Abstract
Given the current conditions of life, one of the problems that the world is facing and is rapidly expanding is diabetes. The existing treatment methods are not responsive to these patients. Today, due to the advent of tissue engineering, cell and stem cell therapies, there are many hopes for treating these patients. In the present study, Polyethersulfone (PES) nanofibers were fabricated by electrospinning and then coated by collagen (PES-collagen), since this protein is abundant at the pancreatic extracellular matrix. After scaffold characterization, pancreatic differentiation potential of human induced pluripotent stem cells (hiPSCs) was investigated when cultured on PES-collagen by RT-qPCR, Immunofluorescence staining and insulin and C-peptide release assays. Pancreatic genes and proteins in cultured iPSCs on PES-collagen were expressed significantly higher than those cultured on culture plate as 2 D control group. Although differentiated cells in both groups are functional and secrete C-peptide and insulin in response to glucose challenges according to the immunoassay result. Considered together, PES-collagen demonstrated that it can be effective during pancreatic differentiation of the stem cells and can also be considered as a promising candidate for use in pancreatic tissue engineering application.
Introduction
The production of engineered tissue is the basis of a new therapeutic approach for patients whose tissue function is lost in one organ. These tissues can also be used in in vitro studies to evaluate the natural growth of the tissue or pathologic function [Citation1]. One of the methods of constructing a new tissue is to culture high-density cells uniformly on three-dimensional (3D) polymer structures (scaffolds) and transferring into the proper culture medium to produce a normal and functional tissue [Citation2]. The scaffolds provide defined and specific structures for the cells to connect and form the tissue. Since one of the most important diseases during last decades is type I diabetes mellitus (T1DM) which many patients through the world suffer from this and the current treatment does not cure many of the complications associated with the disease [Citation3,Citation4], many researchers have been tried to obtain new opportunities from tissue engineering and regenerative medicine to solve these problems [Citation5–7]. Among many methods used to construct 3 D scaffolds, similar to the extracellular matrix (ECM), electrospinning is an economic technique for the preparation of very thin polymer fibers, which can be easily applied in a lab or on an industrial scale [Citation8]. Among many existing polymeric material sources that can be used to build cellular scaffolds, synthetic polymers are more appropriate choice than natural, since their structure and mechanical strength can be manipulated in the direction of the target tissues [Citation8–10]. Polyethersulfone (PES) was used in this study as a synthetic polymer that used previously for blood purification and to generate other tissues during last decade [Citation11–14]. Although fabricated electrospun of this polymer is hydrophobic and need to increase their wettability according to the increase in their biological functions, there are several choices such as composite with hydrophilic polymers, plasma surface treatment and coating/grafting with functional biological macromolecules which already exists in the natural tissues of the body [Citation15]. We chose collagen for grafting at the surface of the PES electrospun nanofibers as the major protein in the ECM of many tissues in the body [Citation16,Citation17]. One of the other most important part of the tissue engineered construct is cells/stem cells, that could be chosen according to the patient’s condition and their problem. Although many studies were performed by adult stem cells during last decades, it is clear that adult stem cells have many challenges such as shortage of the tissue sources for stem cell isolation, infection, limited differentiation potential, invasive tissue harvest process and ethical issue and concern [Citation18]. For this reason,induced pluripotent stem cells(iPSCs) were generated by Yamanaka et al., through up-regulation of four transcription factor( Oct-4, Sox-2, Klf-4 and Myc-C ) more than a decade ago [Citation19]. During the last decade, studies have been reported that iPSCs promising potentials in solving problems accompained with another type of stem cells in regenerative medicine [Citation20]. One of the available methods with the proper capabilities and perspective of treating diabetic patients is the production of the cells that can produce and secrete insulin, which can be transplanted to the diabetic patients [Citation21,Citation22]. Several studies have been reported that iPSCs have a great insulin-producing cells (IPCs) differentiation potential either by gene transfer or after culturing under different differential mediums in the conventional culture plates including two-dimensional (2D) and three-dimensional (3D) phase. [Citation13,Citation23–25]. In this present study, we improved the biological activity of Polyethersulfone (PES) nanofibers by coating them with collagen and after its characterization, investigated the capacity of PES-collagen to generate IPCs, while iPSCs culture on them in the gene and protein levels.
Material and methods
Stem cell expansion
Human iPSCs that previously produced and characterized in Stem Cells Technology Research Center were cultured and expanded according to the protocol reported in our previous study [Citation13].
Nanofibrous scaffold preparation
Electrospun PES nanofibrous scaffold was according to a protocol modified with Shabani et al [Citation26,Citation27] and after plasma treatment [Citation28], more surface modification was performed by a coating of the collagen on the surface of the fabricated PES nanofibers. Collagen solution (0.1 ng/ml, Sigma Aldrich, St. Louis, MO) was added to the nanofibers and incubated at 4 °C overnight and later washed two times by PBS.
Scanning electron microscopy
For SEM imaging, nanofibers which contains stem cells were fixed by glutaraldehyde (2.5%, Merck, Darmstadt, Germany) for 2 h at room temperature and then dehydrated in a graded ethanol series and then mounted on aluminum and covered with palladium-gold in a sputter-coater (Hitachi, Tokyo, Japan). The specimens were observed under SEM (Hitachi S-4500, Japan).
Insulin-producing cells differentiation
HiPSCs were differentiated to the IPC by applying of multi-step differentiation medium that previously reported in our studies [Citation13,Citation24]: In brief, hiPS EBs were treated with serum-free high glucose DMEM supplemented with 0.5 mmol/L β-ME and 50 ng/ml activin A (Sigma Aldrich) for one week and continued for another week with the medium containing 1% NEAA (Invitrogen, Carlsbad, CA), 20 ng/ml EGF (Sigma Aldrich), 20 ng/ml bFGF, 2 mmol/L L-glutamine, 2% B27 (Invitrogen, Carlsbad, CA), and 10 ng/ml exendin-4 (Sigma Aldrich). In the last week, cells were treated with DMEM supplemented with 10 ng/ml betacellulin (Sigma Aldrich), 2% B27, 10 mmol/L nicotinamide (Sigma Aldrich), and 10 ng/ml exendin-4.
Biocompatibility assay
Fabricated scaffold’s biocompatibility was investigated by viability measurement of the hiPSCs while cultured on them using MTT assay at days 1, 3, 5 and 7 according to the previously reported protocol [Citation13].
Real-time RT-PCR
Total cell RNA was isolated from all groups with the RNeasy Mini Kit (Qiagen, Valencia, CA), then, cDNA was synthesized using the random hexamer primer and M-MuLV Reverse Transcriptase kit (Fermentas, Helsingborg, Sweden) according to the manufactured protocol. SYBR premix ExTaq (Takara Bio, Shiga, Japan) was applied to real-time RT-PCR using an ABI StepOne system with primers displayed in while β2M was considered as an internal control gene.
Table 1. Primer sequences used for real time-PCR in this study.
Immunofluorescence staining
All groups were fixed with 4% cold paraformaldehyde (Sigma Aldrich) for 45 min and then permeabilized with 0.2% Triton X-100 (Sigma Aldrich) and then stained through immunocytochemistry (ICC) assay. ICC was followed by blocking and incubating with anti-Pdx1(1:2000; #ab47267), anti-Insulin (1:200; #ab181547) and 0.1 μg/ml DAPI (Sigma Aldrich) was carried out according to our previously reported protocol [Citation13]. ImageJ software (Version 1.45 s, NIH, USA) was applied for the quantification of immunocytochemistry images.
Glucose-stimulated islet insulin or C-peptide secretion
Cells in all groups were incubated in glucose-free Krebs–Ringer bicarbonate (KRB) buffer containing 5 mM KCl, 25 mM NaHCO3, 2.5 mM CaCl2, 120 mM NaCl, 1.1 mM MgCl2 and 0.1% BSA (Sigma Aldrich) for 2 h and then incubated with various concentrations of glucose. After that medium was collected and insulin and C-peptide secretion was measured using the Ultrasensitive ELISA kit (Mecodia, Uppsala, Sweden).
Statistics
Experiments were carried out in triplicates, REST2009 was applied to real-time RT-PCR data analysis and data were reported as a mean ± standard deviation (SD). the one-way analysis of variance (ANOVA) using SPSS software (SPSS version 17, Chicago, IL) was used for evaluation of significance whereas 0.05 was considered as p values for statistical significance.
Results
Morphological study
The inverted phase contrast and scanning electron microscopes were applied for imaging of the hiPSCs while differentiating into IPCs at the end of the study which is depicted in and respectively. As showed in , images depicted using SEM indicated that scaffolds have a porous surface morphology and interconnected pore structures inside the scaffolds. Human iPSCs cultured on culture plates are shown in , and when cultured on PES-collagen under differentiation medium are shown in and .
Figure 1. Morphological changes of cultured cells in TCPS group. (a) hiPSCs in DMEM (control group). (b) hiPSCs in IPCs differentiation media at day-21. Scale bars are 100 µm.
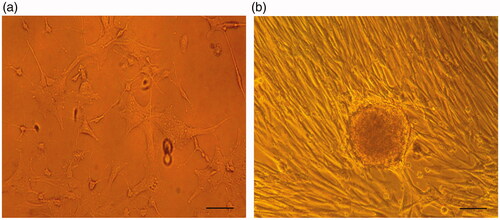
Figure 2. Morphological changes of cultured cells on the 3D nanofibrous scaffold. (a) Unseeded scaffold with interconnected pores. (b) hiPSCs-seeded on the nanofibrous scaffold (control group). The cells show spindle-shape morphology. (c) the hiPSCs-seeded scaffold in IPCs differentiation media at day-21. The cells are round in shape and form spherical dense clusters. Scale bars are a, and b: 30 µm, c: 50 µm.
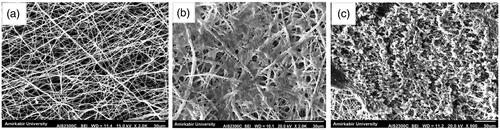
Cell viability
For quantitative biocompatibility evaluation of the fabricated scaffold, hiPSCs were cultured on them and their viability was investigated using MTT assay (. Viability and proliferation rate of hiPSCs was significantly increased when cultured on PES-collagen scaffold in comparison control group at days 3, 5 and 7.
Gene expression
Pdx1, Ngn3, Insulin, Glucagon and Glut2 relative mRNA expression levels were depicted in . Undifferentiated hiPSCs as the control group was expressed in a small amount of these genes, Although these expressions were up-regulated in hiPSCs when cultured under differentiated medium. The most important point in the results obtained from gene expression assay was that the hiPSCs that were cultured on PES-collagen scaffolds showed the highest expression of these genes in comparison with other groups.
Figure 4. Relative expression of pancreatic-specific genes in end-stage derived-IPCs. Gene transcripts of the 3D group are compared to the 2D group. Relative gene expressions were normalized to the human β2M as a reference gene. The value is shown in each graph as mean ± SD. *p < .05, **p < .01, ***p < .001 and ****p < .0001.
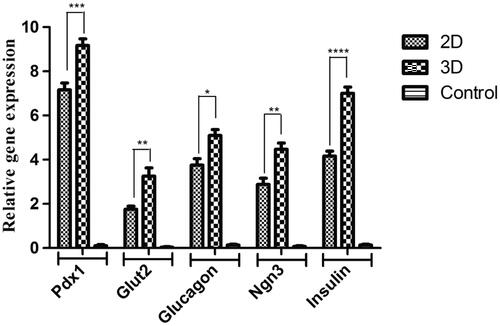
Immunocytochemistry
In agreement with gene expression results, the expression level of pdx1 protein in the nuclei of the hiPSCs cultured under differentiation medium on TCPS was low as well as expression level of insulin protein in cytoplasm as shown in . When these protein expressions evaluated in those cells cultured on PES-collagen scaffolds under differentiation medium, these were significantly sharp. Any expression point of pdx1 and insulin protein was not detected in control group. ICC results were obtained on day 21 and finally as shown in , ICC images were quantified by ImageJ software.
Figure 5. Immunocytochemical analysis of differentiated cells in end-stage derived-IPCs. Nuclei localization of Pdx1 and cytoplasmic localization of insulin in differentiated IPCs at day-14 in the 3D group (Figure 5(a)) and 2D group (Figure 5(b)) were detected by immunofluorescence analysis. Counter-staining of the nucleus (blue) was performed by DAPI and images were obtained by a fluorescence microscope. Bar graph quantification of the immunocytochemistry assay. Each bar represents the relative value of insulin and Pdx1 markers, differences were observed between 3D and 2D groups. Scale bars are 100 µm.
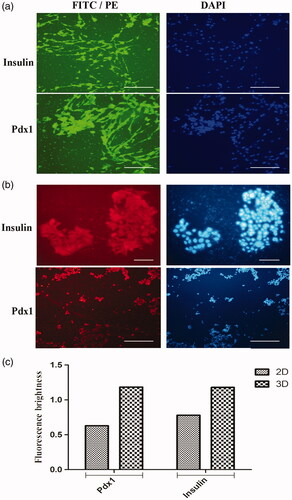
Insulin and C-peptide release
For more detailed evaluation of the hiPSCs differentiation when cultured on TCPS and PES-collagen scaffolds, insulin and C-peptide secretion was measured in response to glucose concentrations. As shown in , these secretions were not significant in control group and secretion differences between TCPS and scaffold groups were also not significant in response to 5.5 mM glucose. Although it was significant in response to 15 and 25 mM glucose.
Figure 6. In vitro release of insulin and C-peptide in IPCs derived at day-21 of differentiation. (a and b) insulin secretion and C-peptide release changes in two groups of IPCs and control group in response to various concentrations of glucose from 5.5 to 25 mM. Values are expressed as mean ± SEM. (n = 6). *p < .05 and **p < .01.
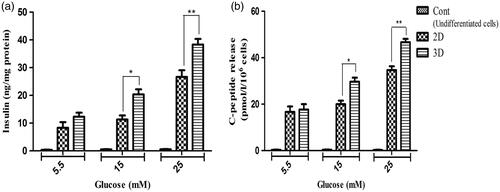
Discussion
One of the most important characteristics of the iPSCs is its patients specificity that can solve all ethical and rejection concerns acompained with transplantation as well as its pluripotency differentiation potential into most type of the cells in the body. Another unique property of the iPSCs is the possibility of the gene editing during iPSC preparation process to overcome any cells deficiency in the patient [Citation29]. Several studies demonstrated that iPSCs have a great potential to differentiate into IPCs when cultured on several 2 D substrate and under differentiation medium [Citation23,Citation30]. But since IPCs are functional cells and need to produce and secrete insulin, simulation of the natural extracellular matrix (ECM) play a very important role in the differentiation and function of these cells [Citation31]. For this reason, many researchers also have been tried to introduce proper 3 D substrate which can properly simulate natural condition of the pancreatic cells [Citation16,Citation32,Citation33]. In the present study, we tried to investigate IPCs differentiation potential of the iPSCs while cultured on the surface of the nanofibrous PES scaffold after coating with collagen, since higher protein present in the islets ECM is collagen to further simulate the pancreatic ECM in vitro [Citation16]. Those stem cells cultured on culture plate dishes (TCPS) as a conventional 2 D substrate were considered as a control group. To indicate biocompatibility of the constructed nanofibers, MTT assay was performed and results showed that the proliferation rate of the iPSCs was increased when cultured on the surface of the PES-collagen in comparison with TCPS as control group, this could be the result of a 3 D structure with higher area in space and also the presence of collagen in the fabricated nanofibrous scaffold. Inverted and electron microscope images demonstrated that differentiated iPSCs on TCPS and PES-collagen substrate respectively showed spherical-round clusters formation (similar to islets observed within pancreatic tissues). After that, to detailed evaluation of the differentiation, gene expression were applied for pancreatic and duodenal homeobox 1 (Pdx1) a key regulator of the identity and function of the beta cell, glucose transporter 2 (Glut2) a transmembrane carrier protein, glucagon secreted by pancreatic islets, neurogenin-3 (Ngn3) that is vital to develop endocrine cells of the islets and insulin. Pdx1 and insulin were also chosen for more investigation at the protein level by using ICC staining. In addition, insulin and C-peptide secretion as a critical functional assay of the IPCs were measured in the IPCs differentiated iPSCs. Our results were demonstrated higher expression level of these markers in iPSCs when cultured on PES-collagen nanofibers in comparison with those cultured on TCPS during the study period. Regarding the results acquired in the previous study [Citation13] and the present study, coating of the collagen on the surface of the PES scaffold resulted in the improved IPCs differentiation efficacy of the iPSCs and it can be introduced as a more appropriate scaffold in comparison with intact PES nanofibers for use in pancreatic tissue engineering applications. Taken together, our results showed that the coating of nanofibers with proteins which are already present in the body can improve biocompatibility of the scaffolds and can promote differentiation process of the stem cells via mimicking the natural microenvironment of the natural tissues.
Conclusion
Improved capacity of PES nanofibers for IPCs production from iPSCs was exhibited at the present study by a coating of PES with collagen as an abundant protein in pancreatic ECM. According to the results, derived IPCs on PES-collagen nanofibers displayed a significant higher expression of pancreatic-specific markers at the gene and protein levels in comparison with TCPS as control group. In addition, IPCs produced in this study were demonstrated as an acceptable response to the glucose challenges as a functional analysis. Overall, PES-collagen is shown as a great potential to be used for pancreatic tissue engineering and regenerative medicine applications in combination with human iPSCs and can consider as a promising candidate for the treatment of patients suffered from diabetic disorders, however, more research can help to accept this suggestion particularly in in vivo investigations.
Acknowledgments
We wish to thank Stem Cell Technology Research Center for the use of their laboratory facilities.
Disclosure statement
No potential conflict of interest was reported by the authors.
References
- Ravi M, Paramesh V, Kaviya S, et al. 3D cell culture systems: advantages and applications. J Cell Physiol. 2015;230:16–26.
- Page H, Flood P, Reynaud EG. Three-dimensional tissue cultures: current trends and beyond. Cell Tissue Res. 2013;352:123–131.
- Saberzadeh-Ardestani B, Karamzadeh R, Basiri M, et al. Type 1 diabetes mellitus: cellular and molecular pathophysiology at a glance. Cell Journal. 2018;20:294–301.
- Association AD. 2. Classification and diagnosis of diabetes. Dia Care. 2016;39:S13–S22.
- Goh S-K, Bertera S, Olsen P, et al. Perfusion-decellularized pancreas as a natural 3D scaffold for pancreatic tissue and whole organ engineering. Biomaterials. 2013;34:6760–6772.
- Niknamasl A, Ostad SN, Soleimani M, et al. A new approach for pancreatic tissue engineering: human endometrial stem cells encapsulated in fibrin gel can differentiate to pancreatic islet beta‐cell. Cell Biol Int. 2014;38:1174–1182.
- Javad H, Parvin P, Masoud S, et al. Application of a novel bioreactor for in vivo engineering of pancreas tissue. J Cell Physiol. 2017;5:3805–3816
- Zhang Y, Lim CT, Ramakrishna S, et al. Recent development of polymer nanofibers for biomedical and biotechnological applications. J Mater Sci: Mater Med. 2005;16:933–946.
- Green JJ, Elisseeff JH. Mimicking biological functionality with polymers for biomedical applications. Nature. 2016;540:386.
- Pereira LX, Viana CTR, Orellano LAA, et al. Synthetic matrix of polyether-polyurethane as a biological platform for pancreatic regeneration. Life Sciences. 2017;176:67–74.
- Falkenhagen D, Strobl W, Vogt G, et al. Fractionated plasma separation and adsorption system: a novel system for blood purification to remove albumin bound substances. Artif Organs. 1999;23:81–86.
- Ma Z, Kotaki M, Inai R, et al. Potential of nanofiber matrix as tissue-engineering scaffolds. Tissue Eng. 2005;11:101–109.
- Mansour RN, Barati G, Soleimani M, et al. Generation of high-yield insulin producing cells from human-induced pluripotent stem cells on polyethersulfone nanofibrous scaffold. Artif Cells Nanomed Biotechnol. 2018;12:1–7.
- Mahboudi H, Soleimani M, Enderami SE, et al. The effect of nanofibre-based polyethersulfone (PES) scaffold on the chondrogenesis of human induced pluripotent stem cells. Artif Cells Nanomed Biotechnol. 2017;6:1–9.
- Meng X, Leslie P, Zhang Y, et al. Stem cells in a three-dimensional scaffold environment. Springerplus. 2014;3:80.
- Aloysious N, Nair PD. Enhanced survival and function of islet-like clusters differentiated from adipose stem cells on a three-dimensional natural polymeric scaffold: an in vitro study. Tissue Eng Part A. 2014;20:1508–1522.
- Parenteau-Bareil R, Gauvin R, Berthod F. Collagen-based biomaterials for tissue engineering applications. Materials. 2010;3:1863–1887.
- Caplan AI. Adult mesenchymal stem cells for tissue engineering versus regenerative medicine. J Cell Physiol. 2007;213:341–347.
- Takahashi K, Yamanaka S. Induction of pluripotent stem cells from mouse embryonic and adult fibroblast cultures by defined factors. Cell. 2006;126:663–676.
- Schroeder IS. Potential of pluripotent stem cells for diabetes therapy. Curr Diab Rep. 2012;12:490–498.
- Sneddon JB, Tang Q, Stock P, et al. Stem cell therapies for treating diabetes: progress and remaining challenges. Cell Stem Cell. 2018;22:810–823.
- Abdulazeez SS. Diabetes treatment: a rapid review of the current and future scope of stem cell research. Saudi Pharm J. 2015;23:333–340.
- Enderami SE, Mortazavi Y, Soleimani M, et al. Generation of insulin‐producing cells from human‐induced pluripotent stem cells using a stepwise differentiation protocol optimized with platelet‐rich plasma. J Cell Physiol. 2017;10:2878–2886
- Abazari MF, Soleimanifar F, Aleagha MN, et al. PCL/PVA nanofibrous scaffold improve insulin-producing cells generation from human induced pluripotent stem cells. Gene. 2018. doi: 10.1016/j.gene.2018.05.115.
- Lahmy R, Soleimani M, Sanati MH, et al. miRNA-375 promotes beta pancreatic differentiation in human induced pluripotent stem (hiPS) cells. Mol Biol Rep. 2014;41:2055–2066.
- Shabani I, Haddadi-Asl V, Seyedjafari E, et al. Improved infiltration of stem cells on electrospun nanofibers. Biochem Biophys Res Commun. 2009;382:129–133.
- Mahboudi H, Soleimani M, Enderami S, et al. The effect of nanofibre-based polyethersulfone (PES) scaffold on the chondrogenesis of human induced pluripotent stem cells. Artif Cells Nanomed Biotechnol. 2017;6:1–9.
- Mahmoodinia Maymand M, Soleimanpour-lichaei HR, Ardeshirylajimi A, et al. Improvement of hepatogenic differentiation of iPS cells on an aligned polyethersulfone compared to random nanofibers. Artif Cells Nanomed Biotechnol. 2017;4:1–8.
- Kondo Y, Toyoda T, Inagaki N, et al. iPSC technology‐based regenerative therapy for diabetes. J Diabetes Investig. 2018;9:234–243.
- Zhang D, Jiang W, Liu M, et al. Highly efficient differentiation of human ES cells and iPS cells into mature pancreatic insulin-producing cells. Cell Res. 2009;19:429–438.
- Benton G, George J, Kleinman H, et al. Advancing science and technology via 3D culture on basement membrane matrix. J Cell Physiol. 2009;221:18–25.
- Enderami SE, Kehtari M, Abazari MF, et al. Generation of insulin-producing cells from human induced pluripotent stem cells on PLLA/PVA nanofiber scaffold. Artif Cells Nanomed Biotechnol. 2018;27:1–8.
- Enderami SE, Soleimani M, Mortazavi Y, et al. Generation of insulin‐producing cells from human adipose‐derived mesenchymal stem cells on PVA scaffold by optimized differentiation protocol. J Cell Physiol. 2017;5:4327–4337.