Abstract
The lipopolysaccharide (LPS) of Vibrio cholerae (V. cholerae) plays an important role in cholera disease and the induction of primary protection. In this study, we evaluate mice humoral immune response in intranasal and intraperitoneal administrated V. cholerae LPS. The results showed that the intranasal administration of LPS–chitosan nanoparticle induced the high level of antibodies compared to intraperitoneal injection of antigen without chitosan (P < .001). These results indicated that intranasal and intraperitoneal administration of LPS has been able to induce the high level of antibodies both in the sera and lavage fluid and confirmed our strategy for using intranasal administration of antigen.
Introduction
Cholera disease is the life threatening diarrhea caused by Vibrio cholerae (V. cholerae). This disease is a topical infection of the small intestine and the therefore, the topical defense might be a main determinant of protection against recurrent infection (Onischenko et al. Citation2016). In general, following a natural infection by the V. cholerae, similar to the parenteral injection of killed V. cholerae whole cells vaccine, circulation of specific antibodies could be detected. These antibodies that rose in V. cholerae whole cells when exposed to people were against LPS, outer membrane proteins, flagellum, etc. Furthermore, removal of anti-LPS antibody from these polyclonal antiserums resulted in substantial loss of its protective effect against experimental cholera (Rahman et al. Citation2013, Uddin et al. Citation2014).
The lipopolysaccharide (LPS) of V. cholerae has been known to have an important role in the spread of cholera disease (Chatterjee and Chaudhuri Citation2006). Thus, the endotoxic properties of the V. cholerae is shown to be due to the lipid A. However, the O antigen polysaccharide plays the key role in the immunogenicity and production of vibriocidal antibodies in the host (Sayeed et al. Citation2015). Due to the above-mentioned property, in addition to correlation between serum vibriocidal antibody and protection against cholera disease, the LPS has been used for the preparation and evaluation of cholera vaccines (Patel et al. Citation2012). Several studies revealed that anti-vibrio LPS antibodies can mediate protection from cholera disease through inhibition of intestinal adherence and colonization activities of vibrio (Leung et al. Citation2013, Saha et al. Citation2016).
In recent years, researchers have focused on nanoparticle (NP) as therapeutic agents’ delivery system. Chitosan is a polymer derived from de-N-acetylation of shrimp shell chitin, consists of 20% GlcNAc and 80% GlcN residues (Doavi et al. Citation2016). The biological activity, excellent biocompatibility, and complete biodegradability of the chitosan by combining low toxicity materials, has made it to have extensive applications in medicine, agriculture, food, and non-food industries (Mirnejad et al. Citation2014). Chitosan is a safe biopolymer for delivery of therapeutic agents and antigens. This muco-adhesive NP is shown to enhance bioavailability and mucosal absorption of antigen and improve immunogenicity of intranasal antigen delivery systems (Jahantigh et al. Citation2014). Chitosan NP as site specific vaccine delivery system with specific properties such as high uptake by intestinal M-cells, dendritic cells and also high loading capacity (LC) was used in mucosal immunization (Abkar et al. Citation2015).
Since there is a close relation between local immune defense mediated by intestinal antibodies and recurrence of cholera disease, in this research we loaded V. cholerae LPS on chitosan NP to immunize mice by intranasal administration and compared it to intra-peritoneal injection of antigen-Freund without chitosan.
Materials and methods
Extraction of LPS
Detoxified LPS was extracted from the V. cholerae by the hot phenol–water method as previously described (Pawlowska et al. Citation2004). Briefly, bacterial suspensions centrifuged and the pellets were washed twice in PBS. Pellets were resuspended in PBS and sonicated on ice and protein and nucleic acid of lysed bacteria eliminated enzymatically. At the next step, hot phenol was added to the lysate and after shaking, suspension was centrifuged and sodium acetate and ethanol were added to precipitate the LPS. LPS was dialyzed against distilled water and after characterization, purified LPS was lyophilized and stored at 4 °C.
Preparation and characterization of LPS loaded chitosan nanoparticle
Ten milligrams of chitosan were dissolved in 5 ml of 1% acetic acid containing 1 mg LPS and 0.5% (w/w) Tween 80. Aqueous TPP solution (1 mg/ml) was added drop wise to the chitosan-LPS solution while stirring. Aliquots of 1 ml of the resulting antigen-loaded chitosan NPs suspensions were centrifuged for 15 min at 10,000×g and LPS content of the supernatant was measured by phenol–sulfuric method for evaluation of loading efficacy (LE) (Masuko et al. Citation2005). Morphology of the NPs was determined by scanning electron microscopy (SEM). Size and zeta potential of the synthesized NP were examined by dynamic light scattering (DLS) using a zetasizer SZ3000 (Malvern instrument, Worcestershier, UK).
The amount of LPS entrapped in the NPs was calculated by the difference between the total amounts added to the loading solution and the amount of non-entrapped LPS remaining in the supernatant. A non-loaded NP suspension without LPS was used as a blank to correct any unwanted interference by chitosan (Amini et al. Citation2016). The LE and the LC of the LPS loaded chitosan NPs were calculated from following equations, respectively:
LPSt = total amount of lipopolysaccharide and LPSf = free lipopolysaccharide.
In vitro release study
LPS loaded chitosan NPs were re-suspended in 6 ml of 0.1 M PBS (pH 7.4) and was kept at 37 °C under magnetic stirring (100 rpm). At different time intervals, 0.5 ml of the suspension was taken and centrifuged (18,000×g, 15 min). The carbohydrate concentration in the supernatant was analyzed by phenol–sulfuric assay. The same volume of fresh PBS buffer was added to the release medium to reach the original volume. A sample consisting of only non-loaded chitosan NPs re-suspended in PBS was used as a blank (Amini et al. Citation2016).
Immunization of mice
Sixteen BALB/c female mice aged 8 weeks old were purchased from Pasture Institute of Iran and divided in two groups randomly. All mice were housed in animal unit for three days before doing any experiment. Blood samples were collected from all mice before immunization and the sera were kept at −20 °C for further use as control in each group respectively (Abdollahi et al. Citation2016).
Intraperitoneal immunization by LPS–Freund
The detoxified LPS (5 μg) was mixed with equal volume of complete adjuvant and 350 μl of mixed antigen was injected into peritoneal of each mouse. For booster immunization, 5 μg LPS was mixed with equal volume of incomplete adjuvant and injections were performed after 14 and 28 days. Blood samples were collected at day 35 of first immunization and centrifuged at 3500×g for 5 minutes. Sera were separated and stored at −20 °C for measuring sera antibodies by ELISA (Fasihi-Ramandi et al. Citation2014).
Airway immunization by LPS loaded chitosan
In this group, each mouse was exposed to LPS–chitosan NP (contained 5 μg detoxified LPS) in 20 μl volumes by intranasal administration. Booster immunizations were performed at days 14 and 28 after first immunization. Blood samples were collected at day 35 and centrifuged at 3500×g for 5 minutes. Sera were separated and stored at −20 °C for measuring sera antibodies by ELISA.
Preparation of lavage fluid
At the day 35 of immunization and the collection of blood samples, mice were anesthetized and a seizure was induced in the chest location. Half ml ice cold PBS solution (containing 20 μg/ml PMSF) was injected in to lavage through trachea, and aspirated three times (Ahmadi and Solgue Citation2006). The fluid samples were centrifuged and the supernatants were stored at −20 °C for measuring lavage antibodies by ELISA.
Measurement of antibodies by ELISA
ELISA microplates were coated with 5 μg purified LPS of V. cholerae in 100 μl volumes and left over night at 4 °C. Non-binding sites were blocked by adding 100 μl of 5% skim milk solution into each well for 30 minutes at room temperature. The sera and lavage samples were brought out from 4 °C and let them come to room temperature. Briefly, 100 μl of different dilutions of sample ranging from 1/100 to 1/3200 were added to each lysed LPS pre-coated 96 wells plate and incubated for 60 min at 37 °C (all experiments were done in triplicate).
Microplates were washed three times by washing buffer, and 100 μl of goat antimouse antibody (total antibodies), goat antimouse IgM, IgG and IgA HRP conjugated antibody (diluted 1/4000) (Sigma Aldrich Co., St. Louis, MO) were added to respective wells and incubated for further 1 h at 37 °C. Plates were washed again and substrate (TMB) was added in 100 μl volume and incubated in dark room for 25 minutes. Stopper solution was added and the absorbance was read at 450 nm using Multiscan MS Labsystem (Miami, FL) (Fasihi-Ramandi et al. Citation2015).
Statistical analysis
The results are expressed as the mean of experiments (n = 8) ± standard error. Unpaired t-test was used to determine the probability (P) of two set of data from experiment being different from each other.
Results
Purification yield of detoxified LPS was about 300 mg per liter of fermentation broth of V. cholerae. Purified LPS was found to have protein and nucleic acid contents of <1% (wt/wt) without any significant level of endotoxin (<0.1 EU/μg of sugar) indicating the complete removal of lipid A.
LPS-loaded chitosan NP properties
LPS-loaded chitosan NPs were prepared and optimized in size, charge, and shape. The average diameter of NPs was 150 ± 10 nm and their surface charge was +27 ± 2 mV. LPS-loaded chitosan NP showed the typical spherical shape with smooth surface ()
The loading efficiency and loading capacity of LPS-loaded chitosan NP
In order to calculate LE and LC, the amount of free LPS remaining in the supernatant of the solution and also the weight of dried LPS loaded NP were measured. The loading efficiency and LC of chitosan were determined as 85 ± 3% and 19 ± 2%, respectively.
The profile of LPS release from LPS-loaded chitosan NP
According to the release curves, LPS released from chitosan NP over a 96 h period was evaluated at pH 7.4. In this condition, within 48 h and 72 h, 61.9% and 81.2% of physically loaded LPS was released respectively. After 96 h, percentage of LPS released from LPS loaded chitosan NP reaches 95.3% at pH 7.4 ().
Sera antibodies
The increased antibody against V. cholerae in both two groups of immunized mice indicated that intranasal administration of LPS loaded-chitosan has been able to stimulate immune responses. Moreover, there was a significant difference between these two groups and the level of antibody in nasal group was found to be greater than another group (). The results of the sera IgA showed significant differences between two groups ().
Figure 3. Serum titration of immunized mice with LPS–Freund and LPS–chitosan nanoparticle. Titration of sera total antibody (A) and titration of sera IgA (B) showed a significant difference between two groups (P < .001).
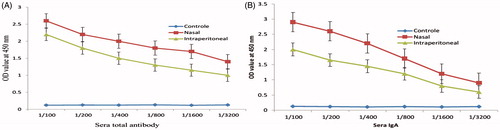
In the case of IgG antibody, the results revealed that both group produced the high level of IgG and the mice subjected to intranasal administration of chitosan-loaded LPS produced greater IgG than other group (). The results of IgM isotype showed no significant differences between two groups ().
Lavage fluid antibodies
The results of IgG and IgA antibodies in the lavage fluid revealed that both groups produced high levels of antibodies and the mice subjected to nasal administration of chitosan-loaded LPS produced greater IgG and IgA in comparison to intraperitoneal group but IgG level has no significant difference between nasal and intraperitoneal administration roots ().
Discussion
V. cholerae LPS has been shown to have a significant role in the spread of the disease and the O-antigen polysaccharide (O-PS) is mainly responsible for the immunogenicity and the production of antibodies (Chatterjee and Chaudhuri Citation2006). V. cholerae LPS with adjuvant properties also exhibits mitogenic effect and induces the proliferation of murine splenocytes (Bhuiyan et al. Citation2015, Patel et al. Citation2012). This bacterial component is immunogenic and induces protective immune responses in host (Fournier Citation1998). Therefore, it could be used for cholera vaccine. The measurable level of antibodies of the main isotypes in the sera and lavage fluid of both groups indicate that the nasal administration of LPS-loaded chitosan in to BALB/c mice has been able to stimulate the humoral immunity response (Leung et al. Citation2014, Song and Sun Citation2015). In this research, we observed high levels of IgG and IgA isotype antibodies against V. cholerae LPS. Supporting our findings, Patel also reported the presence of memory B cell responses and production of specific IgA and IgG against V. cholerae LPS (Patel et al. Citation2012). In other studies, it was revealed that anti-LPS antibodies, especially IgG is present at the mucosal surface of lavage and intestinal tract as a result of transudation process and also in some other pathogenic organisms, high concentration of purified LPS could act as mitogenic agent for mouse B cells resulting in all immunoglobulin production (Fasihi-Ramandi et al. Citation2016, Pasetti et al. Citation2011, Skidmore et al. Citation1975). On the other hand, the specific IgG and IgA antibodies against V. cholerae LPS in the serum, milk, and saliva of human after subcutaneous cholera vaccine confirmed the similarity of immune response against V. cholerae LPS in both human and mice (Karlsson et al. Citation2014, Murugaiah et al. Citation2014). Thus, exposure to V. cholerae by either vaccination or natural infection results in induction of both T cell dependent (IgG, IgA) and T cell independent response (Longini et al. Citation2007). Therefore, production of IgG and IgA antibodies by chitosan-V. cholerae LPS in this study may help scientists for finding the new protective vaccine in future studies.
In this study, we used intranasal administration as one of the promising routes for needle-free antigen delivering to novel vaccination that has several advantages such as reduction of the necessary antigen dose in result of low protease activity of nasal cavity, induction of humoral and mucosal immune responses, providing additional protection correlated with SIgA and greater acceptable alternative to patients (De Magistris Citation2006). In this study, the chitosan-loaded V. cholerae LPS NP was used to deliver the V. cholerae LPS antigen into animal model to induce immune response. In agreement to our claim, intranasal administration of diphtheria loaded chitosan in BALB/c mice has been used for the production of protective antibody against diphtheria toxin (Arthanari et al. Citation2016, Rezaei Mokarram and Alonso Citation2016). Though, vibriocidal responses seem to correlate better with IgM than IgG isotype yet, IgA and IgG, rather than IgM, play an important role in the protection against cholera disease. IgG antibodies penetrate into the lumen of the intestine; therefore, it is likely to be more effective in the presence of secretory IgA in the sera and fluid (Boustanshenas et al. Citation2013). Therefore, finding high level IgG and IgA in the mice subjected to nasal administration of chitosan-loaded V. cholerae LPS NP or intra peritoneal injection of V. cholerae LPS in this study suggested that chitosan-loaded V. cholerae LPS NP could be a suitable choice to induce protective immunity against V. cholerae.
Acknowledgements
This article is extracted from MSc thesis of Hamideh Ghobadi-Ghadikolaee. The authors wish to thank their colleagues in the Immunology Laboratory for their kind help.
Human and animal right: All protocols of the study were approved by institutional animal ethics committee of Baqiyatallah University of Medical Sciences which follows the NIH guidelines for care and use of animal.
Disclosure statement
The authors declare that they have no conflicts of interest.
Additional information
Funding
References
- Abdollahi A, Mansouri S, Amani J, Fasihi MR, Moradi M. 2016. Immunoreactivity evaluation of a new recombinant chimeric protein against Brucella in the murine model. Iran J Microbiol. 8:193–202.
- Abkar M, Lotfi AS, Amani J, Eskandari K, Ramandi MF, Salimian J, et al. 2015. Survey of Omp19 immunogenicity against Brucella abortus and Brucella melitensis: influence of nanoparticulation versus traditional immunization. Vet Res Commun. 39:217–228.
- Ahmadi K, Solgue G. 2006. Cytokine pattern in sera and broncho-alveolar lavage six months after single exposer to sulfur mustard. Med J Islam Repub Iran (MJIRI). 20:52–56.
- Amini Y, Moradi B, Fasihi-Ramandi M. 2016. Aluminum hydroxide nanoparticles show strong activity to stimulate Th-1 immune response against tuberculosis. Artif Cells Nanomed Biotechnol. 20:1–5.
- Amini Y, Tebianian M, Mosavari N, Fasihi Ramandi M, Ebrahimi SM, Najminejad H, et al. 2016. Development of an effective delivery system for intranasal immunization against Mycobacterium tuberculosis ESAT-6 antigen. Artif Cells Nanomed Biotechnol. 28:1–6.
- Arthanari S, Mani G, Peng MM, Jang HT. 2016. Chitosan-HPMC-blended microspheres as a vaccine carrier for the delivery of tetanus toxoid. Artif Cells Nanomed Biotechnol. 44:517–523.
- Bhuiyan TR, Hoq MR, Nishat NS, Al Mahbuba D, Rashu R, Islam K, et al. 2015. Enumeration of Gut-Homing beta7-positive, pathogen-specific antibody-secreting cells in whole blood from enterotoxigenic Escherichia coli- and Vibrio cholerae-infected patients, determined using an enzyme-linked immunosorbent spot assay technique. Clin Vac Immunol. 23:27–36.
- Boustanshenas M, Bakhshi B, Ghorbani M. 2013. Investigation into immunological responses against a native recombinant CTB whole‐cell Vibrio cholerae vaccine in a rabbit model. J Appl Microbiol. 114:509–515.
- Chatterjee S, Chaudhuri K. 2006. Lipopolysaccharides of Vibrio cholerae: III. Biological functions. Biochim Biophys Acta (BBA)-Mol Basis Dis. 1762:1–16.
- De Magistris MT. 2006. Mucosal delivery of vaccine antigens and its advantages in pediatrics. Adv Drug Deliv Rev. 58:52–67.
- Doavi T, Mousavi SL, Kamali M, Amani J, Fasihi Ramandi M. 2016. Chitosan-based intranasal vaccine against Escherichia coli O157:H7. Iran Biomed J. 20:97–108.
- Fasihi-Ramandi M, Amani J, Salmanian A, Moazzeni S, Ahmadi K. 2015. Production and Characterization of new anti-human CD20 monoclonal antibody. Iran J Allergy Asthma Immunol. 14:502–508.
- Fasihi-Ramandi M, Ghobadi-Ghadikolaee H, Ahmadi-Renani S, Ahmadi K. 2016. Serum anti-Vibrio cholerae immunoglobulin isotype in BALB/c mice immunized with ompW-loaded chitosan. Int J Enteric Pathog. 4:e33137. Epub 2016-05-01.
- Fasihi-Ramandi M, Nedjad-Moghaddam A, Arabsalmany F, Asgari S, Ahmadi-Renani S, Ahmadi K. 2014. Production and characterization of monoclonal and polyclonal antibody against recombinant outer membrane protein. Am J Immunol. 10:56–62.
- Fournier JM. 1998. The current status of research on a cholera vaccine. Bull Soc Pathol Exot. 91:412–415.
- Jahantigh DSM, Fasihi Ramandi M, Mousavi M, Zand AM. 2014. Novel intranasal vaccine delivery system by chitosan nanofibrous membrane containing N-terminal region of IpaD antigen as a nasal Shigellosis vaccine, Studies in Guinea pigs. J Drug Deliv Sci Technol. 24:33–39.
- Karlsson SL, Ax E, Nygren E, Kallgard S, Blomquist M, Ekman A, et al. 2014. Development of stable Vibrio cholerae O1 Hikojima type vaccine strains co-expressing the Inaba and Ogawa lipopolysaccharide antigens. PLoS One. 9:e108521.
- Leung DT, Bhuiyan TR, Nishat NS, Hoq MR, Aktar A, Rahman MA, et al. 2014. Circulating mucosal associated invariant T cells are activated in Vibrio cholerae O1 infection and associated with lipopolysaccharide antibody responses. PLoS Negl Trop Dis. 8:e3076.
- Leung DT, Uddin T, Xu P, Aktar A, Johnson RA, Rahman MA, et al. 2013. Immune responses to the O-specific polysaccharide antigen in children who received a killed oral cholera vaccine compared to responses following natural cholera infection in Bangladesh. Clin Vac Immunol. 20:780–788.
- Longini IM Jr, Nizam A, Ali M, Yunus M, Shenvi N, Clemens JD. 2007. Controlling endemic cholera with oral vaccines. PLoS Med. 4:e336.
- Masuko T, Minami A, Iwasaki N, Majima T, Nishimura S, Lee YC. 2005. Carbohydrate analysis by a phenol–sulfuric acid method in microplate format. Anal Biochem. 339:69–72.
- Mirnejad R, Jahromi M, Ali M, Al-Musawi S, Pirestani M, Fasihi Ramandi M, et al. 2014. Curcumin-loaded chitosan tripolyphosphate nanoparticles as a safe, natural and effective antibiotic inhibits the infection of Staphylococcus aureus and Pseudomonas aeruginosa in vivo. Iran J Biotechnol. 12:e1012.
- Murugaiah C, Nik Mohd Noor NZ, Mustafa S, Manickam R, Pattabhiraman L. 2014. Construction and evaluation of V. cholerae O139 mutant, VCUSM21P, as a safe live attenuated cholera vaccine. PLoS One. 9:e81817.
- Onischenko GG, Popova AY, Kutyrev VV, Smirnova NI, Scherbakova SA, Moskvitina EA, et al. 2016. Actual problems of epidemiologic control, laboratory diagnostics and prophylaxis of cholera in Russian Federation. Zh Mikrobiol Epidemiol Immunobiol. 1:89–101.
- Pasetti MF, Simon JK, Sztein MB, Levine MM. 2011. Immunology of gut mucosal vaccines. Immunol Rev. 239:125–148.
- Patel SM, Rahman MA, Mohasin M, Riyadh MA, Leung DT, Alam MM, et al. 2012. Memory B cell responses to Vibrio cholerae O1 lipopolysaccharide are associated with protection against infection from household contacts of patients with cholera in Bangladesh. Clin Vac Immunol. 19:842–848.
- Pawlowska K, Lodowska J, Jaworska-Kik M, Dzierzewicz Z, Weglarz L, Wilczok T. 2004. Choice of isolation procedure for endotoxins from the outer membrane of the bacteria Desulfovibrio desulfuricans. Med Dosw Mikrobiol. 56:293–300.
- Rahman A, Rashu R, Bhuiyan TR, Chowdhury F, Khan AI, Islam K, et al. 2013. Antibody-secreting cell responses after Vibrio cholerae O1 infection and oral cholera vaccination in adults in Bangladesh. Clin Vac Immunol. 20:1592–1598.
- Rezaei Mokarram A, Alonso M. 2016. Preparation and evaluation of chitosan nanoparticles containing diphtheria toxoid as new carriers for nasal vaccine delivery in mice. Arch Razi Inst. 61:13–25.
- Saha A, Khan A, Salma U, Jahan N, Bhuiyan TR, Chowdhury F, et al. 2016. The oral cholera vaccine Shanchol when stored at elevated temperatures maintains the safety and immunogenicity profile in Bangladeshi participants. Vaccine. 34:1551–1558.
- Sayeed MA, Bufano MK, Xu P, Eckhoff G, Charles RC, Alam MM, et al. 2015. A cholera conjugate vaccine containing O-specific polysaccharide (OSP) of V. cholerae O1 Inaba and recombinant fragment of tetanus toxin heavy chain (OSP:rTTHc) induces serum, memory and lamina proprial responses against OSP and is protective in mice. PLoS Negl Trop Dis. 9:e0003881.
- Skidmore BJ, Chiller JM, Morrison DC, Weigle WO. 1975. Immunologic properties of bacterial lipopolysaccharide (LPS): correlation between the mitogenic, adjuvant, and immunogenic activities. J Immunol. 114:770–775.
- Song T, Sun R. 2015. Pharmacodynamics study of zedoary turmeric oil chitosan microspheres administered via arterial embolization. Artif Cells Nanomed Biotechnol. 44:1958–1963.
- Uddin T, Aktar A, Xu P, Johnson RA, Rahman MA, Leung DT, et al. 2014. Immune responses to O-specific polysaccharide and lipopolysaccharide of Vibrio cholerae O1 Ogawa in adult Bangladeshi recipients of an oral killed cholera vaccine and comparison to responses in patients with cholera. Am J Trop Med Hyg. 90:873–881.