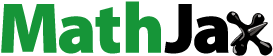
Abstract
This research study is to develop an easy and eco-friendly method for the synthesis of AgNPs using aqueous extract of endophytic fungi, Cladosporium species (CsAgNPs) and investigated the effects of antioxidant, anti-diabetic and anti-acetylcholinesterase (AChE), anti-butyrylcholinesterase (BChE) activity. Cladosporium species-mediated silver nanoparticles were characterized by UV–Vis spectrophotometer, Fourier transform infrared spectroscopy (FTIR), X-ray diffraction (XRD) and energy dispersive X-ray spectroscopy (EDX). The aqueous extract of Cladosporium species has shown the presence of carbohydrates, tannin, phenolic glycosides, terpenoids, alkaloids, phenol and anthraquinones. At 438 nm conformed the absorbance of AgNPs. The SEM result confirms that size, morphology and high density of the synthesized nanoparticles with huge disparity in the particle size distribution. The FTIR analysis confirmed the important biological compounds responsible for reduction of silver. Strong absorption property of AgNPs was studied by EDX. In antioxidant activity, CsAgNPs showed the involvement of NADPH-dependent reductase in the formation of AgNPs. The AgNPs has reduced the activity of α-amylase, α-glucosidase and dipeptidyl peptidase IV in vitro antidiabetic activity. The CsAgNPs showed significant glucose uptake in 3T3L1 cell line. The AgNPs have shown excellent inhibitory activity against AChE and BChE. To our best knowledge, this is the first on the synthesis of silver nanoparticles using endophytic fungi, Cladosporium species isolated from healthy leaf of Loranthus micranthus. Hence, to validate our results the in vivo animal studies at molecular level are needed to develop an antioxidant, anti-diabetic and anti-cholinesterase agent.
Introduction
In recent years, the nanotechnology has great commercial applications in the areas, such as catalysis, electronics, energy, textile, medicine, physics, etc. Out of all metal nanoparticles, the silver nanoparticles have specific applications in related to shape, size and morphologically. Synthesis of physical and chemical methods of nanoparticles resulted in low production rate, high expenditure and release of toxic chemicals and for large-scale synthesis have many drawbacks. The biological synthesis of AgNPS provides alternate promising advantage, such as environmental friendly, easy to scale up for large-scale synthesis [Citation1]. Biological agents (plants, fungi, bacteria and virus) are able to absorb and accumulate metals can be used as reducing agent and controls the nanostructural topography of the metal ions. Endophytic fungi are more ideal for the synthesis of nanoparticles; they can easily form large biomass within short period in laboratory conditions. These endophytic fungi can withstand agitation, flow pressure and conditions of bioreactor. Endophytic micro-organisms produce biologically important substances having use in modern medicine, agriculture, anti-diabetic, immunosuppressant, antioxidant, anticancer, etc. The AgNPs synthesized from endophytic fungi, Aspergillus versicolor [Citation2], Pestalotiopsis microspore [Citation3], Cladosporium cladosporioides [Citation4] have exhibited antioxidant property. No reports are available on the anti-diabetic activity of endophytic fungi mediated synthesized AgNPs. There are reports available plant-mediated synthesized AgNPs shown anti-diabetic activities of Punica granatum [Citation5], Psoralea corylifolia [Citation6]. No reports on anti-cholinesterase activity of endophytic fungal mediated synthesis of AgNPs. The Mellittia pinnata flower-mediated synthesized silver nanoparticles have shown anti-cholinesterase activity [Citation7]. Hence, this research aims to cost-effective, environmental friendly AgNPs using aqueous extract of Cladosporium species, an endophytic fungus of parasitic plant – Loranthus micranthus to explore its antioxidant, anti-diabetic and anticholinesterase activity potential.
Materials and methods
The silver nitrate (AgNO3) (99.98%) reagent grade was used as precursor (Sigma-Aldrich, St. Louis, MO) for the study.
Biosynthesis of silver nanoparticles with Cladosporium species extract
The endophytic fungi, Cladosporium species was collected from stock culture of Department of Biotechnology, Dayananda Sagar College of Engineering, Bengaluru, Karnataka, India and was used as reducing agent. The Cladosporium species was mass cultured by using Potato Dextrose Broth for 15 d at room temperature (26 ± 2 °C). After incubation, the Cladosporium species mycelia were dried by exposure to sunlight. Five gram of dried mycelia mat of Cladosporium species was milled and transferred to a 50 ml conical flask with 20 ml of sterilized distilled water. The aqueous extract of Cladosporium species synthesized AgNPs were investigated for the presence of phytochemicals, namely carbohydrates, alkaloids, saponins, proteins, amino acids, phenol, diterpenes, tannins and phytosterols by following standard biochemical methods [Citation8]. The Cladosporium species solution was heated to 100 °C for 10 min. The extract obtained was mixed with 10 ml of AgNO3 (5 mM) solution at room temperature. After 1 h, observed the colour change of the post-mixtures from light green into dark brown.
Cladosporium species silver nanoparticles (CsAgNPs) structural characterization
UV–visible spectra analysis
The reduction of pure silver ions of the reaction medium at 5 h and diluted with distilled water was measured. Analysis was carried out with UV–visible spectrophotometer UV-2450 (Shimadzu, Kyoto, Japan).
Analysis of silver nanoparticles by SEM
Analysis was carried out with Hitachi S-4500 scanning electron microscope (SEM; Carl Zeiss, Oberkochen, Germany) to analyse morphological character of the sample. Thin film samples were prepared on a carbon coated copper grid by dropping small amount of the sample on the grid, the extra solution was removed using a blotting paper and films were allowed to dry by subjected under a mercury lamp for 5 min.
X-ray diffraction studies
The X-ray diffraction (XRD) technique helps to check the formation and quality of CsAgNPs, and the pattern measured by drop coating film on glass substrate was recorded in a wide range of Bragg angles θ at a scanning rate of 2 min−1, carried out on a Philips PW 1830 instrument that was operated at a voltage of 40 kV and current 30 mA with Cu-Kα radiation (1.5405 Å).
Detection of functional group in CsAgNPs extract
Fourier transform infrared (FTIR) spectral measurements were carried out to identify the potential biomolecules in Cladosporium species extract which is responsible for reducing and capping the bioreduced silver nanoparticles. Fourier transform infrared spectroscopy (FTIR) was performed on Thermo Scientific™ Nicolet iS™50 FTIR Spectrometer (Thermo Fisher Scientific Co., Waltham, MA) to detect the possible functional groups in biomolecules present in the plant extract.
Antioxidant activity of CsAgNPs
The antioxidant properties of the CsAgNPs were studied by using ferric ion reducing antioxidant power (FRAP) and 2,2-diphenyl-1-picrylhydrazyl (DPPH) assays.
FRAP assay
25 ml of acetate buffer (300 mM, pH 3.6) were mixed with 2.5 ml of 2,4,6-tris-(2-pyridyl)-S-triazine (TPTZ) solution (10 mM TPTZ in 40mMl−1 HCl) and 2.5 ml FeCl3 (20 mM) water solution. Each endophytic fungal extract and CsAgNPs sample, 150 μl and 0.5 mg ml−1, was dissolved in methanol, added by freshly prepared FRAP reagent (4.5 ml) and mixed. Absorbance was measured at 593 nm, the FRAP working solution was used as blank [Citation9,Citation10]. Ferrous sulphate (100–1000 μmol l−1) calibration curve was expressed in μmol Fe2+/mg dry weight extract. Each sample activity was compared to L-ascorbic acid.
DPPH radical assay
The effect of CsAgNPs extracts was estimated by the method presented by Liyana-Pathirana and Shahidi [Citation11]. The samples were freshly prepared by dissolving 24 mg DPPH in 100 ml ethanol and stored at −20 °C. Of, 150 μl of sample solution (10 μl of sample and 140 μl of distilled water) was allowed to react with 2850 μl of agent (190 μl of reagent and 2660 μl of distilled water) for 24 h, the experiment was done in dark condition and the absorbance was measured at 515 nm. The linear standard curve ranged from 25 to 800 μM of ascorbic acid (aa). Additional dilutions of DPPH values were measured over the linear range of the standard curve. 10 ml of stock solution was mixed with 45 ml of methanol to obtain a solution with the absorbance of 1.1 ± 0.02 units at 517 nm using spectrophotometer [Citation12]. All experiments were done in triplicate. The DPPH radical inhibition percentage of each sample was calculated according to standard formula. Briefly, 0.01274 g of AgNO3 was dissolved in 15 ml (5 mM) of deionized water.
where Acontrol is the absorbance of the DPPH radical with ethanol, Asample is the absorbance of DPPH radical with sample extract/standard.
Anti-diabetic activity of CsAgNPs extract
α-Glucosidase activity
A 36 µl of phosphate buffer solution, 30 ml CsAgNPs solution with various concentrations (10, 25, 50, 100 and 150 µg/ml) and 17 µl of 4-nitrophenyl–α-D glycopyranoside (PNPG) substrate as the concentration of 5 mM were put in 37 °C for 5 min. After 5 min, 17 µl of α-glucosidase solution 0.15 U/ml was added to each well to obtain a total volume of 100 ml. The mixture was incubated for 15 min the reaction was spotted by adding 100 µl of sodium carbonate 200 mM. Absorbance was measured at 405 nm using a microplate reader. Each test was repeated thrice [Citation13]. The calculation was done based on Elya et al. [Citation14].
α-Amylase assay
A 250 ml of 500 µg/ml CsAgNPs extract, 250 µl of starch 2.0% (w.v) and 250 µl of 1 U/ml α-amylase solution was homogeneously mixed into a test tube. After incubated at 20 °C for 3 min, 500 µl of colour reagent (dinitrosalicylic acid) was added to stop the enzymatic reaction. The mixture was kept into boiled water and 250 µl α-amylase 1 U/ml was added immediately. The mixture was heated up to 15 min. Further, the solution was removed from the heating process and cooked at room temperature (26 ± 2 °C) for 3 min. A 4500 αl aqua dest was added to obtain a total volume of 6000 µl. The solution was homogenized using a vortex. The α-amylase activity was determined at 540 nm using spectrophotometry to measure product absorbance (maltose) which reduces DNS. The produced absorbance was compared with a blank. Percent inhibition was calculated using the equation of Elya et al. [Citation14].
Dipeptidyl peptidase IV assay
A 25 µl CsAgNPs extract was added to 50 µl dipeptidyl peptidase (DPP-IV) (500 µg/ml). The mixture was incubated at 37 °C for 5 min. A 100 µl Gly-Pro-p-nitroanilide (GPPN) (2 mM) was added to the wells containing extract and enzyme incubated for 15 min. The reaction was terminated by adding 25 µl acetic acid glacial (25%). The absorbance was measured at λ = 405 nm [Citation14].
Adipocytes myocytes and hepatocytes namely 3T3L1, C2C12 and HepG2 were procured from National Centre for Cell Sciences, Pune, India and grown in media and maintained. Cell culture, differentiation and processing procedure were followed by standard procedure of Rao and Jamil [Citation31].
Assay for glucose uptake assay
Concentrations ranging from 0.034 to 33.4 μg were tested for insulin-mimetic and sensitization effects with or without insulin. The radiolabelled insulin was used to measure the changes in the glucose level uptake activity of cells in response to treatment. The 96 well microplate was used to perform the assay and the counts per minute were measured using a radioactive cell counter [Citation31]. To evaluate two sets of samples were used, i.e. glucose uptake in presence of insulin with CsAgNPs extract and without insulin with CsAgNPs extract with different concentrations 300, 30, 3 and 0.3 µg/well in triplicate for 18 h at 37 °C and 5% CO2, 100 µl of DMEM (Dulbecco’s Modified Eagles Serum Medium). Removed the medium and cells were incubated in Kreb’s Ringer Hepes buffer for 10 min at 37 °C followed by 5% CO2 for 1 min. The incubated insulin was selected at concentrations of 5, 10, 25, 50 and 100 µM in KRH buffer in triplicate. The cells were treated with 0.1, 1, 10, 100 and 500 µg/ml of CsAgNPs extract in triplicate at 37 °C for 20 min followed by 5% CO2 and measured the insulin mimetic activity. By adding 0.5 mm tritiated 2-deoxyglucose (100 µl/well) initiated the glucose uptake reaction in plate counting insulin with CsAgNPs extract or without CsAgNPs extract and plates were incubated at 37 °C for 15 min in a CO2 incubator. The ice-cold KRH buffer was added to stop the reaction after incubation period and the reaction mixture was kept at freezer for 2 min. Using ice-cold KRH buffer washed the reaction cells added 60 µl of KRH was added and plates were incubated at −20 °C for overnight. The cells were scraped and transferred to iso-black plate and added 160 µl of scintillation vials were used to measure glucose uptake in triplicate and expressed as percentage.
Anti-acetyl cholinesterase activity
Acetylcholinesterase activity was carried out by using a 96-well microplate reader according to Ellman’s method. In the 96-well plates, at 37 °C, 70 mM phosphate buffer (Na2HPO4/NaH2PO4, pH 7.4) containing the ChE enzyme (10 ml volume, diluted 100 times in phosphate buffer, pH 7.4), DTNB (5,50-dithiobis-(2-nitro-benzoic acid)) (104 M concentration) and ATCh (1.35 × 10−4 M concentration) was added and the absorbance was measured at 412 nm for 5 min and three replicates were maintained for each experiment. Any increase in absorbance due to the spontaneous hydrolysis of the substrate was corrected by subtracting the rate of the reaction before the addition of the enzyme from the rate of the enzyme reaction. The percentage of inhibition was calculated by comparison of sample rates to a blank [Citation15].
Results and discussion
Phytochemical analysis
The Cladosporium species yielded biologically important phytochemicals, such as carbohydrates, phenolic glycosides, terpenoids, alkaloids, phenol and anthraquinones, but flavonoids and saponins were absent () [Citation16].
Table 1. Phytochemical analysis of endophytic fungi Cladosporium species extract.
UV–visible spectroscopy
The extracted colour changed to brown due to addition of 1 mM AgNO3 within 30 min and no further colour change was observed after 24 h. No colour formation was observed in without addition of Cladosporium species extract (). Due to the presence of active phytochemicals in the extract of Cladosporium species are responsible for reducing silver metal to AgNPs due to excitation of surface plasmon resonance of synthesized AgNPs. The incubated CsAgNPs solution absorption spectra was studied at 438 nm in 30 min, it confirmed the formation of AgNPs (). Our finding is consistent with reports of Li et al. [Citation17] and Rajakumar et al. [Citation7]. The intensity of the absorption peak at 438 nm was increased with time of incubation.
X-ray diffraction analysis
In the XRD analysis, the crystalline nature of the CsAgNPs was observed. The XRD pattern exhibited diffraction peaks corresponding to [111], [200], [220], [311] and [222] appearing at 2θ (). These peaks indicate the crystalline (fcc) nature of the AgNPs and are in agreement with the database of the Joint Committee on Powder Diffraction Standards (JCPDS No. 04–0783). Additional intense peaks at 2θ angles of CsAgNPs are because of the involvement of the AgNO3 which was used for the synthesis of silver nanoparticles [Citation7,Citation17]. The broadening of Bragg’s peaks indicates the formation of nanoparticles and Debye–Scherrer’s equation calculates the mean size of the silver nanoparticles was 24 nm. The XRD patterns suggest that crystallization of the bioorganic phase occurs on the surface of AgNPs. Our results are confirmatory with the findings of Li et al. [Citation17] and Rajakumar et al. [Citation7].
FTIR
FTIR measurement was used to find out the interaction of silver ions with the bioactive components present in the fungal extracts that are responsible for stabilizing of AgNPs. The CsAgNPs FTIR showed absorption bands at 3401, 2916, 2844, 1618, 1381 and 1042 cm−1. The FTIR a spectrum also helps in identify different functional groups in the CsAgNPs extract. The peak 3401 cm−1 is strong alcohol O–H stretch with intermolecular bonded. The peak obtained 2916 cm−1 is strong N–H stretch, maybe amine salt, 2844 cm−1 the peak is medium C–H stretch it is aldehyde doublet, 1618 cm−1 is strong, stretch C–O may be conjugated ketone, 1381 cm−1 is medium C–H bending may be aldehyde and it was due to presence of aromatic group, 1041 cm−1 is strong, broad stretching (). Similar results are reported by Vinay et al. [Citation18]. The peak at 1042 cm−1 was strong are due to ether linkage and it indicates the presence of flavanones adsorbed on the surface of metal nanoparticles [Citation19]. The Cladosporium species extracts have shown many biologically important active compounds might be actively responsible and involved for reduction of Ag+ to Ag0 [Citation20].
SEM
The CsAgNPs morphology was examined using SEM and observed the reduction of AgNO3 with Cladosporium species extract (). The SEM result also depicted the morphology and size details of AgNPs with high-density Ag nanoparticles synthesized by Cladosporium species. The assembling, spherical and uniform AgNPs were observed.
EDX
depicts the energy dispersive a spectrum of the synthesized nanoparticles suggests the presence of silver as the main ingredient element. Metallic silver nanoparticles generally show a typically strong signal peak at 3 keV, due to surface Plasmon resonance [Citation21,Citation22]. provides information on qualitative information of biosynthesized AgNPs. The presence of elements, such as C, N, O, Na and Ag at different percentage was observed (). The synthesized silver nanoparticles showed strong absorption in the range 2.5–4 keV [Citation22–24].
Antioxidant activity
The antioxidant activity of CsAgNPs and aqueous extract of Cladosporium species was evaluated by FRAP and DPPH radical scavenging methods. The CsAgNPs showed potent antioxidant potential and the radical scavenging ability was increasing with the increment in the concentration. In FRAP assay, the CsAgNPs shows significant antioxidant activity was compared with Cladosporium species extract alone ( and ). The antioxidant activity of CsAgNPs was may be due to presence phytochemicals present in the extract. According to Kharat and Mendhulkar [Citation25], Manjunath et al. [Citation26] and Naveen et al. [Citation27], the antioxidant activity of AgNPs was due to presence of phenolic compound. But our endophytic aqueous extract exhibited important phytochemicals and all are these are reported as strong antioxidant molecular.
In vitro anti-diabetic activity
The CsAgNPs has potentially inhibited the activity of α-amylase, α-glucosidase and DPP-IV in vitro assay (). The inhibition of these enzymes is concentration dependent. The CsAgNPs decreased the levels of enzymes, which are responsible for catalysing the hydrolysis of complex carbohydrates and increased the consumption rate of glucose reported by Rajaram et al. [Citation28], Abideen and Sankar [Citation29] and Sengottaiyan et al. [Citation30]. A significant insulin sensitization activity was observed in 3T3L1 cell line followed by C2C12 and HepG2 cell lines at 3.34 µg of concentration of CsAgNPs (47%) (). At the same concentration of CsAgNPs significant insulin mimetic activity in 3T3L1 cell line followed by C2C12 and HepG2 cell lines () [Citation31].
Anti-cholinesterase activity
The CsAgNPs had shown significant inhibitory activity of both AChE and BChE than fungal extract (). The Alzheimer disease is associated with AChE deficiency and d-GNPs could be potential new acetylcholinesterase inhibitors. The nanoparticles interact or adsorption with AChE proteins leads to inhibition of their activity [Citation32] and it clearly indicates that nanoparticles have binding affinity to ChE. The interaction may occur due to lithophilicity of the nanoparticles and hydrophobicity environment of the enzyme ChE molecule [Citation7].
Table 2. Anticholinesterase activity of Cladosporium species-mediated synthesis of AgNPs, Cladosporium species extract and respective standard drugs.
Conclusion
The changes of AgNPs was observed in UV–vis spectra at 438 nm and colour change also noticed. The pattern of XRD revealed that the sample was composed of crystalline face-centred cubic (fcc) lattice structures of elemental silver. The important biological compounds and which are responsible for reduction of silver identified in FTIR method. The nanoparticles strong absorption was analysed by using EDX. The SEM result indicates that size, morphology and high density of the nanoparticles. The CsAgNPs showed potent antioxidant activity in two methods (DPPH and FRAP) and have radical scavenging property. The CsAgNPs inhibited the activity of α-amylase, α-glucosidase and DPP-IV and these enzymes are responsible for diabetes progression. The CsAgNPs also inhibited the activity of AChE and BChE activity significantly. Significant insulin sensitization and insulin mimetic activity was observed with CsAgNPs treated cell lines and confirms the reduction of glucose level in cells. Finally, the synthesis protocol is simple, economic and raw material is also cheap, so further in vivo experiments need to validate our outcomes of this work.
Disclosure statement
The authors report no declarations of interest. The authors alone are responsible for the content and writing of the article.
References
- Zhang XF, Liu ZG, Shen W, et al. Silver nanoparticles: synthesis, characterization, properties, applications and therapeutic approaches. Int J Mol Sci. 2016;17:1534.
- Netala VR, Kotakadi VS, Bobbu P, et al. Endophytic fungi isolate mediated biosynthesis of silver nanoparticles and their free radical scavenging activity and antimicrobial studies. 3 Biotech. 2016;6:132.
- Netala VR, Bethu MS, Pushpalatha B, et al. Biogenesis of silver nanoparticles using endophytic fungus Pestalotiopsis microspore and evaluation of their antioxidant and anticancer activities. Int J Nanomedicine. 2016;11:5683–5696.
- Hulikere MM, Joshi CG. Characterization, antioxidant and antimicrobial activity of silver nanoparticles synthesized using marine endophytic fungus-Cladosporium cladosporioides. Biochem Biophy. 2017;7. DOI:https://doi.org/10.1016/j.bbrep.2017.08.011
- Saratale RG, Shin HS, Kumar G, et al. Exploiting antidiabetic activity of silver nanoparticles synthesized using Punica granatum leaves and anticancer potential against human liver cancer cells (HepG2). Artif Cells Nanomed Biotech. 2018;46:211–222.
- Shankar K, Mohan GK, Hussain MA, et al. Green biosynthesis, characterization, in vitro antidiabetic activity, and investigational acute toxicity studies of some herbal mediated silver nanoparticles on animal model. Pharmacog Magaz. 2017;13:188–192.
- Rajakumar G, Gomathi T, Thiruvengadam M, et al. Evaluation of anti-cholinesterase, antibacterial and cytotoxic activities of green synthesized silver nanoparticles using from Millettia pinnata flower extract. Microb Pathog. 2017;103:123–128.
- Fransworth NR. Biological and phytochemical screening of plants. J Pharm Sci. 1996;55:225–227.
- Szollosi R, Varga SI. Total antioxidant power in some species of Labiatae (Adaptation of FRAP method). Acta Biol Szeged. 2002;46:125–127.
- Tomić A, Petrović S, Drobac M, et al. Antimicrobial and antioxidant properties of methanol extracts of two Athamanta turbith subspecies. Pharm Biol. 2009;47:314–319.
- Liyana-Pathirana CM, Shahidi F. Antioxidant activity of commercial soft and hard wheat (Triticum aestivum L.) as affected by gastric pH conditions. J Agric Food Chem. 2005;53:2433–2440.
- Katalinic V, Milos M, Kulisic T, et al. Screening of 70 medicinal plant extracts for antioxidant capacity and total phenols. Food Chem. 2006;94:550–557.
- Dewi RT, Iskandar YM, Hanafi M, et al. Inhibitory effect of Koji Aspergillus terreus on α-glucosidase activity and postprandial hyperglycemia. Pak J Biol Sci. 2007;10:3131–3135.
- Elya B, Handayani R, Sauriasari R, et al. Antidiabetic activity and phytochemical screening of extracts from Indonesia plants by inhibition of alpha amylase, alpha glucosidase and dipeptidyl peptidase IV. Pak J Biol Sci. 2015;18:279–284.
- Ellman GL, Courtney KD, Andres V. Jr, et al. A new and rapid colorimetric determination of acetylcholinesterase activity. Biochem Pharmacol. 1961;7:88–90.
- Osadebe PO, Omeje EO, Uzor PF, et al. Seasonal variation for the antidiabetic activity of Loranthus micranthus methanol extract. Asian Pacific J Trop Med. 2010;3:196–199.
- Li G, Dan H, Qian Y, et al. Fungus-mediated green synthesis of silver nanoparticles using Aspergillus terreus. Int J Mol Sci. 2012;13:466–476.
- Vinay SP, Chandrasekhar N, Chandrappa CP. Eco-friendly approach for the green synthesis of silver nanoparticles using flower extracts of Sphagneticola trilobata and study of antibacterial activity. Int J Phar Biol Sci. 2017;7:145–152.
- Shankar SS, Rai A, Ahmad A, et al. Rapid synthesis of Au, Ag, and bimetallic Au core-Ag shell nanoparticles using neem (Azadirachta indica) leaf broth. J Colloid Interface Sci. 2004;275:496–502.
- Zuas O, Hamim H, Sampora Y. Biosynthesis of silver nanoparticles using water extract of Myrmecodia pendans (Sarang Semutplant). Material Let. 2014;123:156–159.
- Das J, Das MP, Velusamy P. Sesbania grandiflora leaf extract mediated green synthesis of antibacterial silver nanoparticles against selected human pathogens. Spectrochim Acta Part A. 2013; 104:265–270.
- Anandalakshmi K, Venugobal J, Ramasamy V. Characterization of silver nanoparticles of green synthesis method using Pedalium murex leaf extract and their antibacterial activity. Appl Nanosci. 2015;6:399–408.
- Jagtap UB, Bapat VA. Green synthesis of silver nanoparticles using Artocarpus heterophyllus Lam. Seed extract and its antibacterial activity. Indian Crops Prod. 2013;46:132–137.
- Vijayakumar M, Priya K, Nancy FT, et al. Biosynthesis, characterization and anti-bacterial effect of plant mediated silver nanoparticles using Artemisia nilagirica. Indian Crops Prod. 2013; 41:235–240.
- Kharat SN, Mendhulkar VD. Synthesis, characterization, and studies on antioxidant activity of silver nanoparticles using Elephantopus scaber leaf extract. Mater Sci Eng C Mater Biol Appl. 2016;62:719–724.
- Manjunath HM, Joshi CG, Raju NG. Biofabrication of gold nanoparticles using marine endophytic fungus – Penicillium citrinum. IET Nanobiotechnol. 2017;11:40–44.
- Naveen KSH, Kumar G, Karthik L, et al. Extracellular biosynthesis of silver nanoparticles using the filamentous fungus Penicillium sp. Arch Appl Sci Res. 2010;2:61–167.
- Rajaram K, Aiswarya DC, Sureshkumar P. Green synthesis of silver nanoparticle using Tephrosia tinctoria and its anti-diabetic activity. Mater Lett. 2015;138:251–254.
- Abideen S, Sankar VM. In vitro screening of anti-diabetic and antimicrobial activity against green synthesized AgNO3 using seaweeds. Nanomed Nanotech. 2015;S6:001. DOI:https://doi.org/10.4172/2157-7439.S6-001
- Sengottaiyan A, Aravinthan A, Sudhakar C, et al. Synthesis and characterization of Solanum nigrum mediated silver nanoparticles and its protective effect on alloxan-induced diabetic rats. J Nanost Chem. 2016;6:41–48.
- Rao AP, Jamil K. Pharmacological evaluation of herbal extracts for their in vitro hypoglycemic activity. Int J Pharm Biosci. 2011; 2:408–416.
- Wang Z, Zhao J, Li F, et al. Adsorption and inhibition of acetylcholinesterase by different nanoparticles. Chemosphere. 2009; 77:67–73.