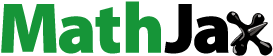
Abstract
Nickel-ferrite (NiFe2O4) nanorods particles (NRP) was biosynthesised for the first time by the Rosemary Extract. The NRP was fully characterised, including the type, nanostructure and physicochemical properties of using XRD, HRTEM, FeSEM, XPS, FTIR and VSM. TEM confirmed rod-shaped nano-sized particles with average sizes ranging from 10 nm to 28 nm. The EDAX Analysis showed the presence of iron, nickel, oxygen, and carbon. XRD analysis confirmed the synthesis of NiFe2O4 crystals. XPS curves showed photoelectron for iron, oxygen and nickel. EDS showed the atomic, weight percentages ratios of Ni(12%): Fe(24%) and: O(48) are close to the theoretical value (Ni: Fe:O = 1:2:4), of bimetallic magnetic NiFe2O4 NRP. NiFe2O4 NRP had cytotoxicity effect on MCF-7 cells survival which suggests that NiFe2O4 NRP can be used as a new class of anticancer agent in design novel cancer therapy research.
Introduction
The past decade has been a huge surge in the applications of the metallic based nanoparticles (NP) ranging from biomedical applications to other industries such as catalytic water treatment or electronic. Nowadays, extensive research into the production of metal nanostructures and study the application of them is widely developed [Citation1–4]. Among the various types of nanostructures produced, NP of iron, cobalt and nickel are considered for magnetic reasons [Citation5].
Magnetic NPs have attracted a lot of attention due to their unique properties, especially magnetic and electromagnetic ones, and have been used in various applications [Citation6–10]. Magnetic materials based on spinel ferrite with the general formula MFe2O4(M: divalent metal ion, e.g. Mn, Mg, Zn, Ni, Co, Cu, etc) [Citation11] due to their unique properties, have excellent potential for application in electronics [Citation12], catalyst [Citation13], battery [Citation14], magnetic storage devices and various medical fields such as antibacterial agents [Citation15], immunoassays [Citation16], hyperthermia therapy [Citation17] and Magnetic resonance imaging [Citation18]. One of the most important and attractive nanostructures of spinel ferrite is the nickel-ferrite (NiFe2O4). This attraction is because of their saturation magnetisation and paramagnetic behaviour, super-paramagnetism and unique magnetic structure [Citation19]. These properties are strongly dependent on the shape, chemical composition and of size NP that can be determined by the synthesis process. In order to produce NP with the desired physico-chemical properties, research and development of various methods for the synthesis of NP is being carried out using various methods [Citation20–30]. Different synthesis methods have been reported for the production of NiFe2O4 bimetallic nanostructures. A lot of efforts have been put to build NiFe2O4 nanostructures using co-precipitation [Citation22], sol-gel [Citation23], electrospinning [Citation14], combustion [Citation31] and microwave [Citation25] methods. Sivakumar et al. [Citation32] synthesised NiFe2O4 bimetallic NP using ethyl acetate solution with co-precipitation method. Ethyl acetate is flammable and toxic, and this chemical can damage internal organs if it is exposed repeatedly or prolonged. Ethyl acetate can also cause irritation to the eyes or skin [Citation32,Citation33].
Nejati and Zabihi [Citation34] were synthesised NiFe2O4 bimetallic NP by the hydrothermal method using Hydrazine hydrate. Hydrazine hydrate is toxic to humans [Citation35]. Current mentioned chemical methods usually have disadvantages such as dependence on expensive equipment, high energy consumption and dependence on chemical compounds toxic to the environment and humans [Citation36–43]. Therefore, finding simple, economical, inexpensive, non-toxic and environmentally friendly methods are very important and necessary for the synthesis of these types of NP. A method that can eliminate the need to use any harmful chemicals is shown in [Citation44]. The methods that recently attracted the attention of scientists are synthesis of nanostructures using natural resources such as herbal, bacterial, fungal or their derivative (green methods). Compared with chemical synthesis methods, green methods have various important advantages, including facility, low external energy consumption, affordable, rapid synthesis process, non-toxicity, convenient one-step process and eco-friendly [Citation45–49].
The aim of this study was the development of a novel technique of synthesising of bimetallic NiFe2O4 NRP using natural resources. For the first time, NiFe2O4 NRP was synthesis using rosemary. The use of pharmaceutical grade rosemary extract provides an alternative method for the rapid, one-step process and low external energy consumption for the production of NiFe2O4 NRP.
Materials and methods
Synthesis of NiFe2O4 nanorod particles
Initially rosemary young leaves were washed in distilled water containing Sodium hypochlorite (Merck, Germany) and then placed at room temperature to remove the surface moisture. 200 g of rosemary healthy leaves were added to 1000 mL of deionised water and heated at 80° C for period of one hour, then filtered with Whatman filter paper with the size No. 40.
NiFe2O4 NRP were synthesised by green and sustainable process using natural plant extract. For synthesis of NiFe2O4 nanostructure, 0.5 g of FeCl3.6H2O (98%) and 0.1 g of NiCl2.6H2O (98%, Merck) were dissolved in 30 mL of rosemary extract at 70° C under vigorous stirring. Then, Na2CO3 (≥99.5%) 1 M solution used to bring pH to 7.5 and stirred at 25 °C for 3 h. Finally, NRP were washed with ethanol followed by deionised water three times each and then dried in oven (at 60° C for 12 h).
Characterisation of NiFe2O4 nanorod particles
High-resolution Transmission electron microscope (HRTEM, HRTEM, FEI, Tecnai 20) was used to study the structure and morphology of metallic NP. Field emission scanning electron microscopy (FESEM, MIRA3, TE-SCAN,Czech Republic) were used to study the morphology and size of resulting NiFe2O4 NRP. The chemical composition of the NRP was determined using Energy Dispersive X-Ray Spectroscopy (EDS). Strength of magnetic behaviour of NP was investigated using a vibrating-sample magnetometer (VSM, LBKFB model-Meghnatis Daghigh Kavir Company). The X-ray powder diffraction (XRD) was use to analyse the crystal structure of synthesised NRP using X′PertPro, Panalytical (Made in Netherland) with Cu Kα radiation (λ = 1.54 Å). Fourier-transform infra-red spectroscopy (FTIR) spectra of the rosemary extract alone and greener synthesis NiFe2O4 NRP was done to evaluate possible functional groups involved in the synthesis process. This analysis obtained using an FTIR spectrometer (TENSOR II, Bruker, Germany) in the range of 400–4000 cm−1. The X-ray photoelectron spectroscopy (XPS) was done for studying the surface chemistry of a NRP by Thermofisher Scientific K-Alpha (Thermofisher Scientific K-Alpha).
Cytotoxicity investigation of NiFe2O4 nanorod particles
The human breast cancer (MCF-7) cell lines were cultured in Dulbecco’s Modified Eagle Medium (DMEM, Sigma-Aldrich) supplemented with 100 µg/mL of streptomycin (Sigma-Aldrich), 10% foetal bovine serum (Sigma-Aldrich) and 100 IU/mL of penicillin (Invitrogen, UK).
104 cells/well of MCF-7 cell lines were seeded on the plates (96 well plate) and kept at 37 °C with 5% CO2. After 24 h, the medium was aspirated and 100 µL NiFe2O4 nanorod particles with concentrations of 2, 4, 8, 16, 32, 64, 128, 256 and 512 μg/mL were added to each well, separately. Then the 96 well plates were incubated for 24 h at 37 °C with 5% CO2. The WST-1 (10 µL) was added to the wells, and then plates incubated for 4 h. Finally the optical density (OD) was measured at 420–580 nm using ELISA (BioTeks Elx 800).
The survival rate (%) was calculated using following EquationEquation (1)(1)
(1) .
(1)
(1)
Results
The X-ray diffraction pattern of NiFe2O4 NRP is shown in . XRD spectrum showed peaks in 2θ around 30.02°, 35.67°, 38.19° 54.01°, 57.98° and 62.92° that are corresponded to the 220, 311, 222, 422, 511 and 440 reflection planes of the NiFe2O4, respectively [Citation50]. The three peaks are 311, 222 and 440 planes of the spinel NiFe2O4 structure [Citation51,Citation52]. The phenolic compounds of the rosemary extract and extensive of the XRD pattern expresses the size of very fine particles and the background of amorphous [Citation53,Citation54]. The results are close of the previously published reported of NiFe2O4 NRP XRD pattern [Citation32,Citation55–58]
FTIR analysis
shows the functional groups involved in the synthesis process. The broad absorption band at 3448.92 cm−1, 400–700 cm−1 and the peak around 1637.72 cm−1 were related to O–H functional group, bending out of plane C-H and stretching vibrations of conjugated C–C of benzenoid ring, respectively, in rosemary extract [Citation59]. The intensity of these bands decreases significantly in NiFe2O4 NRP curve. The FTIR absorption band at ≃600 cm−1 (v1), corresponds to intrinsic stretching vibrations of the metal ion at the tetrahedral site, (M − O) and absorption band at around ≃450 cm−1 (v2) is assigned to octahedral metal stretching vibration (M − O; Fe3+ ↔ O and Ni2+ ↔ O) [Citation60].
Figure 2. FTIR spectrum of rosemary extract alone (1, blue) and greener synthesised of NiFe2O4 nanorod particle (2, red).
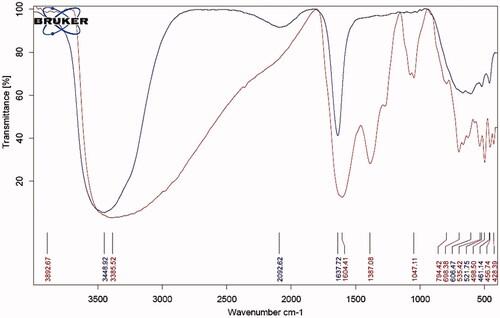
The force constant of octahedral (Kocta) and the tetrahedral (Ktetra) site have been estimated for v1 and v2 by using the Waldron [Citation61] suggested EquationEquation (2)(2)
(2) :
(2)
(2)
“K” is the force constant, “v” is the corresponding wave number, “M” is the atomic mass, “C” is speed of light (free space).
In FTIR spectra of greener NiFe2O4 NRP the band at 3385.52 cm−1 are attributed to the H–OH bending vibration of the free hydroxyl groups of phenolic compounds of can be due to the free or absorbed water on the surface of ferrite nanoparticles [Citation62]. The band at 1604.41 cm−1 are attributed to the H–OH bending stretching of the carboxylic acid [Citation54,Citation55]. The broad absorption band at higher ∼600 cm−1 corresponds to intrinsic stretching vibrations of the metal at the tetrahedral complexes (Fe ↔ O) and the absorption band lower at ∼400 cm−1 corresponds to the vibrations of octahedral complexes (Ni ↔ O) [Citation52]. These two absorption bands show very small particles of spinel ferrites [Citation63,Citation64].
FESEM and EDS was used to observe the morphology and chemical composition of bimetallic magnetic NiFe2O4 NRP. The results () show the synthesis of rod-shaped particles with the particle size of 40–200 nm and a diameter of about 5 nm. The EDS analysis confirmed the presence of Ni, Fe and O elements in the NiFe2O4 NRP sample ().
EDS spectra () of the bimetallic magnetic NiFe2O4 NRP showed the atomic, weight percentages ratios of Ni(12%): Fe(24%) and: O(48) are close to the theoretical value (Ni: Fe:O = 1:2:4), suggesting purity of green synthesised bimetallic magnetic NiFe2O4 NRP. The content of C element is due to the residual organic matters (plant extract).
In are shown the results obtained from HRTEM and SAED analyses. HRTEM micrograph identified the of NiFe2O4 rod-like nanostructure (). The EDS chemical composition analysis confirmed the presence of Ni, Fe and O elements in the NiFe2O4 NRP sample (). The selected area electron diffraction (SAED) pattern shows diffuse rings matches well with (222), (311) and (440) NiFe2O4 planes. Also, the overlapping of individual rings indicates the presence of amorphous nanorod [Citation55,Citation65].
Figure 4. HRTEM images at different scale (a), EDS results (b) and SAED patterns (c) of bimetallic magnetic NiFe2O4 nanorods particles s synthesis using Rosemary.
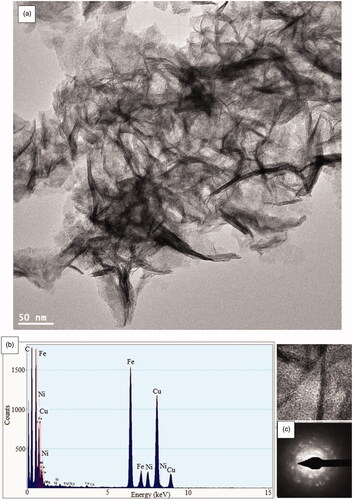
The shows the composition of the surface chemistry of NiFe2O4 NRP samples using XPS. The peak over binding energy curves showed photoelectron for iron (Fe 2p3/2, at 711 eV and Fe 2p1/2, 725 eV), oxygen (Fe 2p1/2 at 725 eV), and nickel, Ni 2p showed two spit-orbit peaks in the range of Ni 2p1/2 at 874 eV) and Ni 2p3/2 at 856 eV). In addition the presence of two peaks 874 and 856 with two peaks 879 and 862 is shows presence ions Ni2+/Ni3+ on the of the surface of the NiFe2O4 NRP. This has leads significant increase in electro-catalytic activity in the synthesis of NiFe2O4 NRP.
The magnetic properties of bimetallic magnetic NiFe2O4 NRP were investigated by VSM. Greener synthesised NiFe2O4 NRP showed a magnetic saturation value of 0.54 emu g−1 at room temperature (). The saturation values for bulk of Fe, Ni and NiFe2O4 and are 221.7, 54.4 and 56 emu/g, respectively [Citation31,Citation66]. Reduction of the magnetisation for greener NiFe2O4 NRP can be due to formation thin oxide layer, the surface spin disorder and the size confinement [Citation66].
shows the toxicity of the NiFe2O4 NRP does rate. The increase in NiFe2O4 NRP over 4 μg/mL significantly effect of MCF-7 cancer cells studied survival, at the concentration of the 500 µg/mL, there was over 55% cells died.
Discussion
The results of this study revealed a successful self-assembly NiFe2O4 NRP using a green, simple, non-toxic, low-cost synthesis methodologies using rosemary extracts. XRD spectrum of NiFe2O4 NRP showed peaks in 2θ around 30.02°, 35.67°, 38.19° 54.01°, 57.98° and 62.92° that are corresponded to the 220, 311, 222, 422, 511 and 440 reflection planes of the NiFe2O4, respectively. The three peaks are 311, 222 and 440 planes of the spinel NiFe2O4 structure. HRTEM micrograph identified the of NiFe2O4 rod-like nanostructure. Greener synthesised NiFe2O4 NRP showed a magnetic saturation value of 0.54 emu g–1 at room temperature. NiFe2O4 nano-sized particles are of great attention due to their applications in environment, medicine and energy [Citation67]. The proposed method of the synthesis provides less risk to the human health and environment and due to the low cost higher applications. In our knowledge, this is the first time rosemary extract has been used to synthesis NiFe2O4 with nanorod shape structures. EDS spectra of the bimetallic magnetic NiFe2O4 NRP showed the atomic, weight percentages ratios of Ni(12%): Fe(24%) and: O(48) are close to the theoretical value (Ni: Fe:O = 1:2:4), suggesting purity of green synthesised bimetallic magnetic NiFe2O4 NRP. The content of C element is due to the residual organic matters (plant extract).
We have a serious lack of investigation regarding the application of NiFe2O4 NP in medicine. Our result showed that NiFe2O4 nanorod particles with cytotoxicity activity can be used as a new class of anticancer agent in design novel cancer therapy research.
The use of pharmaceutical rosemary provides an alternative method for the one-step rapid process and low external energy consumption and non-toxicity synthesis of NiFe2O4 nanorod particles. The green synthesis of NiFe2O4 NP using rosemary avoids the need for harmful reducing or capping agents.
There are few publications attempted to develop NiFe2O4 NRP, using verity of the methods, but the synthesis technique either has been complex or not high-quality end products. Examples are the synthesis of NiFe2O4 nanorod shape particles using Urtica plant extract [Citation62], but they had to use a hydrothermal process by employing Teflon autoclave. Their TEM results showed that their NPs are agglomerated with irregular morphology. However cannot recognise a rod-shaped NP in their SEM or TEM results. Other attempted to synthesis NiFe2O4/Fe2O3/CeO2 nanocomposite using a walnut green hull extract with calcinated at 600 °C [Citation68]. Their results showed that their NPs are highly agglomerated with irregular morphology. Our technique of production of the NiFe2O4 NRP is simple and cost effective by rosemary plants without using any external device, calcination or chemicals. We have fully characterised the product and showed that regular rod morphology of NiFe2O4 nano-sized particles without agglomeration. In term of the cytotoxicity, our results are similar to others. showed the schematic of the green and sustainable process for NiFe2O4 nano-rod particles.
Conclusion
We have developed a NiFe2O4 nanorods particles using greener and cost-effective synthesised methodologies using for first time Rosemary extract. The application of metallic nanoparticles in biomedicine and environmental are a multibillion-dollar. This technique helps the industries application faster to the end products. The type, structure and physicochemical properties of nanostructures produced were studied using HRTEM, XRD, FeSEM, XPS, VSM and FTIR. Moreover, NiFe2O4 NP had cytotoxicity effect on MCF-7 cells survival which suggests that NiFe2O4 NP can be used as a new class of anticancer agent in design novel cancer therapy research. NiFe2O4 nanorod particles can be used to increase the level of public health. Bimetallic magnetic NiFe2O4 NP synthesis using Rosemary should be used in design for biomedical applications such as medical senor or antibacterial application.
Authors contributions
The authors read and approved the final manuscript.
Abbreviations | ||
Fig. | = | Figure |
mL | = | Millilitre |
nm | = | Nanometre |
FeCl3.6H2O | = | Ferric chloride hexahydrate |
NiCl2.6H2O | = | Nickel(II) chloride hexahydrate |
Na2CO3 | = | Sodium carbonate |
XRD | = | X-ray diffraction |
NiFe2O4 | = | Nickel ferrite |
HRTEM | = | High-resolution Transmission electron microscope |
FESEM | = | Field emission scanning electron microscopy |
VSM | = | vibrating-sample magnetometer |
FTIR | = | Fourier-transform infra-red spectroscopy |
XPS | = | X-ray photoelectron spectroscopy |
DMEM | = | Dulbecco’s Modified Eagle Medium |
Acknowledgments
This research was carried out using the funding and support of Research and Technology Institute of Plant Production of Shahid Bahonar University of Kerman.
Disclosure statement
No potential conflict of interest was reported by the authors.
References
- Khorasani-Motlagh M, Noroozifar M, Jahani S. Preparation and characterization of nano-sized magnetic particles LaCoO3 by ultrasonic-assisted coprecipitation method. Synth React Inorg M. 2015;45(10):1591–1595.
- Singh P, Kim Y-J, Zhang D, et al. Biological synthesis of nanoparticles from plants and microorganisms. Trends in Biotechnol. 2016;34(7):588–599.
- Singh H, Du J, Singh P, et al. Extracellular synthesis of silver nanoparticles by Pseudomonas sp. THG-LS1.4 and their antimicrobial application. J Pharm Anal. 2018;8(4):258.
- Alijani HQ, Pourseyedi S, Torkzadeh Mahani M, et al. Green synthesis of zinc sulfide (ZnS) nanoparticles using Stevia rebaudiana Bertoni and evaluation of its cytotoxic properties. J Mol Struct. 2019;1175:214–218.
- Rathi A, Meka VM, Jayaraman TV. Synthesis of nanocrystalline equiatomic nickel-cobalt-iron alloy powders by mechanical alloying and their structural and magnetic characterization. J Magn Magn Mater. 2019;469:467–482.
- Boruah PK, Borthakur P, Das MR. Magnetic metal/metal oxide nanoparticles and nanocomposite materials for water purification. In: Nanoscale materials in water purification. Netherlands: Elsevier; 2019. p. 473–503.
- Khatami M, Iravani SRS, Varma RV, et al. Cockroach wings-promoted safe and greener synthesis of silver nanoparticles and their insecticidal activity. Bioprocess Biosyst Eng. 2019;42(12):2007–2014.
- Gholami L, Kazemi Oskuee R, Tafaghodi M, et al. Green facile synthesis of low-toxic superparamagnetic iron oxide nanoparticles (SPIONs) and their cytotoxicity effects toward Neuro2A and HUVEC cell lines. Ceram Int. 2018;44(8):9263.
- Seddighi NS, Salari S, Izadi AR. Evaluation of antifungal effect of iron-oxide nanoparticles against different Candida species. IET Nanobiotechnol. 2017;11(7):883.
- Mirzaei H, Nasiri AA, Mohamadee R, et al. Direct growth of ternary copper nickel cobalt oxide nanowires as binder-free electrode on carbon cloth for nonenzymatic glucose sensing. Microchem J. 2018;142:343–351.
- Chaibakhsh N, Moradi-Shoeili Z. Enzyme mimetic activities of spinel substituted nanoferrites (MFe2O4): a review of synthesis, mechanism and potential applications. Mat Sci Eng C. 2019;99:1424–1447.
- Demir L, Perişanoğlu U, Şahin M. Investigating XRF parameters and valance electronic structure of the Co, Ni, and Cu spinel ferrites. Ceram Int. 2019;45(6):7748–7753.
- Anwar A, Yousuf MA, Tahir B, et al. New Er3+-substituted NiFe2O4 nanoparticles and their nano-heterostructures with graphene for visible light-driven photo-catalysis and other potential applications. CNANO. 2019;15(3):267–278.
- Cherian CT, Sundaramurthy J, Reddy M, et al. Morphologically robust NiFe2O4 nanofibers as high capacity Li-ion battery anode material. ACS Appl Mater Interfaces. 2013;5(20):9957–9963.
- Jose J, Thomas S, Kalarikkal N, et al. Antimicrobial properties of MFe2O4 (M = Mn, Mg)/reduced graphene oxide composites synthesized via solvothermal method. Mat Sci Eng C. 2019;95:43–48.
- Karmakar S, Routray KL, Panda B, et al. Construction of core@ shell nanostructured NiFe2O4@ TiO2 ferrite NAND logic gate using fluorescence quenching mechanism for TiO2 sensing. J Alloys Compd. 2018;765:527–537.
- Sangeetha K, Ashok M, Girija E. Development of multifunctional cobalt ferrite/hydroxyapatite nanocomposites by microwave assisted wet precipitation method: a promising platform for synergistic chemo-hyperthermia therapy. Ceram Int. 2019;45(10):12860.
- Hankiewicz J, Stoll J, Stroud J, et al. Nano-sized ferrite particles for magnetic resonance imaging thermometry. J Magn Magn Mater. 2019;469:550–557.
- Sivakumar P, Ramesh R, Ramanand A, et al. Preparation and properties of nickel ferrite (NiFe2O4) nanoparticles via sol–gel auto-combustion method. Mater Res Bull. 2011;46(12):2204–2207.
- Chen D-H, He X-R. Synthesis of nickel ferrite nanoparticles by sol-gel method. Mater Res Bull. 2001;36(7–8):1369–1377.
- Srivastava M, Chaubey S, Ojha AK. Investigation on size dependent structural and magnetic behavior of nickel ferrite nanoparticles prepared by sol–gel and hydrothermal methods. Mater Chem Phys. 2009;118(1):174–180.
- Maaz K, Karim S, Mumtaz A, et al. Synthesis and magnetic characterization of nickel ferrite nanoparticles prepared by co-precipitation route. J Magn Magn Mater. 2009;321(12):1838–1842.
- Pérez E, Marquez G, Sagredo V. Effect of calcination on characteristics of nickel ferrite nanoparticles synthesized by sol-gel method. Iraqi J App Phy. 2019;15(1):13–17.
- Udhaya PA, Bessy T, Meena M. Antibacterial activity of nickel and magnesium substituted ferrite nanoparticles synthesized via self-combustion method. Mater Today Proceedings. 2019;8:169–175.
- Kombaiah K, Vijaya JJ, Kennedy LJ, et al. Catalytic studies of NiFe2O4 nanoparticles prepared by conventional and microwave combustion method. Mater Chem Phys. 2019;221:11–28.
- Rahi A, Sattarahmady N, Heli H. Zepto-molar electrochemical detection of Brucella genome based on gold nanoribbons covered by gold nanoblooms. Sci Rep. 2016;5(1):18060.
- Vais RD, Sattarahmady N, Heli H. Green electrodeposition of gold nanostructures by diverse size, shape, and electrochemical activity. Gold Bull. 2016;49(3–4):95–102.
- Hossein H, Masoud N. Applications of nanoflowers in biomedicine. Recent Pat Nanotech. 2017;11:1–12.
- Dağlıoğlu Y, Yılmaz Öztürk B. A novel intracellular synthesis of silver nanoparticles using Desmodesmus sp. (Scenedesmaceae): different methods of pigment change. Rend Fis Acc Lincei. 2019;30(3):611–621.
- Minhas FT, Arslan G, Gubbuk IH, et al. Evaluation of antibacterial properties on polysulfone composite membranes using synthesized biogenic silver nanoparticles with Ulva compressa (L.) Kütz. and Cladophora glomerata (L.) Kütz. extracts. Int J Biol Macromol. 2018;107:157–165.
- Kooti M, Sedeh AN. Synthesis and characterization of NiFe2O4 magnetic nanoparticles by combustion method. J Mater Sci Technol. 2013;29(1):34–38.
- Sivakumar P, Ramesh R, Ramanand A, et al. Preparation of sheet like polycrystalline NiFe2O4 nanostructure with PVA matrices and their properties. Mater Lett. 2011;65(9):1438–1440.
- Tao X, Cush JJ, Garret M, et al. A phase I study of ethyl acetate extract of the chinese antirheumatic herb Tripterygium wilfordii hook F in rheumatoid arthritis. J Rheumatol. 2001;28(10):2160–2167.
- Nejati K, Zabihi R. Preparation and magnetic properties of nano size nickel ferrite particles using hydrothermal method. Chem Cent J. 2012;6(1):23.
- Zelnick SD, Mattie DR, Stepaniak PC. Occupational exposure to hydrazines: treatment of acute central nervous system toxicity. Aviat Space Environ Med. 2003;74(12):1285–1291.
- Miri A, Sarani M. Biosynthesis, characterization and cytotoxic activity of CeO2 nanoparticles. Ceram Int. 2018;44(11):12642.
- Jamdagni P, Rana JS, Khatri P, et al. Comparative account of antifungal activity of green and chemically synthesized Zinc Oxide nanoparticles in combination with agricultural fungicides. Inter J Nano Dimension. 2018;9(2):198–208.
- Hamedi S, Shojaosadati SA, Shokrollahzadeh S, et al. Mechanism study of silver nanoparticle production using Neurospora intermedia. IET Nanobiotechnol. 2017;11(2):157–163.
- Karthik K, Dhanuskodi S, Gobinath C, et al. Multifunctional properties of microwave assisted CdO–NiO–ZnO mixed metal oxide nanocomposite: enhanced photocatalytic and antibacterial activities. J Mater Sci Mater Electron. 2018;29(7):5459–5471.
- Karthik K, Madhukara Naik M, Shashank M, et al. Microwave-assisted ZrO2 nanoparticles and its photocatalytic and antibacterial studies. J Clust Sci. 2018;30(2):311–318.
- Khan FU, Chen Y, Khan NU, et al. Visible light inactivation of E. coli, Cytotoxicity and ROS determination of biochemically capped gold nanoparticles. Microb Pathogene. 2017;107:419–424.
- Khan ZUH, Khan A, Chen Y, et al. Photo catalytic applications of gold nanoparticles synthesized by green route and electrochemical degradation of phenolic Azo dyes using AuNPs/GC as modified paste electrode. J Alloys Compd. 2017;725:869–876.
- Karthik K, Dhanuskodi S, Gobinath C, et al. Fabrication of MgO nanostructures and its efficient photocatalytic, antibacterial and anticancer performance. J Photoch Photobio B. 2019;190:8–20.
- Khan AU, Yuan Q, Khan ZUH, et al. An eco-benign synthesis of AgNPs using aqueous extract of Longan fruit peel: antiproliferative response against human breast cancer cell line MCF-7, antioxidant and photocatalytic deprivation of methylene blue. J Photoch Photobio B. 2018;183:367–373.
- Khan ZUH, Khan A, Chen Y, et al. Biomedical applications of green synthesized Nobel metal nanoparticles. J Photoch Photobio B. 2017;173:150–164.
- Khatami M, Sharifi I, Nobre MAL, et al. Waste-grass-mediated green synthesis of silver nanoparticles and evaluation of their anticancer, antifungal and antibacterial activity. Green Chem Lett Rev. 2018;11(2):125–134.
- Chelli VR, Chakraborty S, Golder AK. Ag-doping on TiO2 using plant-based glycosidic compounds for high photonic efficiency degradative oxidation under visible light. J Mol Liq. 2018;271:380–388.
- Varma RS. Greener approach to nanomaterials and their sustainable applications. Curr Opin Chem Eng. 2012;1(2):123–128.
- Mohammadinejad R, Karimi S, Iravani S, et al. Plant-derived nanostructures: types and applications. Green Chem. 2016;18(1):20–52.
- Kargan H. Synthesis of nickel ferrite nanoparticles by co-precipitation chemical method. Int J Phys Sci. 2013;8(18):854–858.
- Sen P, De A. Electrochemical performances of poly (3, 4-ethylenedioxythiophene)–NiFe2O4 nanocomposite as electrode for supercapacitor. Electrochim Acta. 2010;55(16):4677–4684.
- Maensiri S, Masingboon C, Boonchom B, et al. A simple route to synthesize nickel ferrite (NiFe2O4) nanoparticles using egg white. Scripta Materialia. 2007;56(9):797–800.
- Tong G-X, Liu F-T, Wu W-H, et al. Polymorphous α- and β-Ni(OH)2 complex architectures: morphological and phasal evolution mechanisms and enhanced catalytic activity as non-enzymatic glucose sensors. CrystEngComm. 2012;14(18):5963–5973.
- Wang Z, Fang C, Megharaj M. Characterization of iron–polyphenol nanoparticles synthesized by three plant extracts and their fenton oxidation of azo dye. ACS Sustainable Chem Eng. 2014;2(4):1022–1025.
- Sivakumar P, Ramesh R, Ramanand A, et al. Synthesis and characterization of NiFe2O4 nanoparticles and nanorods. J Alloys Compd. 2013;563:6–11.
- Gabal MA, Kosa S, El Muttairi TS. Magnetic dilution effect of nano-crystalline NiFe2O4 synthesized via sucrose-assisted combustion route. Ceram Int. 2014;40(1):675–681.
- Ahlawat A, Sathe V. Raman study of NiFe2O4 nanoparticles, bulk and films: effect of laser power. J Raman Spectrosc. 2011;42(5):1087–1094.
- Peng T, Zhang X, Lv H, et al. Preparation of NiFe2O4 nanoparticles and its visible-light-driven photoactivity for hydrogen production. Catal Commun. 2012;28:116–119.
- Ghaedi M, Yousefinejad M, Safarpoor M, et al. Rosmarinus officinalis leaf extract mediated green synthesis of silver nanoparticles and investigation of its antimicrobial properties. J Ind Eng Chem. 2015;31:167–172.
- De M, Mukherjee A, Tewari HS. Characterization of cadmium substituted nickel ferrites prepared using auto-combustion technique. PAC. 2015;9(4):193–197.
- Waldron R. Infrared spectra of ferrites. Phys Rev. 1955;99(6):1727.
- Amiri M, Pardakhti A, Ahmadi-Zeidabadi M, et al. Magnetic nickel ferrite nanoparticles: green synthesis by Urtica and therapeutic effect of frequency magnetic field on creating cytotoxic response in neural cell lines. Colloids Surf B. 2018;172:244–253.
- Baykal A, Kasapoğlu N, Köseoğlu Y, et al. CTAB-assisted hydrothermal synthesis of NiFe2O4 and its magnetic characterization. J Alloys Compd. 2008;464(1–2):514–518.
- Salavati-Niasari M, Davar F, Mahmoudi T. A simple route to synthesize nanocrystalline nickel ferrite (NiFe2O4) in the presence of octanoic acid as a surfactant. Polyhedron. 2009;28(8):1455–1458.
- Zhang D, Tong Z, Xu G, et al. Templated fabrication of NiFe2O4 nanorods: characterization, magnetic and electrochemical properties. Solid State Sci. 2009;11(1):113–117.
- Ceylan A, Ozcan S, Ni C, et al. Solid state reaction synthesis of NiFe2O4 nanoparticles. J Magn Magn Mater. 2008;320(6):857–863.
- Zhang Y, Rimal G, Tang J, et al. Synthesis of NiFe2O4 nanoparticles for energy and environment applications. Mater Res Express. 2018;5(2):025023.
- Reza Mohammad Shafiee M, Kargar M, Sadat Hashemi M, et al. Green synthesis of NiFe2O4/Fe2O3/CeO2 nanocomposite in a Walnut Green Hulls extract medium: magnetic properties and characterization. Curr Nanosci. 2016;12(5):645–649.