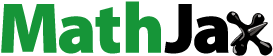
Abstract
Pseudomonas aeruginosa and its lipopolysaccharides play a key role in the pathogenesis of diabetic foot infection, for which, currently no effective therapeutic agents are available. Hence, newer forms of therapeutic agents are required for treating Pseudomonas aeruginosa infection. In this present study, nanocrystalline silver nanoparticles (AgNPs) were synthesized using culture filtrate of Brevibacillus brevis KN8(2) followed by an investigation of its in vivo anti-pseudomonal and anti-endotoxic properties. Biosynthesized AgNPs was predominantly cubical in shape with an average particle size of 15.40 nm as observed through field emission scanning electron microscopy (FESEM) and X-ray diffraction analysis. The LC-ESI-MS/MS analysis indicates the presence of surfactin in culture filtrate of B. brevis KN8(2). The MIC of surfactin-stabilized AgNPs against P. aeruginosa was 10 μg ml−1 and its wound repair activity was observed in P. aeruginosa-infected wounds of diabetic mice by measuring wound area closure, bacterial counts, mRNA expressions, and histopathology. Further, surfactin-stabilized AgNPs suppressed the transcription of LPS-triggered expression of the TNF-α in wounds that LPS-assisted extension of wound repair in diabetes mellitus conditions was circumvented quite well. Results gathered in this study established that surfactin-stabilized AgNPs could effectively offer to the novel treatment of Gram-negative bacilli infection in diabetic wounds.
Introduction
Diabetes mellitus, affecting nearly 6.4% of the population worldwide, is a major public health problem that plagues human kind across age, gender, geographical boundaries, and any other form of distinguishable or discriminatory divides. Projection made on this health catastrophe is alarming that individuals affected by this disease are expected to double by the year 2030 [Citation1]. Diabetic manifestations are recognized by various disorders including diabetic foot ulcer [Citation2] and it arises primarily from peripheral neuropathy or mechanical changes in the foot architecture. Ulceration creates a portal entry for several opportunistic pathogens including the Gram-negative pathogenic bacteria Pseudomonas aeruginosa and it establishes infection in and around the wound sites [Citation3]. Infections caused by P. aeruginosa and its released virulence factors, particularly endotoxin (lipopolysaccharide [LPS]) predispose wounds to prolonged inflammatory conditions which induces higher concentrations of proteases causing the degradation of multiple growth factors, receptors, and matrix proteins that are essential for wound healing [Citation4]. This leads to the formation of chronic wounds eventually ending up in lower limb amputation. Infection in diabetic foot ulcer is clearly a primary cause of lower extremity amputations in diabetic patients than those without infection in wounds [Citation5,Citation6]. Gariani et al. [Citation7] observed that diabetic patients who have undergone a lower extremity amputation have a maximal chance of survival for only 5-years or less among which 50% succumb to death earlier than in comparison with most cancers. For this reason, chronically infected diabetic foot ulcers are considered the most significant wound care problem in the world and it is imperative to prevent or reduce limp amputation with newer forms of therapeutic strategies.
Silver has been used as anti-infective agent against a diverse group of pathogenic bacteria since the ancient period, but the discovery of antibiotics has been replaced the usage of silver. However, the development of resistance among microbial pathogens including P. aeruginosa has stimulated the rebirth of using silver especially silver nanoparticles (AgNPs) to manage the resistant pathogens [Citation8]. Although AgNPs are produced through both physical and chemical means for a long time, biological synthesis of AgNPs using microorganisms has been received deserving attention in the recent times. Medical applications of physico-chemically synthesized AgNPs have certain constraints due to the harmful elements they carry as they exert toxic effects on mammalian cells. Therefore, biologically synthesized AgNPs can only be the viable alternate for biomedical applications [Citation9–12]. Studies pursued with the soil bacteria Brevibacillus brevis KN8(2) being reported elsewhere show that peptide antibiotic isolates collected from cell pellets can offer to the production of antimicrobial compounds (Natarajan et al. unpublished data), while AgNPs prepared from the supernatant can be hired for the treatment of diabetic wound infection caused by gram-negative bacilli such as P. aeruginosa and to neutralize its virulence factor such as LPS which assisted extension of wound repair in diabetes mellitus conditions. We report here that AgNPs were synthesized from the rhizosphere soil bacterium B. brevis KN8(2) that can be attributed with anti-pseudomonal and anti-endotoxin activity that would find immense implications in curing wound infection relevant in the treatment of diabetic foot infection.
Materials and methods
Biosynthesis of silver nanoparticles
The bacteria B. brevis KN8(2) (NCBI accession no. JX971445.1) was used for the synthesis of AgNPs. Brevibacillus brevis KN8(2) was inoculated in nutrient broth and incubated at 37 °C in a shaking incubator for 2 d at 37 °C. The fermentation broth was centrifuged at 6000 rpm for 20 min and the cell pellet was discarded. The supernatant solution was collected and mixed with an aqueous solution of 1 mM AgNO3 and it kept at 37 °C in dark.
Characterization of silver nanoparticles
UV–visible spectroscopy
After the preliminary detection of AgNPs synthesis carried out by visual observation based on colour change of the culture filtrate, the optical characteristics of synthesized AgNPs was measured using UV–visible spectrophotometer (ELICO SL-159 model, ELICO, Hyderabad, India) at the resolution of 1 nm from 300 to 900 nm.
Field emission scanning electron microscopy (FESEM)
For characterizing shape of the biosynthesized AgNPs, FESEM was performed. A drop of the sample was loaded over copper-coated grid, and the solvent was allowed to evaporate under infra-red light for 30 min. The FESEM images were acquired by using FESEM (ZEISS ULTRA 55, ZEISS, Oberkochen, Germany) operated at an accelerating voltage of 3.0 KV.
Dynamic light scattering (DLS) and zeta potential
The particle size distribution and zeta potential of the AgNPs was characterized using DLS measurements conducted with a Malvern Zetasizer Nanoseries compact scattering spectrometer (Malvern Instruments Ltd, Malvern, UK).
X-ray diffraction (XRD)
XRD measurement of the biologically synthesized AgNPs was done on X-ray diffractometer (XRD, Phillip’s XPERT PRO, PANalytical B.V., Eindhoven, The Netherlands) operating at a voltage of 40 kV and a current of 30 mA with Cu Kα1 radiation of 1.54060 nm wavelength. The scanning was done in the region of 2θ from 20° to 80° at 0.02°/min and the time constant was 10 s.
Characterization of biomolecule responsible for synthesis and stabilization of silver nanoparticles
Fourier transform-infrared spectroscopy (FT-IR)
To identify the functional groups of reducing and stabilizing agent present in the cell-free culture filtrate of B. brevis KN8(2) for synthesis of AgNPs, cell-free culture filtrate was acidified and the product obtained by acidic precipitation then subsequent recovery with methanol. The precipitate containing the metabolite was dissolved in sterile distilled water and FT-IR spectrum was recorded using the Perkin-Elemer, Waltham, MA, Model: Spectrum RX1. Measurement was carried out in the frequency range of 400–4000 cm−1 at a resolution of 4 cm−1.
Liquid chromatography–electrospray ionization–mass spectroscopy/mass spectroscopy (LC–ESI–MS/MS)
The LC–ESI–MS/MS analysis was carried out by passing the sample through a reverse-phase column (Zorbax 300 SB-C8, 2.1 mm 6100 mm, 3.5 mm, Thermo Fisher Scientific, Waltham, MA) attached to an electro spray ionization mass spectrometer. Separation of the compound was effected in this column via a gradient elution using water and acetonitrile (ACN), each containing 0.1% formic acid, at a flow rate of 0.2 ml min−1, and the eluting peptide was characterized by conventional MS/MS (LC–ESI–MS; LC–ESI–MS/MS). The mass spectrometer, an HCT Ultra PTM Discovery (BrukerDaltonics, Billerica, MA), had an ESI source and housed a classic ion trap (Paul type), using which, tandem MS (LC–ESI–MS/MS) data were acquired. The MS conditions were capillary temperature 250 °C, source voltage 4.0 kV, source current 80.0 μA, and capillary voltage 7.0 V, in positive mode. Mass spectrum was analyzed using Flex-analysis software, provided by the manufacturer.
Determination of minimum inhibitory concentration (MIC)
The MIC of the AgNPs synthesized from the cell-free culture filtrate of B. brevis KN8(2) against P. aeruginosa was determined by the Luria Broth (LB) dilution method. Various concentrations of AgNPs were added from a starting concentration of 1 μg ml−1 and they were diluted serially in the 96 wells microtiter plate. Gentamycin was used as a positive control while sterile distilled water was used as a negative control. They were then incubated at 37 °C for 24 h. The minimum concentration of AgNPs that induced a complete growth inhibition was recorded as MIC.
Bacterial growth and inoculums
The pathogenic bacterium used in this study was P. aeruginosa (MTCC 741) and it was grown in Luria–Bertani (LB) medium. Aliquots (50 μl) of overnight cultures were sub cultured in fresh LB broth and grown at 37 °C for 4 h to an optical density of approximately 0.9 at 600 nm. A 100 μl aliquot of the culture was then pelleted, washed in phosphate-buffered saline (PBS) and serially diluted (10-fold serial dilutions) in PBS. A 20 μl aliquot of the 10−3 dilution (equivalent to approximately 104 CFU) was applied topically to the wound of each mouse.
Mouse model for chronic infected diabetic wound healing
The in vivo efficacy of surfactin-stabilized biogenic AgNPS against P. aeruginosa was evaluated using Swiss albino mice as animal model. Healthy pathogen-free Swiss albino male mice weighing 30–35 g was used in this study. The animals were maintained under standard conditions of humidity, temperature (25 ± 2° C), and light (12 h light/12 h dark). The animals were housed in individual cages with a standard animal-pelleted diet and had free access to water. The protocol of the present investigation was approved by Institutional Animal Ethics Committee (IAEC), Bharathidasan University, India.
Diabetes was induced by a single intraperitoneal injection of streptozotocin (STZ) (60 mg/kg) in citrate buffer, pH 4.5. Seven days after diabetes induction blood glucose levels were checked using an Accu-Chek Aviva glucometer (Roche Diagnostics GmbH, Berlin, Germany). Animals with blood glucose levels higher than 300 mg/dl were considered diabetic. Mice were anesthetized by intraperitoneal injection of xylazine (13 mg/kg) and ketamine (66.7 mg/kg). The dorsal hairs of diabetic mice were shaved and 2 cm × 2 cm surgical excision wounds were created with sterile surgical blade and scissor. Infections of the wounds in mice were initiated by applying 20 μl of P. aeruginosa culture containing ∼104 CFU. All the mice with excisional wounds were divided into four groups of three mice each (1) uninfected group, (2) infected untreated group, (3) infected treated with gentamycin group, and (4) infected treated with surfactin-stabilized AgNPs group. Two hour after inoculation, per day two times (morning and evening) 50 μl of gentamycin (0.5%) was topically applied on the third group of mice for 4 weeks and 50 μl of surfactin stabilized AgNPs (0.1%) was topically applied on the fourth group of mice for 4 weeks and the wound area were covered with sterile dressings in subsequent days. The mice were sacrificed humanely by cervical dislocation on the 28th day. Tissue samples of the wounds were harvested for RNA extraction and histopathological examination.
Assessment of bacterial load and wound closure
Bacterial counts in wounds were assessed quantitatively by spread plate method from the homogenized tissue biopsy at day 28. To assess the effect of surfactin-stabilized AgNPs on healing of the wounds on each mouse were digitally photographed on days 1, 14, and 28. The wound size was calculated on days 1 and 28 using ImageJ software (v 1.36 b; National Institutes of Health, Bethesda, MD). The percentage of wound closure was calculated according to the following formula:
Reverse transcription polymerase chain reaction(RT-PCR)
Total RNA was extracted from granulation tissues by using TRIzol Reagent (Invitrogen, Carlsbad, CA). One microgram of total RNA was used for cDNA synthesis using high capacity cDNA reverse transcription kit (Applied Biosystem, Foster City CA) according to the instructions from the manufacturer. Then PCR was performed in eppendorf thermocycler for the cDNA using the following specific sets of primers () were used for target gene amplification. Then PCR products were subjected to electrophoresis in 1% agarose gel, which was then stained with ethidium bromide and photographed under UV illumination. The fold changes of mRNA expressions were analyzed by using image J software (National Institutes of Health, Bethesda, MD).
Table 1. Primer sequences for PCR for each target gene.
Histopathological analysis
In order to study the response of wounds to treatment, wounded tissues from mice of different groups were excised on day 28. The tissues were fixed in 10% neutral buffered formalin, following which all the sections were processed using a standard histological procedure. The deparafinized sections were stained with haematoxylin/eosin (H&E) staining. The slides were examined under a microscope to determine the tissue change such as fibroblast, collagen deposition, blood capillary, and re-epithelization.
Neutralization of LPS toxicity in wounds
LPS was isolated and purified from P. aeruginosa (MTCC 741), grown overnight in Luria–Bertani broth at 37 °C using the phenol/chloroform/petroleum ether method. The isolated and purified LPS were measured using an assay for the specific sugar 2-keto-3-deoxyoctosonic acid (KDO assay) [Citation13]. The isolated LPS was resuspended in endotoxin-free water and used at a concentration of 5 mg kg−1 to induce toxicity in diabetic wounds. The ability of surfactin-stabilized AgNPs to neutralize the toxicity of LPS on wounds was examined by the expression of pro-inflammatory cytokine such as TNF-α from skin wounds treated with LPS. Streptozotocin-induced diabetic mouse was anaesthetized by intraperitoneal injection of xylazine (13 mg/kg) and ketamine (66.7 mg/kg). The dorsal hairs of each mouse were shaved and a 2 cm × 2 cm surgical excision wounds were created with sterile surgical blade and scissor. In these experiments, mouse were randomly divided into four groups of three mice each, group 1 – PBS treated; group 2 – LPS treated; group 3–LPS + surfactin-stabilized AgNPS treated; group 4 – surfactin-stabilized AgNPS treated. Then LPS (5 mg kg−1) was topically applied on the wounds of groups 2 and 3 for 5 d and surfactin stabilized AgNPs (0.1%) was topically applied on the wounds of groups 3 and 4 for 5 d and the wound area was covered with sterile dressings. After 2 weeks, tissue samples of the wounds were harvested for RNA extraction then reverse transcription polymerase chain reaction was performed to quantify the mRNA levels of TNF-α in LPS-treated wounds treated with and without surfactin-stabilized AgNPs. The fold changes of mRNA expressions were analyzed by using image J software (National Institutes of Health, Bethesda, MD).
Data analysis
The values are expressed as mean ± standard deviation (SD). Differences between groups were assessed by one-way ANOVA using Graph Pad Prism 6.0 software package for Windows (Graph Pad Inc., San Diego, CA). Values are statistically significant at ***p < .001, **p < .01, *p < .05.
Results
Synthesis and characterization of silver nanoparticles
Cell-free culture filtrate of B. brevis KN8(2) being mixed with aqueous solution of 1 mM AgNO3 and incubated for about 120 h resulted in the reaction mixture turning from light yellow to dark brown. This clearly indicates the formation of AgNPs substantiated by surface plasmon resonance (. Reaction mixture subjected to UV–visible spectroscopy analysis revealed a characteristic surface plasmon peak at 420 nm which is specific for AgNPs ().
Figure 1. Colour changes and UV?visible absorption spectrum of biologically synthesized silver nanoparticles. (A) cell-free culture filtrate of B. brevis KN8(2) without AgNO3 [yellow colour]; (B) cell-free culture filtrate of B. brevis KN8(2) with1 mM AgNO3 [dark brown colour].
![Figure 1. Colour changes and UV?visible absorption spectrum of biologically synthesized silver nanoparticles. (A) cell-free culture filtrate of B. brevis KN8(2) without AgNO3 [yellow colour]; (B) cell-free culture filtrate of B. brevis KN8(2) with1 mM AgNO3 [dark brown colour].](/cms/asset/49cbba32-9fd3-4800-a26a-4bd4c9402971/ianb_a_1324461_f0001_c.jpg)
Studies undertaken to determine the shape of AgNPs synthesized from B. brevis KN8(2) via FESEM measurement revealed that the particles were cubical in shape and were in polydispersed nature (). Further confirmation of AgNPs with XRD analysis showed the peaks at 38.2°, 45.39°, 66.3°, and 75.3° can be indexed to the (1 1 1), (2 0 0), (2 2 0), and (3 1 1) Bragg’s reflections of cubic structure of silver, respectively (JCPDS no. 03-0921) (). Further, the crystalline size was calculated from the width of the XRD peaks, assuming that they are free from non-uniform strains, using Scherer’s formula D = kλ/βcosθ, where D is the crystallite size, k Scherrer coefficient (0.94), λ wavelength of X-rays (CuKα) (1.5406 Å), β full width half maxima (FWHM) value was 1, and θ Bragg’s angle (half of 2θ–38.2) = 19.1°. The calculated value for the biogenic silver crystalline size was found to be approximately 15.40 nm. The DLS pattern revealed that the synthesized AgNPs have a zeta average diameter of 312.40 nm with polydispersity index of 0.447 (). In addition to hydrodynamic size measurements, the zeta potential was also measured and in the current studies the presence of negatively charged AgNPs with a zeta potential (−36.6 mV) was confirmed by zeta potential measurement ().
Characterization of biomolecule responsible for synthesis and stabilization of silver nanoparticles
The FT-IR spectroscopy analysis was carried out to identify the biomolecules that could be present and be responsible for the synthesis and stabilization of AgNPs in the cell-free culture filtrate of B. brevis KN8(2). The result of the FT-IR spectrum of extracellular extract secreted by B. brevis KN8(2) and AgNPs had shown that the presence of prominent peaks with different values at 3418.29, 2075.17, 1638.46, 1426.04,1365.39, 1226.69, 1096.74, and 674.75 regions () in extracellular extract of B. brevis KN8(2) and AGNPs spectrum had showed that the presence of prominent peaks with different values at 3452.30, 2067.77, 1637.30, 1147.76, 1081.32, 1020.03, and 680.28 regions (). Among the peaks, 3418.29, 1638.46, 1226.69, and 1096.74 present in the culture filtrate and 3452.30 and 1637.30 present in the AgNPs spectrum may be assigned to NH-stretch amide, C=O stretch amide I, C–N stretch amine, and C–N stretch aliphatic amines. This observation indicates the presence of extracellular peptide in the culture filtrate of B. brevis KN8(2) responsible for synthesis and stabilization of cubical shaped AgNPs. Further, the molecular mass of the peptide present in the culture filtrate of B. brevis KN8(2) was analyzed by LC–ESI–MS/MS (). The MS/MS fragmentation pattern obtained from LC–ESI–MS/MS analysis showed the presence of compound with molecular weight 1045.7 which is equivalent to that of surfactin.
MIC
Anti-bacterial efficacy studies pursued in vitro revealed that AgNPs synthesized from B. brevis KN8(2) had the capacity to evince better anti-pseudomonal activity than gentamycin (positive control). The minimum inhibitory concentration of AgNPs against P. aeruginosa is 10 μg ml−1.
Effect of silver nanoparticles on healing of P. aeruginosa-infected wounds
Preclinical trials performed with Swiss albino mice presented interesting insights on the role of surfactin stabilized AgNPs on healing of streptozotocin-induced diabetic wounds infected with P. aeruginosa. For initiating infection in mice wounds, 20 μl of P. aeruginosa suspension containing 104 CFU ml−1 was applied on wounds after 24 h morphologically the wound had more yellowish regions and purulent discharge compared to uninfected wounds. This feature confirmed infection in mice initially. Repeated observations made in this investigation offers to a conclusive finding that amelioration of wound repair response is clearly enhanced in P. aeruginosa infected streptozotocin-induced diabetic wounds by surfactin-stabilized AgNPs prepared using the B. brevis KN8(2) culture extracts (). Post-surfactin-stabilized AgNPs treatment changes in wound size in animals monitored in the group which was compared with other groups showed that surfactin-stabilized AgNPs treated mice evinced strong wound-healing activity in P. aeruginosa-infected diabetic wounds. During the healing process, P. aeruginosa-infected group treated with surfactin-stabilized AgNPs showed a highly significant reduction in the wound area closing up to 98% (p < .01) of the wound surface in duration at day 28 after the application. In contrast, with the P. aeruginosa-infected-untreated group that showed initially an increase in wound size which eventually on positive wound healing could offer a relief only up to 68% (p < .01) reduction of wound size in the same period (). Surfactin-stabilized AgNPs-treated wounds contracted faster and were completely healed by the 29th day revealing the healing response which could be claimed equally in comparison with uninfected groups. It could be noticed that the antibiotic gentamycin-treated mice showed only 84% (p < .01) reduction of wound size after 28th days.
Figure 7. Surfactin-stabilized AgNPs (SSAgNPs) accelerated wound repair in P. aeruginosa infected diabetic mice: (A) representative photographs showing wound closure morphology; (B) percentage of wound area closure at day 28; (C) P. aeruginosa bacterial counts at day 28 from control, infected untreated, infected treated with gentamycin and infected treated with SSAgNPs. Values represent mean ± SD Values are statistically significant at **p < .01, ***p < .001.
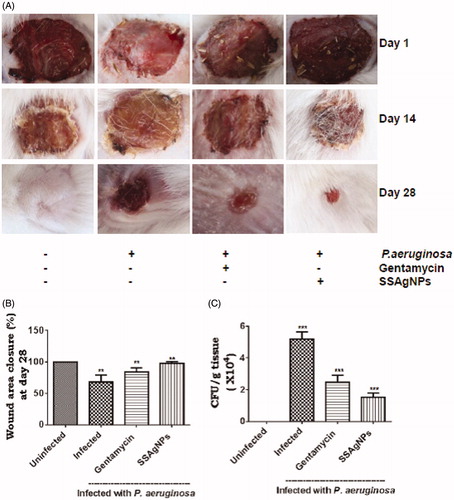
Microbial load in treated wounds
In vivo efficacy of surfactin-stabilized AgNPs assessed by applying 0.1% surfactin-stabilized AgNPs, in a parallel trial with 0.5% gentamycin on topical application in wounds infected with P. aeruginosa elicited convincing results. The change in bacterial counts in infected wounds with and without surfactin stabilized AgNPs; gentamycin is shown in . At day 28, the bacterial counts in surfactin-stabilized AgNPs-treated group remained significantly lower (p < .001) than that of the untreated wounds and wounds borne on animals in gentamycin-treated group.
Pro, anti-inflammatory cytokine, MMPs, TIMPs, growth factors, and collagen gene expression at the wound site
The mRNA expression of the wound-healing signaling molecules such as pro-inflammatory cytokines (TNF-α, IL-1β and IL-6) and anti-inflammatory cytokine (IL-10) measured showed sharp differences in various groups of animals. Other important factors, such as matrix metalloproteinases (MMP-2 and MMP-9) and its tissue inhibitor of matrix metalloproteinases (TIMP-1 and TIMP-2), evaluated also reflected a sharper deviation. A scrutiny of different growth factors involved such as transforming growth factor β-1 (TGF-β1) and vascular endothelial growth factor-A (VEGF-A), and Collagen-1 (COL-1) in the uninfected, infected untreated, and infected treated with gentamycin groups clearly showed a different profile from that of surfactin-stabilized AgNPs-treated wounds (). Analysis made in the 28th day wound mRNA expression of TNF-α, IL-1β, IL-6, and MMP-2, MMP-9 increased (p < .05) in P. aeruginosa-infected wounds compared with uninfected wounds. Whereas infected wounds from surfactin-stabilized AgNPs-treated groups contained low levels of the pro-inflammatory cytokines (p < .05) and matrix metalloproteinases (p < .01) as equal to uninfected wounds but superior than gentamycin. On the contrary, surfactin-stabilized AgNPs treatment induced the higher expression of the IL-10 (p < .05), TIMP-1, TIMP-2 (p < .01), and TGF-β1, VEGF-A (p < .05), and COL-1 (p < .01) in P. aeruginosa-infected wounds. Equated with untreated P. aeruginosa-infected wounds measured for the anti-inflammatory cytokine, tissue inhibitor of matrix metalloproteinases, growth factors, and collagen-1 showed a decrease (p < .05) in diabetic wounds of animals ().
Figure 8. (A) mRNA expressions and (B) fold changes of TNF-α, IL-1β, IL-6, IL-10, TIMP-1, TIMP-2, MMP-2, MMP-9, TGF-β1, VEGF-A, and COL-1 from control, infected untreated, infected treated with gentamycin, and infected treated with SSAgNPs at day 28 were analyzed by RT-PCR. GAPDH was used as an internal control. Values represent mean ± SD. Values are statistically significant at *p < .05, **p < .01.
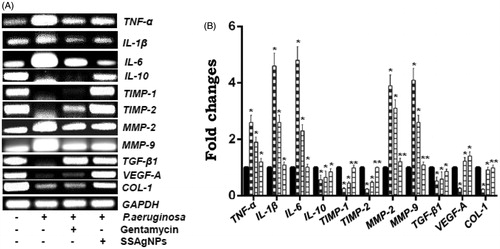
Histological analysis
Microscopic investigation of tissue samples undertaken to establish the re-epithelialization process confirms the effectiveness of surfactin-stabilized AgNPs treatment on diabetic wound infected with P. aeruginosa. depicts the infected wounded tissue with and without treatments after the 28th day. Compared with uninfected wounds which showed high blood capillary, fibroblast, collagen deposition, and re-epithelialization, the untreated P. aeruginosa-infected wounds and infected wounds those treated with gentamycin showed histological features of incomplete healing with low blood capillary, fibroblast, and collagen deposition. That wounds treated with surfactin-stabilized AgNPs showed better blood capillary, fibroblast, collagen deposition, and re-epithelialization as equal to that seen in uninfected wounds clearly indicate the ability of surfactin-stabilized AgNPs to mitigate infection better than the standard antibiotic gentamycin.
Neutralization of LPS toxicity in wounds
In the study designed to examine the endotoxin neutralizing activity of surfactin stabilized AgNPs in skin wounds, it was evident that LPS increased the expression of the pro-inflammatory cytokine TNF-α (p < .05) in wounds. Conversely, surfactin-stabilized AgNPs decreased the transcription of TNF-α level (p < .05) in LPS-pretreated wounds demonstrating the endotoxin neutralizing activity of surfactin-stabilized AgNPs synthesized from B. brevis KN8(2) cell-free culture filtrate which contained the endotoxin neutralizing lipopeptide surfactin ().
Discussion
Diabetic foot infection is a frequent clinical problem in diabetic patients which needs to be understood better and addressed with utmost attention. The disease demands proper management failing which the extrication of limb becomes inevitable and amputation brings down the life span of individual as quickly as cancer. The most important aim of diabetic foot infection therapy is to make the patient avoid the limb loss and that survival of the patient is not put to any risk due to terminal infections [Citation14]. Infections in the diabetic wound are polymicrobial in origin with predominant representation from the Gram-negative organisms in tropical Asian countries and in India, unlike the temperate western geographic regions. Pseudomonas aeruginosa the dreaded Gram-negative bacterium associated is of major concern in diabetic foot infection [Citation15,Citation16]. Although the infecting pathogen tends not to invade deeper tissues, it releases a variety of virulence factors, including endotoxin (LPS) causing significant wound deterioration and hence prolongs the ulcer to heal [Citation17,Citation18].
Owing to surface dwelling habit of P. aeruginosa, dermatologists mostly endorse topical application as a possible option for the treatment to avoid adverse effects that would emanate from systemic treatment [Citation19]. However, the possibilities that certain topical antimicrobial agents applied to the wound stimulate an enhanced release of LPS in the areas of the wound which may interfere with wound healing [Citation20–23]. Therefore, to be ideally effective, the control agent should not only be killing the causative bacteria but also must exert an endotoxin neutralizing activity [Citation24]. So, this present study focuses on finding solution to inadvertent adverse effects caused by P. aeruginosa and its LPS offers to the synthesis, characterization of AgNPs using the rhizosphere soil bacterium B. brevis KN8(2) cell-free culture filtrate which contained the endotoxin neutralizing lipopeptide surfactin as confirmed by LC–ESI–MS/MS. The results obtained using high-throughput techniques such as UV–Visible spectroscopy, FESEM, DLS, zeta potential, and XRD measurements of the biosynthesized AgNPs are much in conformity to the earlier results [Citation25–30]. The size of silver nanoparticles produced by B. brevis KN8(2), additionally confirmed using DLS techniques, confers a larger particle size than XRD indicating a higher hydrodynamic radius which could be due to the presence of capping agents. Average zeta potential of silver nanoparticles measured to be −36.6 mV also suggesting that negatively charged components of surfactin molecules have been capped in the surface of silver nanoparticles imparting a net negative charge. It could be well assumed that the biomolecules present in extracellular filtrate are not only involved in the synthesis but also in providing the surface coating of silver nanoparticles that makes the particles stable [Citation31–34]. Surfactin, a cyclic lipopeptide present in the culture exudates of B. brevis, has been demonstrated for the first time in the synthesis and the stabilization of silver nanoparticles. Furthermore, the biogenic surfactin-stabilized silver nanoparticles were tested and found to neutralize the toxic effects of lipopolysaccharide of P. aeruginosa in diabetic skin injury in addition with anti-pseudomonal activity is the novel findings of this present study.
The wound repair activity of surfactin-stabilized AgNPs in P. aeruginosa-infected streptozotocin-induced diabetic wounds in Swiss albino mice was visibly stepped up in mice treated with surfactin-stabilized AgNPs. In diabetes mellitus, the inability of infected wounds to heal is associated with an abnormality in one or more phases of the healing process [Citation35]. In the present study, it is observed that defective wound repair in infected diabetic mice is associated with higher concentration of pro-inflammatory cytokines and matrix metalloproteinases. Anti-inflammatory cytokine, tissue inhibitor of matrix metalloproteinases (TIMPs), growth factors, and collagen synthesis were seen to decline confirming that the response at the inflammatory phase is more crucial than the successive one. Surfactin-stabilized AgNPs-treated-infected mice showed a higher production of anti-inflammatory cytokine, tissue inhibitor of matrix metalloproteinase (TIMPs), growth factors, and collagen-1 (COL-1). In contrast, the levels of pro-inflammatory cytokine and matrix metalloproteinases showed a decline only to earlier reports on anti-inflammatory curative potential of AgNPs [Citation36]. Preclinical trials in Swiss albino mice clearly established the effectiveness of the substance of bacterial origin which interestingly is more convincing than even the standard antibiotic gentamycin used as a positive control. While this observation itself is good enough to evoke interest among pharmacists the finding that surfactin stabilized AgNPs which synthesized from B. brevis KN8(2) which is ideal and novel therapeutic agent for biomedical applications. Surfactin-stabilized AgNPs not only kills the bacteria but also reduces the toxic effects of LPS on wounds in terms of suppressing the pro-inflammatory cytokine such as TNF-α which induced by LPS. TNF-α is an auto-stimulative cytokine which can induce other inflammatory cytokines including IL-1β and IL-6 and inhibit the production of tissue inhibitor of matrix metalloproteinases (TIMP1 and TIMP-2) paving way for stimulated synthesis and secretion of high concentrations of matrix metalloproteinases (MMP-2 and MMP-9). Excessive matrix metalloproteinases degrade multiple growth factors (TGF-β1 and VEGF-A) and matrix proteins (COL-1) that are essential for granulation tissue formation which take parts in wound-healing processes are disrupted or delayed and non-healing chronic wounds develop rendering the treatment of Gram-negative bacilli infection ineffective. So, the management of Gram-negative bacilli infection, therefore, should ideally be based not only on killing the causative bacteria but also on neutralizing the LPS. Results gathered in this present study explains that topically applied surfactin stabilized AgNPs decreased the expression of pro-inflammatory cytokine such as TNF-α in both P. aeruginosa-infected and LPS-treated wounds in diabetic mice leads to increase in the rate of wound repair in P. aeruginosa-infected wounds by modulating pro-, anti-inflammatory cytokines, MMPs, TIMPs, growth factors, COL-1, and reduced the toxic effects of LPS in diabetic wounds thus provide a new and effective therapeutic intervention in dealing with diabetic foot infection.
Conclusion
To conclude, the present study documents the first ever synthesis and characterization of AgNPs from extracellular exudates which contained surfactin as reducing and stabilizing agents of B. brevis KN8(2). The surfactin-stabilized AgNPs accelerated wound repair in P. aeruginosa-infected diabetic mice. In addition to anti-pseudomonal activity, surfactin stabilized AgNPs is seen to have the ability to reduce LPS induced pro-inflammatory response in wounds and the surfactin which is bound in AgNPs may be responsible for endotoxin neutralizing activity. On the basis of these findings, it is concluded that the topical application of surfactin-stabilized AgNPs synthesized from B. brevis KN8(2) can certainly provide a dependable novel therapeutic treatment to heal Gram-negative bacilli infected diabetic wounds.
Disclosure statement
The authors declare that they have no conflict of interest.
References
- Peters BM, Rizk MAJ, O’May GA, et al. Polymicrobial interactions: impact on pathogenesis and human disease. Clin Microbiol Rev. 2012;25:193–213.
- Intine RV, Sarras MP, Jr. Metabolic memory and chronic diabetes complications: potential role for epigenetic mechanisms. Curr Diab Rep. 2012;12:551–559.
- Watters C, DeLeon K, Trivedi U, et al. Pseudomonas aeruginosa biofilms perturb wound resolution and antibiotic tolerance in diabetic mice. Med Microbiol Immunol. 2013;202:131–141.
- Thomson K, Ward L, Wrobel S. Method for removing endotoxin from proteins, European patent application. EP 2 650 302 A1; 2013.
- Wang SH, Sun ZL, Guo YJ, et al. Methicillin-resistant Staphylococcus aureus isolated from foot ulcers in diabetic patients in a Chinese care hospital: risk factors for infection and prevalence. J Med Microbiol. 2010;59:1219–1224.
- Rafehi H, El-Osta A, Karagiannis TC. Epigenetic mechanisms in the pathogenesis of diabetic foot ulcers. J Diabetes Complicat. 2012;26:554–561.
- Gariani K, Uckay I, Lipsky BA. Managing diabetic foot infections: a review of the new guidelines. Acta Chir Belg. 2014;114:7–16.
- Jaiswal S, Duffy B, Jaiswal AK, et al. Enhancement of the antibacterial properties of silver nanoparticles using beta-cyclodextrin as a capping agent. Int J Antimicrob Agents. 2010;36:280–283.
- Shahverdi AR, Fakhimi A, Shahverdi HR, et al. Synthesis and effect of silver nanoparticles on the antibacterial activity of different antibiotics against Staphylococcus aureus and Escherichia coli. Nanomed Nanotechnol Biol Med. 2007;3:168–171.
- Durán N, Marcato PD, Durán M, et al. Mechanistic aspects in the biogenic synthesis of extracellular metal nanoparticles by peptides, bacteria, fungi, and plants. Appl Microbiol Biotechnol. 2011;90:1609–1624.
- Korbekandi H, Iravani S, Abbasi S. Optimization of biological synthesis of silver nanoparticles using Lactobacillus casei subsp. Casei. J Chem Technol Biotechnol. 2012;87:932–937.
- Oves M, Khan MS, Zaidi A, et al. Antibacterial and cytotoxic efficacy of extracellular silver nanoparticles biofabricated from chromium reducing novel OS4 strain of Stenotrophomonas maltophilia. PLoS One. 2013;8:e59140.
- Veron W, Lesouhaitier O, Pennanec X, et al. Natriuretic peptides affect Pseudomonas aeruginosa and specifically modify lipopolysaccharide biosynthesis. FEBS J. 2007;274:5852–5864.
- Schaper NC, Dryden M, Kujath P, et al. Efficacy and safety of IV/PO moxifloxacin and IV piperacillin/tazobactam followed by PO amoxicillin/clavulanic acid in the treatment of diabetic foot infections: results of the RELIEF study. Infection. 2013;41:175–186.
- Ramakant P, Verma AK, Misra R, et al. Changing microbiological profile of pathogenic bacteria in diabetic foot infections: time for a rethink on which empirical therapy to choose? Diabetologia. 2011;54:58–64.
- Watters C, Everett JA, Haley C, et al. Insulin treatment modulates the host immune system to enhance Pseudomonas aeruginosa. Wound Biofilms. Infect Immun. 2014;82:92–100.
- Edwards R, Harding KG. Bacteria and wound healing. Curr Opin Infect Dis. 2004;17:91–96.
- Löffler MW, Schuster H, Bühler S, et al. Wound fluid in diabetic foot ulceration: more than just an undefined soup? Int J Low Extrem Wounds. 2013;12:113–129.
- Lipsky BA, Holroyd KJ, Zasloff M. Topical versus systemic antimicrobial therapy for treating mildly infected diabetic foot ulcers: a randomized, controlled, double-blinded, multicenter trial of pexiganan cream. Clin Infect Dis. 2008;47:1537–1545.
- Lipsky BA. Evidence-based antibiotic therapy of diabetic foot infections. FEMS Immunol Med Microbiol. 1999;26:267–276.
- Majerle A, Kidric J, Jerala R. Enhancement of antibacterial and lipopolysaccharide binding activities of a human lactoferrin peptide fragment by the addition of acyl chain. J Antimicrob Chemother. 2003;51:1159–1165.
- Rosenfeld Y, Papo N, Shai Y. Endotoxin (lipopolysaccharide) neutralization by innate immunity host-defense peptides peptide properties and plausible modes. J Biol Chem. 2006;281:1636–1643.
- Sahu K, Sharma M, Bansal H, et al. Topical photodynamic treatment with poly-l-Lysine-chlorin p6 conjugate improves wound healing by reducing hyperinflammatory response in Pseudomonas aeruginosa-infected wounds of mice. Lasers Med Sci. 2013;28:465–471.
- Kömerik N, Wilson M, Poole S. The effect of photodynamic action on two virulence factors of Gram-negative bacteria. Photochem Photobiol. 2000;72:676–680.
- Ganesh BMM, Gunasekaran P. Production and structural characterization of crystalline silver nanoparticles from Bacillus cereus isolate. Colloids Surf B Biointerfaces. 2009;74:191–195.
- Shaligram NS, Bule M, Bhambure R, et al. Biosynthesis of silver nanoparticles using aqueous extract from the compactin producing fungal strain. Process Biochem. 2009;44:939–943.
- Kathiresan K, Alikunhi NM, Pathmanaban S, et al. Analysis of antimicrobial silver nanoparticles synthesized by coastal strains of Escherichia coli and Aspergillus niger. Can J Microbiol. 2010;56:1050–1059.
- Fayaz AM, Balaji K, Girilal M, et al. Biogenic synthesis of silver nanoparticles and their synergistic effect with antibiotics: a study against Gram-positive and Gram-negative bacteria. Nanomed Nanotechnol Biol Med. 2010;6:103–109.
- Kaviya S, Santhanalakshmi J, Viswanathan B, et al. Biosynthesis of silver nanoparticles using Citrus sinensis peel extract and its antibacterial activity. Spectrochim Acta A Mol Biomol Spectrosc. 2011;79:594–598.
- Jain N, Bhargava A, Majumdar S, et al. Extracellular biosynthesis and characterization of silver nanoparticles using Aspergillus flavus NJP08: a mechanism perspective. Nanoscale. 2011;3:635–641.
- Plaza DO, Gallardo C, Straub YD, et al. Biological synthesis of fluorescent nanoparticles by cadmium and tellurite resistant Antarctic bacteria: exploring novel natural nanofactories. Microb Cell Fact. 2016;15:76.
- Siddiqi KS, Husen A. Fabrication of metal nanoparticles from fungi and metal salts: scope and application. Nanoscale Res Lett. 2016;11:98.
- Venil CK, Sathishkumar P, Malathi M, et al. Synthesis of flexirubin-mediated silver nanoparticles using Chryseobacterium artocarpi CECT 8497 and investigation of its anticancer activity. Mater Sci Eng: C. 2016;59:228–234.
- Palanisamy S, Rajasekar P, Vijayaprasath G, et al. Green route to synthesis silver nanoparticles using Sargassum polycystum and its antioxidant and cytotoxic effects: an in vitro analysis. Mater Lett. 2017;189:196–200.
- Hamed S, Ullmann Y, Masoud M, et al. Topical Erythropoietin promotes wound repair in diabetic rats. J Invest Dermatol. 2010;130:287–294.
- Wong KKY, Cheung SOF, Huang L, et al. Further evidence of the anti-inflammatory effects of silver nanoparticles. Chem Med Chem. 2009;4:1129–1135.