Abstract
Poly-l-lysine-coated superparamagnetic iron oxide nanoparticles (SPIONs-PLL) were prepared and used as a novel-carrier for the transfer of brain-derived neurotrophic factor (BDNF) into neural stem cells (NSCs) under the beneficial influence of an external magnetic field. Pro-BDNF, a gene from human brain cDNA libraries, was obtained by polymerase chain reaction and constructed in a mammalian expression vector (PSecTag2/HygroB). The nanoparticles (NPs) were examined using Fourier transform infrared spectroscopy, zeta potential, and Transmission electron microscopy. From the results, the levels of BDNF among the transfected and untransfected cells were 30.326 ± 5.9 and 5.85 ± 3.11 pg/mL, respectively, as detected by an ELISA method. Moreover, the enhanced green fluorescent protein vector was used to evaluate the gene expression efficiency for SPIONs-PLL as a non-viral carrier in NSCs. This was performed under the influence of a magnetic field and the transfection reagents (such as Lipofectamine 2000), which served as a positive control. The histological analysis revealed that the concentration of intracellular NPs was significantly higher than intercellular NPs. These results suggest that SPIONs-PLL can serve as a novel alternative for the transfection of BDNF-NSCs and could be used in gene therapy.
Keywords:
Introduction
Brain-derived neurotrophic factor (BDNF) belongs to the neurotrophin family of growth factors. It is synthesized in a pre-pro-form, consisting of 247 amino acids [Citation1], and has been known as a plasticity and synapse development regulator [Citation2]. Its precursor is initially synthesized as pro-BDNF, and later cleaved to generate the mature (m) BDNF [Citation3]. The synthesized Pro-BDNF is first packaged into secretory vesicles [Citation4] and later released either through activity-dependent or constitutive secretion pathway [Citation5]. Neural stem cells (NSCs) can auto-renew and differentiate into neurons, astrocytes, and oligodendrocytes [Citation6]. The modification of NSCs by the BDNF gene provides an efficient strategy and better therapeutic results than those of native NSCs after stroke [Citation7]. However, the gene transfection efficiency of NSCs has proven to be difficult by conventional viral transduction because of the disadvantages associated with the use of viral carriers, including immunogenicity, toxicity, mutagenicity, and oncogenicity. Hence, non-viral vectors such as cationic polymers, peptides, polyamines, dendrimers, and lipids are considered as proper candidates and have been applied in recent works [Citation8]. Silica nanoparticles (NPs) have been demonstrated for achieving the impermanent gene transfer in vivo as a non-viral vector systems [Citation9]. Some of non-viral carriers were designed and developed to overcome the challenges of ineffective DNA-endosomal capture and release from the carrier vector into the nucleus, and represent a promising methods for gene delivery purposes [Citation10]. Superparamagnetic iron oxide NPs (SPIONs) are widely used for biomedical applications, such as target gene delivery systems and gene therapy, It can be used also in cancer chemotherapy as drug carrier after coated with biocompatible polymers [Citation11,Citation12] SPIONs with unique magnetic properties are being actively investigated as magnetic resonance imaging contrast agents [Citation12], It can be properly designed and engineered to reach their target tissue with less nonspecific cellular interactions [Citation13,Citation14]. In addition, SPIONs showed a high labeling efficiency in different mammalian cell types [Citation15]. However, the aggregation of SPION has limited its application. Therefore, in this study, cationic poly-l-lysine (PLL) was used to checkmate SPION aggregation and to increase its biocompatibility [Citation16]. The use of cationic polymer-coated magnetic iron oxide NPs is considered an alternative approach for gene transfer [Citation17]. PLL is a biocompatible polypeptide and one of the cationic polymers first used for gene delivery [Citation18]. However, PLL shows a limited capacity for intracellular delivery due to the lack of an endosomal escape mechanism [Citation19]. To overcome these limitations, SPION-based materials can be added to enhance gene-transfer efficiency through the electrostatic interaction between the DNA and the nano-complex (SPIONs-PLL). The cell membrane can carry out endocytosis of nanocarrier vectors; SPIONs demonstrate excellent biocompatibility and superparamagnetism, which enable a long-term retention at target sites under the influence of a suitable magnet [Citation20]. In this study, SPION NPs were prepared, optimized, coated with PLL hydrobromide, and used as a carrier for the transfection of pSecTag2/Hygro B plasmids into NSCs.
Materials and methods
Cell culture
Bone marrow cells were aseptically collected from the femur and tibia of adult Sprague–Dawley rats (250–300 g) at the animal house of Razi Vaccine and Serum Research Institute, Tehran, Iran with the approval of the Ethics Committee of Tarbiat Modares University. The bone marrow was aspirated with a 5-mL syringe equipped with a 21 G needle into 5 mL DMEM (Gibco, Germany) containing 500 units of heparin. The collected cells were supplemented with 20% heated fetal bovine serum (FBS), 100 U/mL penicillin-100 μg/mL streptomycin, 25 ng/mL amphotericin B, and 2 mM l-glutamine and transferred into T-25 cm2 culture flasks (Nunc, Denmark). The cells were maintained in a 95% humidified incubator at 37 °C, with 5% CO2. After every 2–3 days, the cells were washed with PBS to remove all non-adherent floating cells and debris before replacing with fresh supplemented media. The cells were characterized by fibronectin as a bone marrow stromal cell (BMSC) marker [Citation21].
Generation of NSCs from BMSCs
The BMSCs of the third passage were harvested with 0.25% trypsin/1 mM EDTA and plated on six wells in neurosphere medium (DMEM:F12 1:1 medium containing 50 U/mL penicillin, 50 mg/mL streptomycin, 2% B-27 supplement, 4 ng/mL heparin, 20 ng/mL epidermal growth factor (EGF), and 20 ng/mL basic fibroblast growth factor (bFGF). The cell culture reagents and the human recombinant bFGF were purchased from Invitrogen (Germany), while the human recombinant EGF was obtained from Sigma (USA). The neurospheres were trypsinized, and the cells were collected, centrifuged, and plated with DMEM:F12 1:1 medium supplemented with 2% B-27 and 5% FBS.
Immunocytochemistry
The BMSC purity was evaluated using fibronectin as a specific BMSC marker. The cells were plated on 60 mm dishes (Nunc, Denmark) containing 0.1% gelatin-coated coverslips until they adhered. The adhered cells were washed thrice with PBS, fixed in 4% formaldehyde for 30 min, washed again with PBS, and permeated with 0.3% Triton X-100 for 1 h. Non-specific antibody reaction was quenched normal goat serum (10%) for 30 min at room temperature (RT), then, incubated with monoclonal mouse anti-fibronectin, anti-CD90, anti-CD105 antibodies (Abcam, UK) overnight at 4 °C. The samples were washed twice with PBS, incubated with rabbit anti-mouse FITC-conjugated antibody (Abcam, UK, 1:100) for 2 h at RT before washing twice with PBS for 15 min. The washed samples were counterstained with ethidium bromide for 1 min (for nuclei demonstration), washed with PBS, and observed under an inverted fluorescence microscope (Olympus IX71, Japan). For the immune staining, the NSCs were fixed with paraformaldehyde (4%) (Merck, Germany) and stained with nestin. Neurofilament (NF) 68 and NF200 were treated the same way as the BMSCs.
Preparation of SPIONs
Ammonium hydroxide (NH4OH), ferrous chloride (FeCl2 4H2O), and ferric chloride (FeCl3 6H2O) were obtained from Sigma-Aldrich (Germany). All aqueous solutions were formulated in deionized water. The SPIONs (Fe3O4) were prepared via a chemical co-precipitating method using an aqueous solution of ferrous and ferric salts [Citation12]. Briefly, 1 M FeCl3(10 mL) and 2 M FeCl2(5 mL) were added to a 100-mL beaker. The solution was stirred and purged with N2 gas before adding 50 mL of NH4OH solution (25%) drop wise over a period of 5 min under vigorous stirring until a pH value of 11 was achieved. The formation Fe3O4 and its separation from the solution were facilitated via ultrasonication (Model B-12 Ultrasonic Bath, Wise Clean Co., Shelton, Connecticut, USA). The formed and separated Fe3O4 was washed with several changes of deionized water until the wash-off water pH was 7. The washed SPIONs were finally dried at 60 °C for 48 h. The concentration of the NPs suspension was estimated to be 5 mg/mL. PLL hydrobromide (Sigma, Catalog No. P1524, USA) at a stock concentration, 1.5 mg/mL was used as a transfection agent.
Characterization of SPIONs and PLL coating
The NPs were characterized using Transmission electron microscopy (TEM; LEO 906, USA) and Fourier transform infrared (FTIR) spectroscopy (FTS-3000 spectrometer: Bio-Rad, USA). The NPs were characterized to demonstrate the successful coating of the surface over a wavelength range of 500–4000 cm−1. The zeta potentials of the coated and uncoated SPIONs were also determined using Zetasizer NANO-ZS (Malvern Instruments, UK). All measurements were carried out at pH 7.4.
Prussian blue staining
The cultured NSCs were plated in six wells containing coverslips coated with 0.1% gelatin. The plates were incubated overnight with the SPIONs-PLL complex and later washed thrice with PBS. The washed cells were fixed with 4% paraformaldehyde for 30 min, washed again with PBS, and incubated with 5% potassium ferrocyanide in 5% hydrochloric acid for 30 min. After 30 min, the cells were washed again and counterstained with safranin stain (Sigma, Germany) before observing the intracellular iron oxide in the labeled cells under a light microscopy.
Vector construction (sub-cloning)
Competent cells (Escherichia coli DH5α bacteria) were used as the carrier vector of the human pre-pro-BDNF for the transformation of the pCMV-SPORT6 (Open Biosystems, USA). The expression vector (pSecTag2/HygroB, Invitrogen) plasmids were separately purified using a plasmid purification kit (PureLinkHiPure, Invitrogen). The pCMV-SPORT6 vector was employed as an amplification template to amplify the human pro-BDNF with the Pfu enzyme using 2 primers that contains specific cleavage sites for the restriction enzymes (EcoRV and XhoI). The PCR product (human pro-BDNF) was purified using a PCR purification kit (MinElute, Qiagen) and digested overnight in separate microtubes at 37 °C with the pSecTag2/Hygro B plasmid (Invitrogen, Germany) using the same restriction enzymes (EcoRV and XhoI). The electrophoresed digested vector (on 1% agarose gel) was purified using a gel extraction kit (MinElute, Qiagen), while the human pro-BDNF waspurified using an enzymatic reaction Clean-Up Kit (Qiagen). Both the PCR product and the pSecTag2/HygroB vector were digested via ligation. The ligation process was done with T4 DNA ligase (Fermentas kit). After the ligation, the reaction mixture was transformed into E. coli DH5α competent cells. The transformed/recombinant cells were seeded on agar plates under the cover of 100 µg/mL ampicillin. Positive colonies were confirmed by colony PCR. A PureLinkHiPure plasmid purification kit (Invitrogen) was used to extract the recombinant pSecTag2/HygroB-human pro-BDNF plasmid, while the accuracy of the human pro-BDNF gene DNA sequence on the pSecTag2/HygroB-human pro-BDNF plasmid was validated via sequencing. The data analysis and identification steps were performed using the Basic Local Alignment Search Tool, which can be freely assessed through the link http://www.ncbi.nlm.nih.gov.
Carrier preparation
PLL hydrobromide was employed as a transfection agent in this study. A stock PLL solution (1.5 mg/mL) was diluted with DMEM:F12 (1:1000) and mixed with 50 µg/mL of SPIONs for 60 min on a rotating shaker at RT [Citation22]. The final SPIONs and PLL concentrations in the solution were 25.0 and 0.75 µg/mL, respectively [Citation23].
Evaluation of NSC transfection efficiency by pEGFP-C1 vector
To evaluate the transfection efficiency in NSCs, the reporter plasmid pEGFP-C1 expressing EGFP was used as a reporter. The transfection was carried out using Lipofectamine 2000 (Invitrogen), a commercially available cationic liposome following the manufacturer’s instructions. This liposome served as a control under both magnetic and non-magnetic fields effect. Briefly, the NSCs were transfected with the pEGFP-C1 vector cells, and after 2 days post-transfection, the cells were observed under an inverted fluorescence microscope (Olympus). The total viable cells in the medium were checked by Trypan blue assay and fluorescent NSCs. After 24 h post-transfection, the number of positive cells (GFP-expressing) was determined and presented as a percentage, while the ratio of GFP-expressing cells versus the total number of cells was estimated using a fluorescence microscope.
MTT assay
To evaluate the viability of SPIONs-PLL-complex labeled rNSCs, 3–(4,5-dimethylthiazol-2-yl)-2,5-diphenyltetrazolium bromide (MTT) assay (Sigma-Aldrich, USA) was used following the manufacturer’s instruction. The NSCs were grown in 96-well plates at a concentration of 1 × 104 cells per well at 37 °C in 5% CO2. The SPIONs-PLL complexes were added in three different concentrations (25, 50, and 100 μg/mL), and after 72 h, the cells were washed twice with PBS before adding fresh 100 µL of DMEM: F-12 medium and 0.5 mg/mL of MTT reagent to each well. The non-labeled cells served as the control group. Afterward, the labeled NSCs were incubated for 4 h at 37 °C in 5% CO2. After 4 h, the medium was gently aspirated and replaced with 100 µg/mL of fresh DMSO. The absorbance of the dissolved formazan product was measured at a wavelength of 540 nm.
RT-PCR
The complete RNA of both the transfected and non-transfected NSCs were isolated with Ambion Kit (PureLink™ RNA Mini Kit, Ambion, USA, Catalog No. 12183020) according to the manufacturer’s instruction. Each extracted RNA was treated with DNase I enzyme (Invitrogen) and the absorbance of the treated RNA was determined at 260/280 nm and further by denaturing agarose gel electrophoresis. A Revert Aid First Strand cDNA Synthesis Kit (Fermentas) was used for the RT-PCR following the manufacturer’s recommendations. A control RT-minus reaction was set up in a similar without the reverse transcriptase enzyme. This control set up was used to check for any chance of contamination. A quantitative RT-PCR based on SYBR Green PCR Master Mix (Applied Biosystems) detection method was performed in a Step One apparatus (Applied Biosystems) using glyceraldehyde-3-phosphate dehydrogenase (GAPDH) as an internal control geneduring the normalization of the values. The primers for the qRT-PCR are (Forward BDNF AAAGTCTGCATTACATTCCT; Reverse BDNF TCTAAAATGTAAACGGAATG; Forward GAPDH GGAGTAAGAAACCCTGGACCAC, Reverse GAPDH AGGGTGCAGCGAACTTTATTG).
Western blot
This assay was performed following the instructions provided by the kit manufacturers. The cells were subjected to lysis in a lysis buffer (containing protease inhibitors) for 10 min and later centrifuged for 15 min at 13.000 rpm to recover the cell lysates. A Micro BCATM protein assay reagent kit was used to determine the protein concentration of the lysates. Further, a dilution of the lysates was made in LDS sample buffer and sample reducing agent. After dilution, the samples were loaded onto NU-PAGE Bis-Tris gels and run at 120 V, 150 mA. The samples were later transferred to nitrocellulose membrane using a tank transfer unit (Hoefer TE 22) and transfer buffer (NuPAGE) which has been supplemented with methanol (20%). The system was run at 90 v and 125 mA for 2 h at RT. The nitrocellulose membrane was quenched with dried skimmed milk (5%) for 60 min and later incubated at 4 °C overnight in a cooled incubator shaker using rabbit anti-BDNF polyclonal antibody (1 µg/mL, Abcam, UK) as a primary antibody. After incubation, free primary and secondary antibodies were washed off with PBS-Tween before incubating the membranes with 1 µg/mL horseradish peroxidase, which has been conjugated with goat anti-rabbit IgG (sc-2005, Santa Cruz Biotechnology, Santa Cruz) for 60 min at RT. The bounded antibodies were determined using an enhanced chemiluminescence ECL kit and visualized on X-ray films.
ELISA
The levels of BDNF secretion from the transfected NSCs were estimated using an ELISA Kit (CYT306 for BDNF) according to the manufacturers’ instructions. The supernatant of the cultured NSCs was analyzed in an ELISA reader at 450 nm to determine the BDNF concentration.
Statistical analysis
ANOVA with Tukey’s multiple comparisons was used to analyze the data derived from the study in SPSS 10 (SPSS Inc., Chicago, IL). Differences with p < .05 were considered statistically significant.
Results and discussion
Cell culture and NSCs generation
After the third passage, the isolated BMSCs exhibited spindle shapes as characterized using an anti-fibronectin antibody. The percentage of the immune-reactive cells was 91.24 ± 0.821%. The cells were immune-stained with other markers such as CD90 and CD105 as presented in . is a phase contrast image of the neurospheres. The NSCs showed immune-reactivity to anti-nestin, anti-NF68, and anti-NF 200 at the respec-tive percentages of 75.56 ± 2.64%, 74.12 ± 2.65%, and 19.48 ± 2.47%. NF-200, a marker for the differentiated neurons presented significantly lower percentages as shown in .
Figure 1. Immunostaining of the BMSCs of the third passage. A, shows the BMSCs labeled with mouse anti-fibronectin monoclonal primary antibody followed by rabbit anti-mouse secondary antibody conjugated with FITC and counterstained with ethidium bromide. B and C were immunostained with anti-CD90 and CD105, respectively. D is a phase contrast image of a neurosphere (scale bar A = 20, B = 20, C = 5, and D = 200 μm).
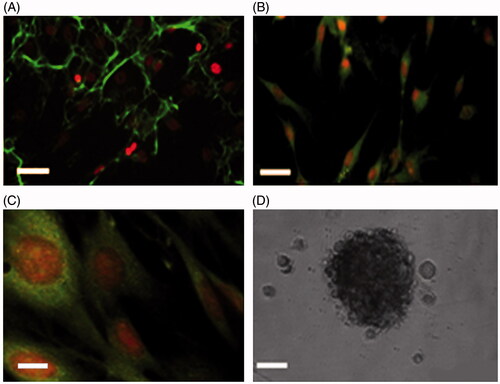
Figure 2. Immunostaining of the NSCs with anti-nestin, NFs (NF 68 and NF-200). A, shows NSCs labeled with mouse anti-nestin monoclonal primary antibody followed by rabbit anti-mouse secondary antibody conjugated with FITC. B and C were immunostained with anti-NF68 and NF200, respectively. D–F, represent the phase contrast images of the same field in A, B, and C, respectively (scale bar = 200 μm).
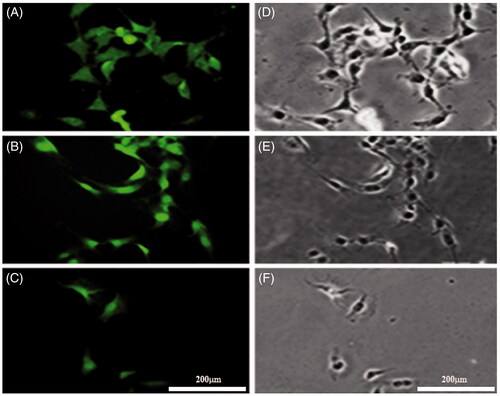
Characterization of SPIONs
The size and morphology of the uncoated SPIONs were characterized by TEM and found to be spherically shaped (), having an average diameter of 10–12 nm, while the PLL-coated SPIONs had an approximately double the size of the uncoated SPIONs (). The zeta potential indicates that the PLL-coated SPIONs had a positive surface charge of ξ + 16.9 mV compared to ξ − 31.3 mV of the uncoated SPIONs (). Additionally, the FTIR spectra () showed that the spectrum of the PLL-coated particles differed from that of the uncoated particles. Moreover, a broad and strong absorption (2086.26 cm−1) was observed in the spectrum of the PLL-coated particles, which could correspond to the ionic interactions between PLL and the SPIONs. This finding suggests the existence of positively charged amine groups at the end of the PLL side chains. These results proved the successful coating of the SPIONs with the PLL. The uptake of the uncoated SPIONs (ξ − 31.3 mV) was compared to that of the PLL-coated SPIONs (ξ + 16.9 mV). The latter can be attracted to the negative charges of the cell membrane, resulting in an increase in their endocytosis [Citation24,Citation25] and a high labeling efficiency of ∼100% ().
Figure 3. Morphological and chemical characterization of coated and uncoated NPs. A, represents transmission electron microscopy images of uncoated supra paramagnetic iron oxide NPs (SPIONs), and SPIONs coated with ploy-l-Lysine (B, SPIONs-PLL, inset; scale bar= 10 μm). C and D, represent the zeta potential of uncoated SPIONs with a sharp peak and negative charge (ξ = –31.3 mV) and coated magnetic NPs (SPIONs-PLL) with positive surface charges (ξ = +16.9 mV). E represents the FTIR spectrum of SPIONs NPs alone and of those coated with the PLL.
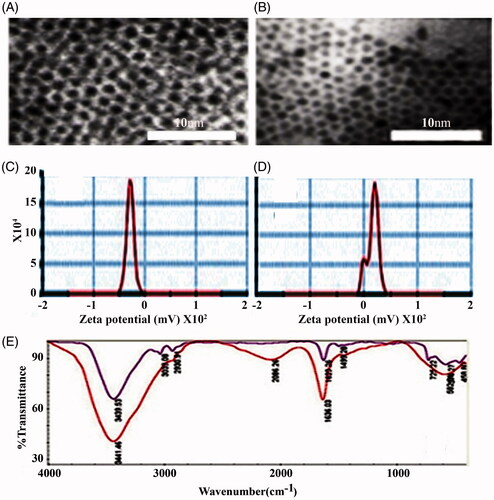
Prussian blue staining
showed the Prussian blue stain of the labeled stem cells. The figure revealed an abundant uptake of the SPIONs in the cytoplasm, as well as a significantly higher number of intracellular NPs compared to the intercellular uptake (, p < .05), as indicated by the histological analysis. The labeling efficiency of the NSCs was ∼100%. Moreover, showed the transfection efficiency of SPIONs-PLL as a non-viral carrier using the EGFP vector. GFP was consistently expressed in the eukaryotic cells with an approximate efficiency of 18%.
Figure 4. Prussian blue staining. A, NSCs labeled with SPIONs and stained with Prussian blue staining (scale bar = 50 μm). Intracytoplasmic blue dots indicate the presence of intracellular SPIONs. B, Immunofluorescent detection of green fluorescence protein expression using SPIONs-PLL as a nonviral transfection method (scale bar = 100 μm). C, Histogram of the mean number of SPIONs particles located at intracellular and intercellular spaces). *is significantly higher than the other experimental groups (p < .05).
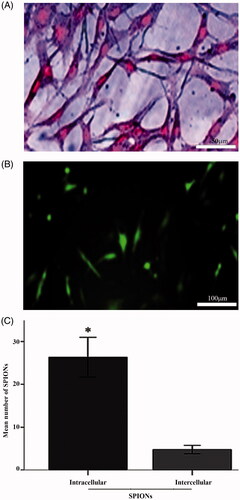
Plasmid constructs
According to data sequencing analysis, the pro-BDNF gene was sub-cloned into the pSecTag2/HygroBafter the murine Ig-Kappa Chain V-J2-C signal peptide without any mutationto construct the pSecTag2/HygroB-human pro-BDNF vector. The inserted pro-BDNF in the expression vector demonstrated the validity of the human pro-BDNF gene sequence as obtained from the UniProt and GenBank bioinformatics databases. The construct (pSecTag2/HygroB-human pro-BDNF vector) was digested with ECORV and XHOI with a separation of the pro-BDNF fragment (690 bp). The transfected cells with the recombinant vector showed the amplification of mRNA by RT-PCR (see ). The results of the Western blot assays of BDNF in the transfected cell lysate and supernatant are shown in , where clear bands of BDNF protein were displayed.
Figure 5. The secretory vector map (pSecTag2/HygroB. A, shows the pSecTag2/HygroB mammalian expression vector construction. Human proBDNF was subcloned into the expression vector after murine Ig-Kappa chain V-J2-C signal peptide in the pSecTag2/HygroB between the EcoRV and XhoI restriction sites to construct pSecTag2/HygroB-human proBDNF vector. B, shows an electrophorogram of a Pro-BDNF gene subcloning into the secretory plasmid pSecTag2/Hygro 2. U: Lane an (undigested: control) and D (digested) show the restriction map of the plasmid digested by ECORV and XHOI (arrowhead: 690 bp, pro-BDNF). (L) represents the DNA Ladder mix. C, shows an electrophorogram of reverse transcriptase polymerase chain reaction (RT-PCR). RT-PCR is a product of BDNF mRNA extracted from transfected rNSCs. L represents the DNA ladder, T: represents a BDNF band of transfected NSCs. N: represents the non-template control (negative control), G: represents GAPDH band (internal control) and C: represents the negative control. D, shows a western blot of BDNF protein in lysates hBDNF transfected rat NSCs (L), and (S) represents the blotting in of the supernatant.
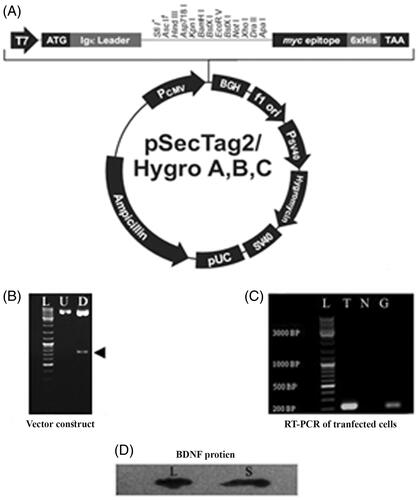
Evaluation of NSC transfection potency
The results showed a time and dose-dependent cytotoxicity of the SPIONs on NSCs (). The SPIONs were less cytotoxic at the concentration of 25 µg/mL as the cell viability was 91% via MTT cell viability assay (). The ELISA results revealed a transfected NSCs supernatant BDNF concentration of 30.326 ± 5.9 pg/mL compared to (5.85 ± 3.11 pg/mL of the non-transfected NSCs (, p < .00). In the last decade, several studies have indicated the concept of magnetic drug targeting in gene-transfer therapies [Citation26] (often referred to as Magnetofection) in both viral and non-viral methods [Citation27]. Our data showed that 18% of the cells were EGFP positive after transfection with the pEGFP-N1 vector. These results agreed with the outcome of a previous study where 11% yield was reported after a transient transfection of rat BMSCs [Citation28]. Some intrinsic and extrinsic pathways such as cell density, ratio of the vector, transfection agent, amount of vector, dilution, vector formation, transfection reagent complexes, expression time course, the presence of serum during transfection, pH, ionic strength, and temperature have been confirmed to affect transfection efficiency. Several viral and non-viral vectors exist for gene transfer in gene therapy. Viral vectors such as retrovirus, adenovirus, and lentivirus have shown a high transduction efficiency [Citation19]. However, this system faces several limitations such as random insertion of DNA, inability to transduce large genes, and a possible host immune reaction. Therefore, their application in-vivo is very limited. Non-viral gene delivery carriers such as cationic polymers have been investigated to overcome some of the problems observed with the viral vectors [Citation29]. DNA fragments can be condensed into nano-sized by cationic polymers via an electrostatic interaction to form a polymer/DNA system for targeted cell nucleus deliveries. Cationic polymers or liposomes can also protect DNA from nuclease attack in cells [Citation30]. Several cationic polymers such as polyethyleneimine [Citation31], polyamidoaminedendrimers [Citation32], and poly(2-dimethylamino)ethyl-methacrylate [Citation33] have been studied as non-viral gene carriers. However, these current systems suffer from inducing a low transfection efficiency or having a high in vitro and in vivo cytotoxicity [Citation34]. Lipofectamine (liposome-mediated gene transfer) is considered as the most referenced transfection reagent and is used as a basis of comparison for the efficiencies of other transfection methods [Citation35]. The negatively charged cell surface facilitates the binding with positively charged PLL, resulting in NPs uptake [Citation36].The conventional calcium phosphate gene-transfer system has been routinely used for both transient and stable transfection in many cell types; however, this system is insufficient and presents poor reproducibility compared to other non-viral carriers [Citation37].
Figure 6. Effect of (SPIONs) in viability of NSCs. A, The percentage of viable cells (NSCs) loaded with supra paramagnetic iron oxide NPs (SPIONs). The concentrations of SPIONs as indicated (10, 25, 50, and 100 µg/mL) at different incubation times (1, 1.5, 3, 6, 12, and 24 hr, respectively). B, the percentage of viable cells loaded with SPIONs coated with ploy-l-Lysine (SPIONs-PLL) at 25, 50, and 100 µg/mL. C, shows a histogram of BDNF expression levels in transfected and non-transfected NSCs. *is significantly higher than the other experimental groups (p < .05).
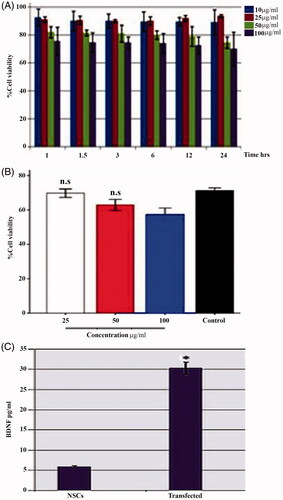
Conclusions
The development of gene-transfer approaches provides new fundamental biological processes of high relevance for molecular medicine. Our data revealed that NSC culture by pSecTag2/Hygro B pro-BDNF vector was efficiently transfected and stabilized by Hygromycin B using SPIONs-PLL as a non-viral nanocarrier. This system provides the cells with a constant supply of BDNF gene that can be used for future in vivo treatmentof neurodegenerative diseases such as Parkinson’s disease, Alzheimer’s disease, Huntington’s disease, and Amyotrophic Lateral Sclerosis. Pro-BDNF gene has been successfully sub-cloned by XHOI and EcoRV of the constructed pSecTag2/Hygro B expression plasmid. Immunocytochemical methods indicated the purity of the isolated stem cells and NSC culture. The magnetic NPs (SPIONs) were prepared using a chemical co-precipitation method, resulting in their synthesis with a diameter of 10–12 nm as confirmed by TEM. Although low concentrations of SPIONs (25 µg/mL) were used, PLL at a concentration of 0.75 μg/mL was used as a polycationic transfection agent for the coating of the SPIONs. The coating was confirmed by zeta potential measurements and FTIR spectroscopy. Our findings demonstrated PLL-coated SPIONs as a promising gene carrier compared to other non-viral gene carriers.
Acknowledgements
The authors are thankful to the Shefa Neurosciences Research Center at Khatam Al-Anbia Hospital, Tehran, Iran. They are also grateful for the support of the Faculty of Biological Science, Tarbiat Modares University, Tehran, Iran for providing necessary facilities.
Disclosure statement
No potential conflict of interest was reported by the authors.
References
- Nagahara AH, Tuszynski MH. Potential therapeutic uses of BDNF in neurological and psychiatric disorders. Nat Rev Drug Discov. 2011;10:209–219.
- Lu B. BDNF and activity-dependent synaptic modulation. Learn Mem. 2003;10:86–98.
- Nagappan G, Zaitsev E, Senatorov VV Jr, et al. Control of extracellular cleavage of ProBDNF by high frequency neuronal activity. Proc Natl Acad Sci USA 2009;106:1267–1272.
- Mowla SJ, Farhadi HF, Pareek S, et al. Biosynthesis and post-translational processing of the precursor to brain-derived neurotrophic factor. J Biol Chem. 2001;276:12660–12666.
- Cohen-Cory S, Kidane AH, Shirkey NJ, et al. Brain-derived neurotrophic factor and the development of structural neuronal connectivity. Dev Neurobiol. 2010;70:271–288.
- Ourednik V, Ourednik J, Park KI, et al. Neural stem cells - a versatile tool for cell replacement and gene therapy in the central nervous system. Clin Genet. 1999;56:267–278.
- Chen B, Gao XQ, Yang CX, et al. Neuroprotective effect of grafting GDNF gene-modified neural stem cells on cerebral ischemia in rats. Brain Res. 2009;1284:1–11.
- Delong R, Stephenson K, Loftus T, Fisher M, et al. Characterization of complexes of oligonucleotides with polyamidoamine starburst dendrimers and effects on intracellular delivery. J Pharm Sci. 1997;86:762–764.
- Rejeeth C, Vivek R. Comparison of two silica based nonviral gene therapy vectors for breast carcinoma: evaluation of the p53 delivery system in Balb/c mice. Artif Cells Nanomed Biotechnol. 2017;45:489–494.
- Nematollahi MH, Torkzadeh-Mahanai M, Pardakhty A, et al. Ternary complex of plasmid DNA with NLS-Mu-Mu protein and cationic niosome for biocompatible and efficient gene delivery: a comparative study with protamine and lipofectamine. Artif Cells Nanomed Biotechnol. 2017;28:1–11.
- Ahmadkhani L, Akbarzadeh A, Abbasian M. Development and characterization dual responsive magnetic nanocomposites for targeted drug delivery systems. Artif Cells Nanomed Biotechnol. 2018;46:1052–1063.
- Zhang Y, Zhang J. Surface modification of monodisperse magnetite nanoparticles for improved intracellular uptake to breast cancer cells. J Colloid Interface Sci. 2005;283:352–357.
- Ramasamy S, Sam David RJR, Enoch IVMV. Folate-molecular encapsulator-tethered biocompatible polymer grafted with magnetic nanoparticles for augmented drug delivery. Artif Cells Nanomed Biotechnol. 2018;4:18.
- Ito A, Kamihira M. Tissue engineering using magnetite nanoparticles. Prog Mol Biol Transl Sci. 2011;104:355–395.
- Babic M, Horák D, Trchová M, et al. Poly(L-lysine)-modified iron oxide nanoparticles for stem cell labeling. Bioconjugate Chem. 2008;19:740–750.
- Wu W, He Q, Jiang C. Magnetic iron oxide nanoparticles: synthesis and surface functionalization strategies. Nanoscale Res Lett. 2008;3:397–415.
- McBain SC, Yiu HH, Dobson J. Magnetic nanoparticles for gene and drug delivery. Int J Nanomed. 2008;3:169–180.
- Wu GY, Wu CH. Receptor-mediated in vitro gene transformation by a soluble DNA carrier system. J Biol Chem 1987;10:4429–4432.
- Midoux P, Monsigny M. Efficient gene transfer by histidylated polylysine/pDNA complexes. Bioconjug Chem. 1999;10:406–411.
- Mok H, Zhang M. Superparamagnetic iron oxide nanoparticle-based delivery systems for biotherapeutics. Expert Opin Drug Deliv. 2013;10:73–87.
- Movaghar B, Tiraihi T, Mesbah-Namin SA. Transdifferentiation of bone marrow stromal cells into Schwann cell phenotype using progesterone as inducer. Brain Res. 2008;1208:17–24.
- Arbab AS, Yocum GT, Wilson LB, et al. Comparison of transfection agents in forming complexes with ferumoxides, cell labeling efficiency, and cellular viability. Mol Imaging 2004;1:24–32.
- Albukhaty S, Naderi-Manesh H, Tiraihi T. In vitro labeling of neural stem cells with poly-L-lysine coated super paramagnetic nanoparticles for green fluorescent protein transfection. Iran Biomed J. 2013;17:71–76.
- Chen L, Mccrate JM, Lee JC, Li H. The role of surface charge on the uptake and biocompatibility of hydroxyapatite nanoparticles with osteoblast cells. Nanotechnology 2011;22(10):105708.
- Frank JA, Anderson SA, Kalsih H, et al. Methods for magnetically labeling stem and other cells for detection by in vivo magnetic resonance imaging. Cytotherapy 2004;6:621–625.
- Amirsaadat S, Pilehvar-Soltanahmadi Y, Zarghami F, et al. Silibinin-loaded magnetic nanoparticles inhibit hTERT gene expression and proliferation of lung cancer cells. Artif Cells Nanomed Biotechnol. 2017;45:1649–1656.
- Scherer F, Anton M, Schillinger U, et al. Magnetofection: enhancing and targeting gene delivery by magnetic force in vitro and in vivo. Gene Ther. 2002;9:102–109.
- Vanderbyl S, MacDonald GN, Sidhu S, et al. Transfer and stable transgene expression of a mammalian artificial chromosome into bone marrow-derived human mesenchymal stem cells. Stem Cells. 2004;22:324–333.
- Nayerossadat N, Maedeh T, Ali PA. Viral and nonviral delivery systems for gene delivery. Adv Biomed Res. 2012;1:27.
- Luo D, Saltzman WM. Synthetic DNA delivery systems. Nat Biotechnol. 2000;18:33–37.
- Bartman CM, Egelston J, Ren X, et al. A simple and efficient method for transfecting mouse embryonic stem cells using polyethylenimine. Exp Cell Res. 2015;330:178–185.
- Wang H, Shi HB, Yin SK. Polyamidoamine dendrimers as gene delivery carriers in the inner ear: how to improve transfection efficiency. Exp Ther Med. 2011;2:777–781.
- Zuidam NJ, Posthuma G, de Vries ET, et al. Effects of physicochemical characteristics of poly(2-(dimethylamino)ethyl methacrylate)-based polyplexes on cellular association and internalization. J Drug Target. 2000;8:51–66.
- Cohen H, Levy RJ, Gao J, et al. Sustained delivery and expression of DNA encapsulated in polymeric nanoparticles. Gene Ther. 2000;7:1896–1905.
- Dalby B, Cates S, Harris A, et al. Advanced transfection with Lipofectamine 2000 reagent: primary neurons, siRNA, and high-throughput applications. Methods. 2004;33:95–103.
- Du J, Sun Y, Shi QS, et al. Biodegradable nanoparticles of mPEG-PLGA-PLL triblock copolymers as novel non-viral vectors for improving siRNA delivery and gene silencing. Int J Mol Sci. 2012;13:516–533.
- Sokolova VV, Radtke I, Heumann R, et al. Effective transfection of cells with multi-shell calcium phosphate-DNA nanoparticles. Biomaterials 2006;27:3147–3153.