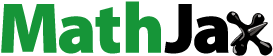
Abstract
Food-originated angiotensin-I-converting enzyme (ACE)-inhibitory peptides to preserve hypertension are widely investigated over the past decade. Our research aims to discovery novel ACE-inhibitory peptides from bovine milk by couple of complex proteases (alcalase and protease). By means of response surface methodology with the conditions of pH 9.01, 61.81 °C and 6.5% ratio of enzyme to substrate, the hydrolysis model contributes to best-performing ACE-inhibitory activity of 85.02%. Through the further purification by consequent ultrafiltration, macroporous resin and gel chromatography, fraction G2-2 is eventually obtained with ACE-inhibitory activity as high as 92.7%. Two novel peptides of VLPVPQ and VAPFPE are identified by Q-Exactive LC–MS/MS. The molecular docking study further suggests that two novel peptides have good combinations of the S1 and S2 active site pockets and Zn(II) of ACE. Our study provides a fitted mathematical model to produce two novel milk-derived ACE-inhibitory peptides, potentially developing the functional foods, especially for hypertension therapy as initial treatment.
Introduction
Hypertension is identified as the leading cause of cardiovascular mortality. It has become a global health challenge due to its high prevalence and severe consequences [Citation1]. Growing rate of adults suffered from hypertension pushes forward the development of pharmacological therapy for hypertension [Citation2]. The direct and indirect costs of cardiovascular therapy were estimated as $316.1 billion from 2012 to 2013 in USA [Citation3]. Considering the increasing costs of hypertension therapy, exploration of bioactive peptide from food to regulate blood pressure would be greatly beneficial since food resource is abundant and natural with less side-effect.
Angiotensin-I-converting enzyme (ACE) (peptidyl-dipeptidase A, EC 3.4.15.1) with broad in vitro substrate specificity produces a highly powerful vasoconstrictor and deactivates the bradykinin catalytic activity [Citation4]. Moreover, the medicines containing ACE-inhibitors are closely associated with various symptoms such as cough, angioedema, hypotension and hyperkalemia. Encouragingly, the recent research demonstrates that dietary-derived bioactive peptides exhibit the identical ACE-inhibitory function during gastrointestinal tract, and indirectly regulate blood pressure [Citation5]. Undoubtedly, this pattern of food-intake is considered as a security approach in comparison with medicinal products, and dramatically alleviates pharmaceutic preparations-induced side-effects. ACE-inhibitory peptides are small protein fragments with specific amino acid sequences, normally contain amino acids in the size range of 2–9 [Citation6]. These special peptides exhibit no effect in their parent protein sequence but display active when they are digested. Therefore, they are potentially suitable for the development of functional food and health products.
Hitherto, various ACE-inhibitory peptides are successively extracted from marine animals or mammal milk by enzymatic digestion or fermentation [Citation7]. The fermented dairy products with typical peptides of Val-Pro-Pro and Ile-Pro-Pro are proved to contribute to antihypertensive in patients. Enzymatic hydrolysis is especially favoured in ACE-inhibitory peptides production because of specific digestion location. The enzymes with high frequency in hydrolysis are alcalase, pepsin, neutral protease and trypsin. Zhang et al. [Citation8] reported that the combined and sequential use of alcalase followed by trypsin provided high degree of protein hydrolysis which was much superior to either of them. The peptides derived from goat milk protein showed high ACE-inhibitory activity when milk protein was hydrolysed by the combination of subtilisin and trypsin in comparison to the individual enzyme [Citation9]. This suggests that complex protease hydrolysis was efficient to produce milk-derived ACE-inhibitory peptides with high activity. However, protease from Bacillus licheniformis is rarely investigated in bovine milk hydrolysis and the related ACE-inhibitory peptides are barely reported. Therefore, our study tries to hydrolyse bovine milk by a new combination of alcalase and protease from Bacillus licheniformis, aiming to release novel ACE-inhibitory peptides.
Bovine dairy products, an important source of human nutrition, have penetrated into all aspects of life. In decades, voluminous researches are dedicated to exploring ACE-inhibitory peptides from bovine milk protein, which have antithrombotic effects and alter the immune response [Citation10,Citation11]. Most milk-originated ACE-inhibitory peptides are characterized with a short chain and relatively low molecular mass for better combination of ACE. Previous researchers found that the binding of peptide and ACE is attributed to the function of the C-terminal tripeptide sequence that containing proline at the end interacts with hydrophobic amino acid of ACE-inhibitory peptides. The relationship between ACE and bioactive peptides may tailor the protein biological expression [Citation12]. The molecular docking model could prejudge the interaction between the maternal protein and the introduced molecules, thereby revealing the relationship between proteins.
In this study, the novel ACE-inhibitory peptides are discovered from bovine milk hydrolysate by a new couple of complex proteases. The hydrolysis conditions are optimized by response surface methodology (RSM). In addition, a molecular docking model is established to uncover the interaction mechanism of the ACE and novel peptides at the molecular level.
Materials and methods
Materials
Skimmed bovine milk powder (Fonterra & Anchor, Auckland, New Zealand) and hippuryl-histidyl-leucine (HHL) and ACE (Sigma Chemical Co. Ltd., St. Louis, MO) were prepared as the experimental precursors. The commercial proteases used in this study were alcalase (2.4 U/g) (Novozymes, Bagsvaerd, Denmark) and protease from Bacillus licheniformis (2.4 U/g) (Sigma-Aldrich, St. Louis, MO).
Enzymatic hydrolysis
Hydrolysis of bovine casein was operated as the traditional Adamson and Reynolds approach [Citation13]. Skimmed bovine milk was diluted by distilled water with the 5% (w/v) ratio, and the proteases were adopted to hydrolyse the proteins under the 6.5% enzyme to substrate (E/S) ratio. The pH value in the hydrolysis mixture was adjusted by adding 0.1 mol/L NaOH (Metrohm Ltd., Herisau, Switzerland). The hydrolysis reaction continued 6 h and was ended by heating at 90 °C for 15 min. The pH 3.5 in the reaction mixture was controlled by adding 1.0 mol/L HCl. After centrifugation, the supernatant was modified to pH value of 8.3 to determine the ACE-inhibitory activity.
Determination of degree of hydrolysis
According to the classical Lowry method [Citation14], the degree of hydrolysis (DH) was clearly determined. Bovine serum albumin was used as a control sample in the experiment. The DH was measured according to the following formula.
(1)
(1)
where B, Mb, α, Mp and htot are the amount of NaOH (mL), concentration of 0.1 mol/L NaOH, degree of dissociation of α-amino (α = 0.442), quality of protein, and protein of the total number of peptides bonds (bovine milk: htot = 8.29 mmol/g).
Determination of ACE-inhibitory activity
According to the traditional Cushman and Cheung approach [Citation15], the ACE-inhibitory activity can be well determined. The 100 μL solution was incubated with 200 μL substrate (5 mM HHL dissolved into 0.1 M Na2B4O7 buffer solution with 0.3 M NaCl at pH 8.3). After that, the 20 μL ACE solution (100 mU/mL) was introduced into the reaction vessel. After incubation at 37 °C in half an hour, the production processes were terminated by dripping 1 M HCl of 0.25 mL. Ethyl acetate of 1.7 mL was then added into solution. Finally, the ethyl acetate layer of 1 mL was collected and evaporated at 120 °C in half an hour. After diluted into distilled water, the absorption spectrum with wavelength of 228 nm was measured by a spectrophotometer UV5300-PC (Yuanxi, Shanghai, China).
The ACE-inhibitory activity rate can be evaluated by the following formula.
(2)
(2)
where A, B and C are the absorbance without the enzymatic peptide sample, with both ACE and enzymatic peptide sample, and without ACE, respectively. These hydrolysate and peptide activities commonly stand for inhibition proportion in the certain protein density or the density at the half of the original activity (IC50).
Box–Behnken’s layout
A Box–Behnken (B–B) layout among pH (X1), temperature (X2) and E/S (X3) was arranged as pH (8.8, 9.0 and 9.2), temperature (56, 60 and 64 °C) and E/S (6.0%, 6.5% and 7%) (). The experimental results were expressed as the following quadratic model:
(3)
(3)
ACE-inhibitory peptides refinement
Bovine casein hydrolysate was separated and fractionated (U1 < 10 kDa, U2 < 5 kDa and U3 < 1 kDa) using VivaSpin molecular weight cut-off (MWCO) membranes. The desalination processes of U3 with the highest ACE-inhibitory activity were performed with macroporous resin DA201-C under the conditions of pH 4.0 and 75% ethanol as eluent. Then, the 150 mg/mL G3 fraction was embedded into the 1.0 cm × 10 cm column (Sephadex G-25) that was eluted by 0.8 mL/min distilled water and detected at 220 nm. The part holding highest ACE-inhibitory was then shifted into a gel filtration column (Sephadex G-15) with 0.8 mL/min distilled water elution.
Amino acid composition analysis by L-8900
To analysis the amino acid composition, the depurated ACE-inhibitory peptides were hydrolysed by 6.0 M HCl of 10 mL, then microwaved for 2 min and flushed with nitrogen at 110 °C for 23 h. After nature cooling, the solutions were filtered by the 0.45 μm needle filtration. The qualitative and quantitative analyses of amino acids were operated by the amino acid analyser (Hitachi L-8900, Tokyo, Japan).
Amino acid sequence identification
The amino acid sequences of the obtained peptides were identified using Q-Exactive LC–MS/MS (Thermo Finnigan, San Jose, CA).
Molecular docking on peptides and ACE
The structure of the obtained peptides was modelled by Chem Draw Ultra 8.0.3 software (CambridgeSoft Corp., Cambridge, MA). In addition, the related ACE structure was adopted on the basis of the Protein Data Bank. In this study, ACE was modified by adding polar hydrogens and removing water molecules and inhibitor lisinopril. Docking study was supported by AutoDock Tools 4.2 software (Scripps Research Institute, San Diego, CA). The 2D structure of peptide binding with ACE was predicted by Moe2008.10 software.
Statistical analysis
The numerical analysis studies were evaluated by the statistical analysis system SAS 9.1 (Cary, NC). All the results were expressed in the format of the means ± SD. Each experiment was re-evaluated three times.
Results and discussion
Modelling the hydrolysis condition by RSM
The application of enzymatic hydrolysis in the production of bioactivity peptides is favoured because of its easy controlling, less protein destruction and specific location hydrolysis. Production of ACE-inhibitory peptide by enzymatic hydrolysis is successfully involved in milk-derived or marine animal-derived peptides process. Alcalase is chosen for the generation of inhibitory peptides from sardine muscle, alaska pollock and jellyfish collagen. As1398 neutral protease contributes greatly in bovine casein hydrolysis for the generation of ACE-inhibitory peptides [Citation16]. Pepsin is considered as a great enzyme to separate ACE-inhibitory peptides in goat casein [Citation17].
In this study, we designed a new couple of alcalase and protease from Bacillus licheniformis with the ratio of 1:1. A three-level-three-factor B–B design was carried out to evaluate the hydrolysis condition and the experimental data are shown in . A list of three factors, pH (X1), temperature (X2) and E/S (X3) were evaluated with regards to ACE-inhibitory activity (Y1) and DH (Y2). The activity range varied greatly from 43.28% to 86.72%. A run of experiments with highest activity was not in consistent with high DH. These outputs suggested that the increase in DH barely had a link to the growth of ACE-inhibitory activity, indicating that the hydrolysis of protease contributed to the production of small peptides but not definitely to bioactivity function. Mullally et al. [Citation18,Citation19] also expressed that the DH has no correlation with the ACE-inhibition activity.
Table 1. The B–B layout and response of the interdependent variables under various hydrolysis conditions.
To better understand the hydrolysis condition, the activity was chosen to the response (Y) for the following optimization. The ANOVA of the response was studied for screening the significance of variables and their interactions effects (). All the terms were statistically evaluated by p values. As listed in , all three variables of pH, temperature, and E/S exhibited high significant effects (p < .01), demonstrating that variables were not proportional to the response Y. The quadratic term of another variable of temperature was not significant (p>.05). However, the related item of E/S×temperature was prominent (p < .05). The low F value of pH×E/S demonstrated that there was a weak mutual interaction between them. The response surface model equation was developed. The F value of 23.65 stated that the mathematics model played a guiding role to predict ACE inhibition (%) (p < .01). The p value of .0014 further confirmed this model was indeed the most momentous. The suitability and validity of the quadratic model were located with determinant coefficient (R2). The values of R2 and adjusted R2 of the polynomial model were 97.70% and 93.57%, respectively, which indicated that the model was appropriate. The calculated value of R2 indicated that more than 97.70% of the data were understood as follows:
(4)
(4)
Table 2. The response discrepancy of ACE-inhibitory activity in the hydrolysates.
The polynomial equation shows that the individual effect of E/S is the most influencing factor and the pH and temperature are next. The 3D response surface of three variables is described in . Curves can be obtained by changing two variables and fixing the other one variable at a central value codified as value 0. The maximum response was achieved as pH of 9.01, temperature of 61.81 °C and E/S of 6.5% corresponding to the predicted Y value of 85.13%. Subsequently, the experimental activity tended to be 85.02%. The optimized hydrolysis condition could be helpful to produce peptides.
ACE-inhibitory peptides purification
Previous studies showed that the majority of peptide was oligopeptide and normally occurred in a fraction under the lowest molecular weight ( < 1 kDa) [Citation20,Citation21]. In this study, the purification processes were traditionally operated. Milk hydrolysis was first separated to three sections of U1 < 10 kDa, U2 < 5 kDa and U3 < 1 kDa. The ACE-inhibitory activity increased from initial 85.22% to 88.45% in fraction U3 (). The obtained fraction D after macroporous resin DA201-C separation showed ACE-inhibitory activity up to 91.9%. Further purification was operated on Sephadex G-25 and G-15 column, as shown in . The ACE-inhibitory activity of fraction G2 after G-25 purification was 92.23%. Fraction G2-2 expressed the best-performing activity of 92.7% (IC50 = 167.33 μg/mL) when purified on G-15 column ().
Identification of purified peptides from milk hydrolysates
The significance of amino acid sequences in binding to ACE attracted the global attentions. So far, the favourable peptides contain hydrophobic amino acids and aromatic amino acids on the C-terminal including Trp, Tyr, Phe and Pro [Citation22]. These amino acids formed bonds magically, which might resistant to human proteolytic enzymes, thereby playing a role in reaching the object point. Therefore, peptides normally containing such amino acids bonds exhibited high activity in vitro and maintained high intestinal stability. However, some peptides with distinct sequence, such as SGGSYADELVSTAK from wheat germ protein hydrolysates, TTFHTSGY from α-lactalbumin hydrolysate, and QLLLQQ from horse gram hydrolysate, exhibited great ACE-inhibitory activity [Citation23–25].
In this work, main amino acids of fraction G2-2 was Tyr, Phe, Glu, Ala, Val and Pro (). The data are presented as percent molar composition for each residue. Ala was presented in the highest mol content of approximately 21.09%, and followed Tyr of 18.31%, Phe of 13.79%, Glu of 8.52%, Val of 6.75%, Met of 5.49% and Pro of 4.68%. The molar percentage of hydrophobic amino acids and aromatic amino acid in G2-2 was 76.59% and 31.86%, respectively, which suggested this part may be the ACE-activity inhibitor.
In , two peptides were identified from fraction G2-2 and listed as VLPVPQ and VAPFPE (). The molecular mass of P1 VLPVPQ was 652 Da. It was similar to an ACE-inhibitory peptide KVLPVPQ, which was purified by Maeno. This peptide KVLPVPQ possessed potent antihypertensive efficacy in spontaneously hypertensive rats (SHRs) but exhibited low ACE-inhibitory activity. Interestingly, a similar peptide KVLPVP showed stronger ACE-inhibitory activity by pancreatic digestion [Citation26].
Table 3. Comparative analysis of ACE-inhibitory peptides obtained in this study and others.
Another peptide P2 was identified as VAPFPE, which was similar to a peptide identified by Ong et al. [Citation27]. They isolated a peptide FVAPFPEVF from cheddar cheese, showing in vivo antihypertensive activity. The relevancy of structure and activity of peptide was still under construction while emerging evidence helps us better understand it [Citation28]. A shorter peptide LHLPLP showed higher activity when it was obtained from the peptide with the sequence of NLHLPLPLL, VENLHLPLPLL or ENLHLPLPLL [Citation29]. Quirós et al. [Citation30] doubled the activity of an ACE-inhibitory peptide by replacing Pro at C-terminal position to Arg (LHLPLR).
Molecular docking
A docking simulation was carried out to demonstrate the molecular mechanism of the purified peptides. Previous reports concluded that the ACE inhibitor mainly interacted with some active spot pockets of S1, S2 and S1′. S1 contained Ala354, Tyr523 and Glu384 residues, while S2 included Gln281, His353, Lys511 and Tyr520 residues, and S1′ contained Glu162 residues [Citation31].
depicts the 2D structures of peptides binding with ACE. In case of P1, the binding site was predicted as His387, Tyr523 and Asn66 with hydrogen bonds. Furthermore, expresses the positive correlation of the P1 and Zn(II) of ACE. Since ACE was one of the zinc proteases, Zn2+ played a critical part in ACE activity, combining to ACE residues Glu411, His383 and His387 [Citation31]. Our peptide P1 showed an close interaction with the S1 pocket and Zn(II) of ACE, which indicated that P1 was a strong candidate of ACE inhibitor. In terms of P2, the docking study showed that it formed hydrogen bonds with His353, His513 and Thr282, suggesting that P2 was involved in S2 active site pocket of ACE (. It was found that a traditional drug of Lisinopril combined with Ala354, His353 and Glu384, and also can be direct connect with the Zn(II) of ACE [Citation32]. ACE-inhibitory peptides exerted a relatively low activity in comparison with medicinal products, but dramatically alleviate side-effects. In addition, food-intake ACE-inhibitory peptides are generally accepted because of the accessible and safe raw materials. Our milk-derived peptides of P1 and P2 can be considered to prevent hypertension through diet.
Conclusions
In this study, a mathematical model for complex enzyme hydrolysis of skim bovine milk was established. The complex enzymes were subjected to alcalase and protease from Bacillus licheniformis with a ratio of around 1:1. The enzymatic hydrolysis condition was optimized as pH of 9.01, temperature of 61.81 °C and E/S of 6.5% corresponding to ACE-inhibitory activity of 85.02%. Fraction G2-2 with IC50 value of 167.33 μg/mL was obtained. Main amino acids of fraction G2-2 was Tyr, Phe, Glu, Ala, Val and Pro. Two novel ACE-inhibitory peptides were identified by Q-Exactive LC–MS/MS and listed as VLPVPQ and VAPFPE. The molecular docking results recovered that these novel peptides combined with the S1 and S2 pockets and Zn(II) of ACE greatly. Whether these novel ACE-inhibitory peptides possess the potent antihypertensive efficacy in SHRs is included in future research programme. We provide an efficient and practical enzymatic combination of alcalase and protease for the milk-derived ACE-inhibitory peptides production. Our novel ACE-inhibitory peptides could potentially develop the functional foods for hypertension therapy.
Acknowledgements
We appreciate Dr. Jing Wang from the Northwest University for the aid of molecular docking study.
Disclosure statement
No potential conflict of interest was reported by the authors.
Additional information
Funding
References
- Dzudie A, Rayner B, Ojji D, et al. Roadmap to achieve 25% hypertension control in Africa by 2025. Global Heart. 2018;13(1):45–59.
- Nagarajan N, Jalal D. Resistant hypertension: diagnosis and management. Adv Chronic Kidney Dis. 2019;26(2):99–109.
- Benjamin EJ, Blaha MJ, Chiuve SE, et al. Heart disease and stroke statistics-2017 update: a report from the American Heart Association. Circulation. 2017;135(10):e146–e603.
- Wang Y, Jiang YQ, Yin YG, et al. Identification and inhibitory mechanism of angiotensin I-converting enzyme inhibitory peptides derived from bovine hemoglobin. Protein J. 2017;36(3):166–173.
- He R, Wang YJ, Yang YJ, et al. Rapeseed protein-derived ACE inhibitory peptides LY, RALP, and GHS show antioxidant and anti-inflammatory effects on spontaneously hypertensive rats. J Funct Foods. 2019;55:211–219.
- O’Keeffe MB, FitzGerald RJ. Identification of short peptide sequences in complex milk protein hydrolysates. Food Chem. 2015;184:140–146.
- Udenigwe CC, Aluko RE. Food protein-derived bioactive peptides: production, processing, and potential health benefits. J Food Sci. 2012;77(1):R11–R24.
- Zhang GS, Ding WY, Wang Y, et al. Optimization of casein hydrolyzed by alcalase and trypsin using response surface methodology. Food Sci. 2013;34:237–241.
- Espejo-Carpio FJ, Gobba DC, Guadix A, et al. Angiotensin I-converting enzyme inhibitory activity of enzymatic hydrolysates of goat milk protein fractions. Int Dairy J. 2013;32(2):175–183.
- Nongonierma AB, O’Keeffe MB, FitzGerald RJ, et al. Milk protein hydrolysates and bioactive peptides. In: McSweeney PLH, O’Mahony JA, editors. Advanced dairy chemistry. New York: Springer, 2016. p. 417–482.
- Norris R, O’Keeffe MB, Poyarkov A, et al. Peptide identification and angiotensin converting enzyme (ACE) inhibitory activity in prolyl endoproteinase digests of bovine a s-casein. Food Chem. 2015;188:210–217.
- Jimsheena VK, Gowda LR. Arachin derived peptides as selective angiotensin I-converting enzyme (ACE) inhibitors: structure–activity relationship. Peptides. 2010;31(6):1165–1176.
- Adamson NJ, Reynolds EC. Characterization of casein phosphopeptides prepared using alcalase: determination of enzyme specificity. Enzyme Microb Technol. 1996;19(3):202–207.
- Lowry OH, Rosebrough NJ, Farr AL, et al. Protein measurement with the folin phenol reagent. J Biol Chem. 1951;193(1):265–275.
- Cushman DW, Cheung HS. Spectrophotometric assay and properties of the angiotensin-converting enzyme of rabbit lung. Biochem Pharmacol. 1971;20(7):1637–1648.
- Jiang J, Chen S, Ren F, et al. Yak milk casein as a functional ingredient: preparation and identification of angiotensin-I-converting enzyme inhibitory peptides. J Dairy Res. 2007;74(1):18–25.
- Lee KJ, Kim SB, Ryu JS, et al. Separation and purification of angiotensin converting enzyme inhibitory peptides derived from goat’s milk casein hydrolysates. Asian Austral J Anim Sci. 2005;18:741–746.
- Mullally MM, Meisel H, FitzGerald RJ. Angiotensin-I-converting enzyme inhibitory activities of gastric and pancreatic proteinase digests of whey proteins. Int Dairy J. 1997;7(5):299–303.
- Mullally MM, Meisel H, FitzGerald RJ. Identification of a novel angiotensin-I-converting enzyme inhibitory peptide corresponding to a tryptic fragment of bovine β-lactoglobulin. FEBS Lett. 1997;402(2–3):99–101.
- Matsui T, Matsufuji H, Seki E, et al. Inhibition of angiotensin-I-converting enzyme by Bacillus licheniformis alkaline protease hydrolyzates derived from sardine muscle. Biosci Biotechnol Biochem. 1993;57(6):922–927.
- Zhuang YL, Sun LP, Li BF. Production of the angiotensin-I-converting enzyme (ACE)-inhibitory peptide from hydrolysates of jellyfish (Rhopilema esculentum) collagen. Food Bioprocess Technol. 2012;5(5):1622–1629.
- Saito Y, Wanezaki K, Kawato A, et al. Structure and activity of angiotensin I converting enzyme inhibitory peptides from sake and sake lees. Biosci Biotechnol Biochem. 1994;58(10):1767–1771.
- Karami Z, Peighambardoust SH, Hesari J, et al. Antioxidant, anticancer and ACE-inhibitory activities of bioactive peptides from wheat germ protein hydrolysates. Food Biosci. 2019;32:100450.
- Villadóniga C, Cantera A. New ACE-inhibitory peptides derived from α-lactalbumin produced by hydrolysis with Bromelia antiacantha peptidases. Biocatal Agric Biotechnol. 2019;20:101258.
- Bhaskar B, Ananthanarayan L, Jamdar S. Purification, identification, and characterization of novel angiotensin I-converting enzyme (ACE) inhibitory peptides from alcalase digested horse gram flour. LWT-Food Sci Technol. 2019;103:155–161.
- Maeno M, Yamamoto N, Takano T. Identification of an antihypertensive peptide from casein hydrolysate produced by a proteinase from Lactobacillus helveticus CP790. J Dairy Sci. 1996;79(8):1316–1321.
- Ong L, Henriksson A, Shah NP. Proteolytic pattern and organic acid profiles of probiotic Cheddar cheese as influenced by probiotic strains of Lactobacillus acidophilus, Lb. paracasei, Lb. casei or Bifidobacterium sp. Int Dairy J. 2007;17(1):67–78.
- FitzGerald RJ, Murray BA, Walsh DJ. Hypotensive peptides from milk proteins. J Nutr. 2004;134(4):980S–988S.
- Robert MC, Razaname A, Mutter M, et al. Identification of angiotensin-I-converting enzyme inhibitory peptides derived from sodium caseinate hydrolysates produced by Lactobacillus helveticus NCC 2765. J Agric Food Chem. 2004;52(23):6923–6931.
- Quirós A, Contreras MdM, Ramos M, et al. Stability to gastrointestinal enzymes and structure–activity relationship of β-casein-peptides with antihypertensive properties. Peptides. 2009;30(10):1848–1853.
- Ko S-C, Jang J, Ye B-R, et al. Purification and molecular docking study of angiotensin I-converting enzyme (ACE) inhibitory peptides from hydrolysates of marine sponge Stylotella aurantium. Process Biochem. 2017;54:180–187.
- Pan DD, Guo HQ, Zhao B, et al. The molecular mechanisms of interactions between bioactive peptides and angiotensin-converting enzyme. Bioorg Med Chem Lett. 2011;21(13):3898–3904.