan protein expression according to the protocol by Hassanian et al. (Citation2014). In brief, differentiated cells were lysed with the Pierce IP lysis buffer (25 mM Tris-HCl, pH 7.4, 150 mM NaCl, 1 mM EDTA, 1% NP-40, 5% glycerol) supplemented with protease and phosphatase inhibitor cocktail (Thermo Scientific Pierce, Rockford, IL). After sonication (Misonix Sonicator 3000), the lysates were centrifuged at 13,500 rpm for 15 min at 4 °C and the supernatant was removed as protein solution, then protein content was measured and loaded on SDS-PAGE, after that the samples were transferred to polyvinylidene difluoride membranes (Immobilon-P, Millipore, Bedford,MA). After blocking the immunoblots, membrane incubated with anti-Collagen II antibody ([EPR12268], (ab188570) abcam) and anti-Aggrecan antibody (ab36861, abcam) as primary and goat anti-Rabbit IgG H&L (HRP) preadsorbed (ab97080) as secondary antibodies were developed with Enhanced Chemiluminescence reagents (Millipore, Billerica, MA).
Statistical analysis
All experiments were performed thrice. Data were shown as mean ± SD. REST 2009 software was used for Real-time RT-PCR data analysis and the one-way analysis of variance (ANOVA) using SPSS version 19 (SPSS, Chicago, IL)was used for the evaluation of significance whereas 0.05 was considered as P values for statistical significance.
Results
Nanofiber characterization
The morphology and size of fabricated nanofibrous scaffolds were characterized using SEM and mechanical stability was analyzed using tensile strength. The results of SEM images of PEO/PCL scaffolds showed that nanofibers are smooth, bead free, and in nanometer scale with an average of 125 ± 568 nm (), morphology and size of nanofibers was not changed in Dex-incorporated PEO/PCL (data were not shown). SEM was also used for the biocompatibility evaluation of scaffolds and as shown in the results MSCs were proliferate and expanded very fine on the surface of nanofibers on day 7 ( for PEO/PCL and for PEO/Dex-PCL) and at day 21 ( for PEO/PCL and for PEO/Dex-PCL). According to the SEM results, it can be understood that increased density and regular distribution of stem cells was detected at day 7 to day 21 in PEO/Dex-PCL compared to the PEO/PCL and demonstrated that Dex-incorporated scaffold is a more suitable substrate for growing stem cells. Mechanical cues play an important role in cells’ fate and tissue regeneration; among these, the mechanical properties of the scaffolds such as stress, strain, and elastic modulus should be suitable to induce Osteogenic differentiation and support loading bar in bone tissue. In order to investigate the effect of PEO/Dex on mechanical properties of PEO/Dex-PCL hybrid nanofibers, typical stress–strain curves of PCL, and PEO/Dex-PCL hybrid nanofibrous scaffolds under tensile loading were compared. The tensile strength and elongation at break were obtained as 1.63 MPa and 72.04%, respectively, for PCL nanofibers. The tensile strength and strain of PEO/Dex-PCL nanofibers were reduced to 1.69 MPa and 10.41%, respectively. Moreover, the elastic modulus of PCL and PEO/Dex-PCL nanofibrous scaffolds were obtained as 20.89 and 88.50 MPa. Contact angle of PCL nanofibers was 128°, which decreased significantly to 35° for PEO/PCL scaffold and 24° for PEO/Dex-PCL (. The profile of the release of Dex during one week demonstrated the burst release of Dex at the first 3 h, which was related to its concentration gradient. Since PEO is a water-soluble polymer, this burst is a result of nanofiber’s surface degradation due to media penetration and could also due to surface existence of Dex resulting in the incomplete incorporation into the fibers. However, release pattern of Dex from scaffold showed increasing trend for more than one week of the study (. MTT assay was used for the quantitative evaluation of scaffolds biocompatibility and Dex effect on cell viability in four groups including MSCs cultured on PEO/Dex-PCL, PEO/PCL, TCPS-Dex, and TCPS and control (. No significant difference was detected during the first 2 days after cell seeding, but from day 3, viability of MSCs cultured on the surface of PEO/Dex-PCL and PEO/PCL was significantly higher than TCPS-Dex and TCPS. MTT results also showed that Dex don’t have effect on viability of stem cells when incorporated in scaffolds or diluted in media in TCPS group.
Figure 1. SEM images. (A and B) Dex-embedded PEO nanofibers composited to PCL (PEO/Dex-PCL) at two magnifications. (C) MSCs seeded PEO/PCL and (D) PEO/Dex-PCL at day 7 and (E) MSCs seeded PEO/PCL and (F) PEO/Dex-PCL at day 21.
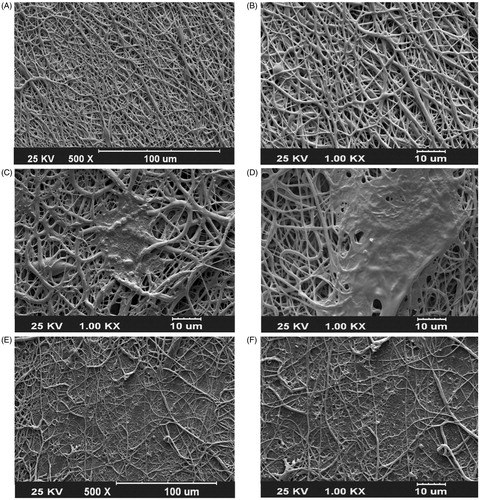
Figure 2. Contact angle measurements to determine hydrophilic properties of PCL, PEO/PCL, and PEO/Dex-PCL nanofibrous scaffolds.
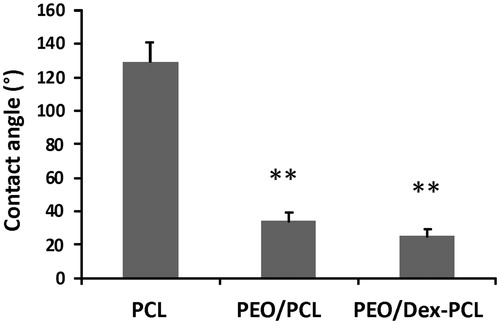
Stem cells culture
Isolated stem cells from human adipose tissue at passage 3 look like fibroblasts, spindle-shape, and almost the same morphology when observed by light microscopy (). When this passage cells reach 90% confluence MSC surface markers were evaluated using flow cytometry (). Stem cells were negative to CD34, HLA-DR, and CD45 and were positive for CD44, CD 90, and CD 105. Morphology of stem cells after 21 days of culture was observed using differentiation medium under light microscopy ().
Chondrogenic differentiation evaluation
Alcian blue staining
For comparing chondrogenic potential of MSCs on Dex-incorporated and unincorporated scaffolds Alcian blue staining (), real-time RT-PCR (), and western blot () was used. Highest-stained glycosaminoglycan (GAG) of the extracellular matrix of differentiated cells was detected in stem cells cultured on PEO/Dex-PCL during days 14 () and 21 () in comparison to the PEO/PCL (). Stem cells cultured on TCPS were also used for Alcian blue staining () but since the light can pass from scaffolds, it’s not true comparing TCPS staining with scaffolds because contrast of TCPS images is very higher than scaffolds images. DAPI staining of nucleus of cultured stem cells was used to confirm that the cells are present on the surface of the nanofibers ().
Figure 6. Glycosaminoglycan (GAG) staining of the extracellular matrix of differentiated cells cultured on TCPS at (B) days 14 and (D) 21, PEO/PCL at days (F) 14 and (H) 21 and PEO/Dex-PCL at days (J) 14 and ( L) 21, (A, C, E, G, I, and K) DAPI-stained nanofibers at days 14 for each groups, magnifications 10×.
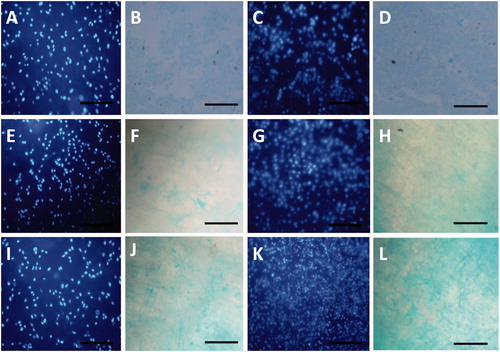
Gene expression
For further evaluation of the chondrogenic potential of MSCs on seeded scaffolds, we also investigated the expression of well-known cartilage-specific gene markers, collagen type II (Col-2), aggrecan, collagen type I (Col-1), and also Sox 9, by Real-time RT-PCR from those cells which were cultured and differentiated on the surface of PEO/Dex-PCL under basal medium (PEO/Dex-PCL-BM), PEO/Dex-PCL under differentiation medium (PEO/Dex-PCL–DM), PEO/PCL under differentiation medium (PEO/PCL-DM), and TCPS under differentiation medium (TCPS-DM) as a control (). Highest expression of Col-2 was detected in PEO/Dex-PCL–DM group during all 7, 14, and 21 days, Col-2 was also significantly highly expressed in PEO/Dex-PCL-BM and PEO/PCL-DM compared to the TCPS-DM group, but no difference was observed between PEO/Dex-PCL-BM and PEO/PCL-DM. Aggrecan was also significantly highly expressed in PEO/Dex-PCL–DM on 3 days. On day 7, stem cells cultured on PEO/Dex-PCL-BM were expressed aggreecan significantly more than TCPS-DM, but on day 14 its expression in scaffolds groups was higher than TCPS-DM. Col-1 expression was also evaluated and result showed that no difference was observed at day 7 and significantly increased at day 14 is detected in PEO/Dex-PCL–DM group, but at day 21 significant increase was observed in PEO/Dex-PCL-BM and PEO/PCL-DM compared to the TCPS-DM. Finally, the highest expression of Sox-9 was also detected in PEO/Dex-PCL–DM group compared to other groups. However, it is important that there were no significant difference between PEO/Dex-PCL-BM and PEO/PCL-DM groups during the Real-time RT-PCR for all four genes.
Protein expression
We also tried to evaluate the expression of two important proteins in chondrogenic differentiation that investigated on the transcript level including Aggrecan () and Col-2 (). As showed in the result, significant over expression of Aggrecan protein was observed in the PEO/PCL/Dex-BM and PEO/PCL/Dex-DM that was the same in the real-time RT-PCR result. Over expression of Col-2 protein was also detected in the PEO/PCL-DM and Pep/PCL/Dex-BM groups and the highest expression was detected in the PEO/PCL/Dex-DM groups.
Discussion
The electrospinning is not an expensive, available, and facile method for fabrication of nanometer scale fibers from several synthetic and natural polymer materials. During the last decade electrospun nanofibrous scaffolds have been used widely in tissue engineering and drug-delivery system due to their unique structural features and good drug-loading capability (Bhardwaj and Kundu Citation2010, Burger et al. Citation2006, Meinel et al. Citation2012). In this study, we used PEO polymers to improve biocompatibility of PCL nanofibers and Dex was also embedded in PEO nanofibers as a simple drug-delivery system to investigate their effects on the chondrogenic differentiation of AT-MSCs. Tailoring the mechanical properties of PEO is necessary for tissue engineering purposes due to drawbacks such as a high-crystallization tendency and a weak mechanical strength.
Various strategies have been considered to overcome these drawbacks such as making hybrid nanofibers of this polymer with suitable polymers such as PCL. So, the hybrid of PEO/Dex and PCL was used as the solution for electrospinning to prepare nanofibrous scaffolds. The fabricated mats showed suitable mechanical properties and also adequate Elastic modulus compared with those observed in pristine PCL scaffolds. Similar results have been obtained by Yao et al. for PCL-PEO hybrid nanofibrous scaffolds. Adult stem cells are very suitable, easily available, and noninvasive source for cartilage tissue engineering application (Guilak et al. Citation2004, Sterodimas et al. Citation2010). These cells have the potential to differentiate into many cells in in vitro culture systems. One of the most well-known adult stem cells is MSCs that is widely used in tissue engineering applications. There are several sources to isolate MSCs, among them adipose tissue is one of the best since the procedure of adipose tissue removal is not invasive, yield of stem cell isolation is high and mortality of isolated stem cells is low (Ardeshirylajimi et al. Citation2015). Several studies have also been shown that chondrogenic differentiation potential of AT-MSCs is very good in comparison to the MSCs isolated from other sources or other stem cells. Hwang et al. demonstrated that cartilage differentiation potential of AT-MSCs in culture plates (Hwang et al. Citation2011).
Tissue engineering required a suitable three-dimensional substrate; this bed creates a good anchorage to cells (Liu et al. Citation2007). Thus, enhancing cell adhesion is important in tissue engineering that is due to hydrophilic surface of scaffolds and mechanical properties of scaffolds are also important to stem cell fate decision. The ideal biological scaffolds need several important criteria that failure in obtaining these criteria limits on certain uses of the biological material. Among them chemical compatibility with aqueous solutions, decomposition of biologically controlled speed, lack of cytotoxic and minimal immune response and inflammation are four very important (Karande and Agrawal Citation2008) limits. PCL, as part of a large family of poly (alpha - hydroxyl esters) and synthetic esters degradable former is widely used in bone and cartilage tissue engineering. Li et al. showed that 3D-scaffolds fabricated from PCL can support adipogenic, chondrogenic, and osteogenic differentiation of MSCs by culturing with a specific medium (Li et al. Citation2005). To improve the chondrogenic potential of PCL nanofibers, Shafiee et al. developed a composite poly (vinyl alcohol) (PVA) with PCL and then the chondrogenic differentiation of MSCs was investigated in vitro and in vivo (Shafiee et al. Citation2011). Their results demonstrated that PVA/PCL nanofibrous scaffolds supported growth and differentiation of stem cells and their animal results were also shown that stem cells seeded PVA/PCL improved healing of rabbit’s cartilage defects. In another study, they investigated chondrogenic potential of four stem cells cultured on PCL hybrid with polylactic acid (PLLA) and their result showed that PCL/PLLA is a very good support for stem cell proliferation and differentiation (Shafiee et al. Citation2015).
In this study, we tried to improve biocompatibility and chondrogenic inductivity of PCL nanofibrous scaffolds by a composite to Dex-incorporated PEO nanofibers. PEO due to graft polar hydrophilic properties and thus can be combined with Dex when put in the cell culture medium, Dex can release slowly and use by cells. Our results showed that biocompatibility of PCL nanofibers significantly increased when composite to PEO nanofibers, but Dex embedding in PEO don’t have a significant effect on PEO/PCL biocompatibility and cell viability. Baker et al. showed that increased pore of PCL scaffold by hybrid with PEO lead to increase infiltration of cells (Baker et al. Citation2008) and this might be one of the reasons of increase cell growth and proliferation that our study and other studies reported. We also found that the mechanical stability of PCL nanofibers significantly decreased after composite with a PEO. Alcian blue staining result showed that best chondrogenic differentiation of stem cells was done in PEO/Dex-PCL. We have done more detailed evaluation of gene expression analysis with increase in groups of study. Gene expression results were shown that PEO/Dex-PCL in the presence of induction media had the best efficiency in differentiation, whereas PEO/Dex-PCL under basal media and PEO/PCL in the presence of induction media almost don’t have much difference in all genes that showed slow release of Dex from nanofibers in PEO/Dex-PCL could have almost the same effects to induction media in a PEO/PCL group. For more verification, western blot assay was also used to evaluate Aggrecan and Col-2 protein expression and results demonstrated that PEO/PCL/Dex-DM has the highest expression level in comparison to the other groups.
In the present study, we try to show that release of Dex from PEO electrospun nanofibers as FDA approved polymers had which effect on the proliferation and chondrogenic potential of AT-MSCs in vitro. Our results demonstrated that the presence of Dex in the medium doesn’t have any effect on the proliferation or viability of At-MSc since no difference was detected in comparison to the control. In the other hands, release of Dex from nanofibers had significant effect on chondrogenic differentiation of stem cells. According to the results, PEO/Dex-PCL could be a promising candidate to use as an implant in cartilage tissue engineering application.
Disclosure statement
The authors report no conflicts of interest. The authors alone are responsible for the content and writing of this article.
References
- Ardeshirylajimi A, Mossahebi‐Mohammadi M, Vakilian S, Langroudi L, Seyedjafari E, Atashi A, Soleimani M. 2015. Comparison of osteogenic differentiation potential of human adult stem cells loaded on bioceramic‐coated electrospun poly (L‐lactide) nanofibres. Cell Prolif. 48:47–58.
- Ardeshirylajimi A, Soleimani M, Hosseinkhani S, Parivar K, Yaghmaei P. 2014. A comparative study of osteogenic differentiation human induced pluripotent stem cells and adipose tissue derived mesenchymal stem cells. Cell J. 16:235–244.
- Baker BM, Gee AO, Metter RB, Nathan AS, Marklein RA, Burdick JA, Mauck RL. 2008. The potential to improve cell infiltration in composite fiber-aligned electrospun scaffolds by the selective removal of sacrificial fibers. Biomaterials. 29:2348–2358.
- Bhardwaj N, Kundu SC. 2010. Electrospinning: a fascinating fiber fabrication technique. Biotechnol Adv. 28:325–347.
- Bhosale AM, Richardson JB. 2008. Articular cartilage: structure, injuries and review of management. Br Med Bull. 87:77–95.
- Buckwalter JA. 2003. Sports, joint injury, and posttraumatic osteoarthritis. J Orthop Sports Phys Ther. 33:578–588.
- Burger C, Hsiao BS, Chu B. 2006. Nanofibrous materials and their applications. Annu Rev Mater Res. 36:333–368.
- Cellesi F. 2012. Thermoresponsive hydrogels for cellular delivery. Ther Deliv. 3:1395–1407.
- Guilak F, Awad HA, Fermor B, Leddy HA, Gimble JM. 2004. Adipose-derived adult stem cells for cartilage tissue engineering. Biorheology. 41:389–399.
- Hassanian SM, Dinarvand P, Rezaie AR. 2014. Adenosine regulates the proinflammatory signaling function of thrombin in endothelial cells. J Cell Physiol. 229:1292–1300.
- Hwang NS, Im SG, Wu PB, Bichara DA, Zhao X, Randolph MA, Langer R, Anderson DG. 2011. Chondrogenic priming adipose-mesenchymal stem cells for cartilage tissue regeneration. Pharm Res. 28:1395–1405.
- Karande T, Agrawal C. Function and Requirement of Synthetic Scaffolds in Tissue Engineering. Boca Raton (FL): CRC Press, 2008.
- Li W-J, Tuli R, Huang X, Laquerriere P, Tuan RS. 2005. Multilineage differentiation of human mesenchymal stem cells in a three-dimensional nanofibrous scaffold. Biomaterials. 26:5158–5166.
- Liu C, Xia Z, Czernuszka J. 2007. Design and development of three-dimensional scaffolds for tissue engineering. Chem Eng Res Des. 85:1051–1064.
- Meinel AJ, Germershaus O, Luhmann T, Merkle HP, Meinel L. 2012. Electrospun matrices for localized drug delivery: current technologies and selected biomedical applications. Eur J Pharm Biopharm. 81:1–13.
- Ng F, Boucher S, Koh S, Sastry KSR, Chase C, Lakshmipathy U, et al. 2008. PDGF, TGF-beta, and FGF signaling is important for differentiation and growth of mesenchymal stem cells (MSCs): transcriptional profiling can identify markers and signaling pathways important in differentiation of MSCs into adipogenic, chondrogenic, and osteogenic lineages. Blood. 112:295–307.
- Patel J, Dunn M. 2015. Cartilage tissue engineering. Regen Eng Musculoskel Tissues Interfaces. 135–160.
- Pirttiniemi P, Peltomäki T, Müller L, Luder HU. 2009. Abnormal mandibular growth and the condylar cartilage. Eur J Orthod. 31:1–11.
- Rath SN, Cohn D, Hutmacher DW. 2008. Comparison of chondrogenesis in static and dynamic environments using a SFF designed and fabricated PCL-PEO scaffold. Virtual Phys Prototyp. 3:209–219.
- Sgaglione NA, Miniaci A, Gillogly SD, Carter TR. 2002. Update on advanced surgical techniques in the treatment of traumatic focal articular cartilage lesions in the knee. Arthroscopy. 18:9–32.
- Shafiee A, Kabiri M, Langroudi L, Soleimani M, Ai J. 2016. Evaluation and comparison of the in vitro characteristics and chondrogenic capacity of four adult stem/progenitor cells for cartilage cell‐based repair. J Biomed Mater Res A. 104:600–610.
- Shafiee A, Soleimani M, Chamheidari GA, Seyedjafari E, Dodel M, Atashi A, Gheisari Y. 2011. Electrospun nanofiber-based regeneration of cartilage enhanced by mesenchymal stem cells. J Biomed Mater Res A. 99:467–478.
- Sterodimas A, de Faria J, Nicaretta B, Pitanguy I. 2010. Tissue engineering with adipose-derived stem cells (ADSCs): current and future applications. J Plast Reconstr Aesthet Surg. 63:1886–1892.
- Temenoff JS, Mikos AG. 2000. Review: tissue engineering for regeneration of articular cartilage. Biomaterials. 21:431–440.
- Yang S, Leong K-F, Du Z, Chua C-K. 2001. The design of scaffolds for use in tissue engineering. Part I. Traditional factors. Tissue Eng. 7:679–689.
- Yang S, Leong K-F, Du Z, Chua C-K. 2002. The design of scaffolds for use in tissue engineering. Part II. Rapid prototyping techniques. Tissue Eng. 8:1–11.