Abstract
Cerebral ischemia/reperfusion (I/R) injury is a brain injury following ischaemic stroke that is associated with oxidative stress. Taraxasterol, a natural product, has been shown to have anti-oxidative and neuro-protective effects. However, the role of taraxasterol in cerebral I/R injury remains unknown. Primary hippocampal neurons were subjected to oxygen-glucose deprivation/reperfusion (OGD/R) to induce cerebral I/R injury in vitro. Cell viability of hippocampal neurons was measured CCK-8 assay. Reactive oxygen species (ROS) production and MDA generation were measured to reflect oxidative stress. Western blotting was performed to evaluate the expressions of bax, bcl-2, NF-E2-related factor 2 (Nrf2), haem oxygenase (HO-1), NAD(P)H:quinone oxidoreductase 1 (NQO-1) and GPx-3. Caspase-3 activity was measured to assess cell apoptosis. Hippocampal neurons were treated with ML385 to inhibit Nrf2 signalling pathway. Our results showed that taraxasterol improved OGD/R-caused decrease in cell viability of hippocampal neurons. In addition, taraxasterol significantly suppressed ROS production and MDA generation in OGD/R-induced hippocampal neurons. Taraxasterol resulted in a significant decrease in caspase-3 activity and bcl-2 expression, as well as increase in bax expression. Furthermore, taraxasterol induced the Nrf2 nuclear accumulation and expressions of HO-1, NQO-1 and GPx-3 in OGD/R-induced hippocampal neurons. Notably, inhibition of Nrf2 signalling reversed the protective effects of taraxasterol on OGD/R-induced hippocampal neurons injury. In conclusion, these findings indicated that taraxasterol protected hippocampal neurons from OGD/R-induced oxidative stress and cell apoptosis via regulating the Nrf2 signalling pathway.
Introduction
Ischaemic stroke is a common type of stroke characterised by the occlusion of a cerebral blood vessel [Citation1]. Ischaemic stroke remains one of the leading causes of death and disability worldwide. In clinic, it is important to restore the blood reperfusion as early as possible for the prevention of brain damage [Citation2,Citation3]. It may be helpful for the reduction of cerebral ischaemic injury and irreversible damage. However, it has been demonstrated that restoration of blood flow to the ischaemic brain usually cause additional injury in the ischaemic brain, contributing to the overproduction of reactive oxygen species (ROS) [Citation4]. The increased level of ROS triggers a complex array of molecular and cellular processes including the activation of oxidative stress, inflammation and apoptosis-related signalling pathways [Citation5]. These alterations may cause brain injury, thereby resulting in cerebral oedema, brain haemorrhage, and neuronal death, which is termed as cerebral ischemia/reperfusion (I/R) injury [Citation6]. Hence, attenuating ROS production along with oxidative stress represents a key approach in the prevention and treatment of cerebral I/R injury.
Taraxasterol, a pentacyclic-triterpene isolated from Taraxacum officinale, has been shown to have anti-inflammatory, anti-oxidative and neuro-protective effects [Citation7–10]. Taraxasterol suppresses the production of ROS and malondialdehyde (MDA), and increases the glutathione (GSH) level and the activity of superoxide dismutase (SOD) in ethanol-induced mice, implying that taraxasterol possesses protective effects against ethanol-induced liver injury though its anti-oxidative activity [Citation9]. Taraxasterol inhibits cigarette smoke-induced lung inflammation by inhibiting ROS production and ROS-mediated recruitment of TLR4 into lipid rafts [Citation11]. Taraxasterol protects C6 glial cells from lipopolysaccharide (LPS)-induced neuroinflammation, as proved by the decreased levels of pro-inflammatory cytokines [Citation8]. However, the role of taraxasterol in cerebral I/R injury has not been fully evaluated. Therefore, we aimed to investigate the effect of taraxasterol on cerebral I/R injury and explore the underlying potential mechanism.
Materials and methods
Cell culture
Primary hippocampal neurons were isolated from newborn C57BL6 pregnant female mice (postnatal day 0–2; Experimental Animal Centre of Beijing Institute of Radiation Medicine, Beijing, China) as described previously [Citation12]. The newborn pups were decapitated, the hippocampi were dissected and digested in 0.25% trypsin (Sigma, St. Louis, MO, USA) for 20 min at 37 °C with gentle shaking. The cells were suspended in DMEM (Hyclone, Logan, UT, USA) containing 10% FBS (Gibco Laboratories, Grand Island, NY, USA), 10% horse serum (Gibco), 1% L-glutamine (Gibco), and 1% penicillin-streptomycin (HyClone). Cells (5 × 105 cells/dish) were plated on 15-mm confocal dishes coated with 0.1 mg/ml poly-D-lysine (Sigma). After 2 h, the plating medium was replaced by neurobasal medium containing 2% B27 (Gibco), 1% penicillin/streptomycin, and 0.25% glutamine. Afterwards, half of the medium was changed every four days.
Oxygen-glucose deprivation/reperfusion (OGD/R) model and treatment
For the OGD/R experiments, hippocampal neurons were incubated with glucose-free DMEM medium (Hyclone) in an anaerobic chamber (1% O2, 5% CO2, 94% N2) at 37 °C for 12 h to imitate ischaemic injury. Thereafter, the cells were incubated with a normal medium for 24 h under normoxic conditions (95% air, 5% CO2) to ensure reoxygenation. Hippocampal neurons were cultured with 2.5, 5 or 10 µM taraxasterol (purity ≥ 98%; Chengdu Herbpurify Co., Ltd., Chengdu, China), or 5 μM ML385 (purity ≥ 98%; Sigma) for 2 h, followed by OGD/R stimulation.
Cell viability assay (CCK-8 assay)
Cell Counting Kit-8 (CCK-8; Dojindo, Kumamoto, Japan) was used for the determination of cell viability. Briefly, hippocampal neurons were seeded in 96-well plates at a density of 5 × 103 cells/well and incubated for 24 h at 37 °C for attachment. After different treatments, 10 μL CCK-8 solution was added into the culture and incubated at 37 °C for 1 h. The absorbance was measured using a microplate Reader (Bio-Rad, Hercules, CA, USA) at 450 nm.
Assessment of ROS level
Intracellular ROS level was measured using a probe 2,7-dichlorofluorescein diacetate (DCFH-DA; Nanjing Jiancheng, Nanjing, China). In brief, hippocampal neurons were incubated with 10 μM DCFH-DA for 30 min at 37 °C in the dark. Thereafter, cells were resuspended in 500 μL PBS and subjected into a FACS Calibur flow cytometer (BD Biosciences, Franklin Lakes, NJ, USA) with 488 nm excitation and 521 nm emission for the measurement of fluorescent intensity.
Elisa
Hippocampal neurons were homogenised in 0.1 M phosphate buffer (pH 7.4) and then centrifuged at 3000 × g for 5 min at 4 °C. The supernatants were used to determine the level of MDA using a commercial MDA kit (Nanjing Jiancheng Bioengineering Institute, Nanjing, China) in accordance with the manufacturer’s instructions.
Caspase-3 activity
Caspase-3 activity of hippocampal neurons was measured using a caspase-3 activity assay kit (Nanjing Jiancheng Bioengineering Institute). According to the instruction, cell lysates of hippocampal neurons were incubated with 50 μL Ac-DEVD-pNA for 4 h at 37 °C. The absorbance at 450 nm was measured using a microplate Reader (Bio-Rad).
Western blotting
The cytoplasmic and nuclear extracts were prepared using NE-PER Nuclear and Cytoplasmic Extraction Reagents Kit (Thermo Scientific, USA) according to the manufacturers’ protocol. Protein concentration in the cytoplasmic and nuclear extracts was quantified using the BCA Protein Assay Kit (Pierce, Appleton, WI, USA). Equal amounts of protein samples (30 μg/lane) were electrophoresed by 10% SDS-PAGE and then blotted onto nitrocellulose membranes, which were blocked in 5% bovine serum albumin (BSA) for 1 h. Afterwards, the membranes were incubated with primary antibodies against bax and bcl-2 (Santa Cruz Biotechnology, Santa Cruz, CA, USA), nuclear Nrf2, lamin B1, haem oxygenase (HO-1), NAD(P)H:quinone oxidoreductase 1 (NQO-1), GPx-3 and β-actin (Abcam, Cambridge, MA, USA), followed by incubation with horseradish peroxidase-conjugated secondary antibody (Abcam). Finally, protein bands on the membranes were visualised using the ECL Western blotting detection reagent (GE Healthcare, Braunschweig, Germany) and quantified by ImageJ software (National Institutes of Health, Bethesda, MD, USA).
Statistical analysis
Experiments were performed in triplicate and the results were analysed using GraphPad Prism 5 software (GraphPad, San Diego, CA, USA). All data were expressed as the mean ± standard deviation (SD). The statistical analysis was performed using the one-way analysis of variance (ANOVA) or unpaired two-tailed Student’s t-test. The differences were considered statistically significant if p value < .05.
Results
Taraxasterol ameliorated OGD/R-induced hippocampal neurons injury
We initially examined cell viability of taraxasterol on hippocampal neurons. As shown in , the concentration of 20 μM taraxasterol produced a significant effect, while the concentration of 10 μM taraxasterol showed no significant change. Therefore, 2.5–10 μM of taraxasterol was used in the following experiments. Then, to study the role of taraxasterol in cerebral I/R injury in vitro, hippocampal neurons were subjected to OGD/R induction to induce injury. As illustrated in , cell viability was dramatically decreased after OGD/R induction. However, taraxasterol pre-treatment improved OGD/R-induced injury in a dose-dependent manner.
Figure 1. Protective effect of taraxasterol on OGD/R-induced hippocampal neurons injury. (A) Hippocampal neurons were cultured with 2.5, 5, 10 or 20 µM taraxasterol for 24 h. Cell viability was measured using CCK-8 assay. *p < .05. (B) Hippocampal neurons were cultured with 2.5, 5 or 10 µM taraxasterol for 2 h, followed by OGD/R stimulation. Cell viability was measured using CCK-8 assay. Data are expressed as mean ± SD. n = 6. *p < .05 vs. control hippocampal neurons; #p < .05 vs. OGD/R-induced hippocampal neurons.
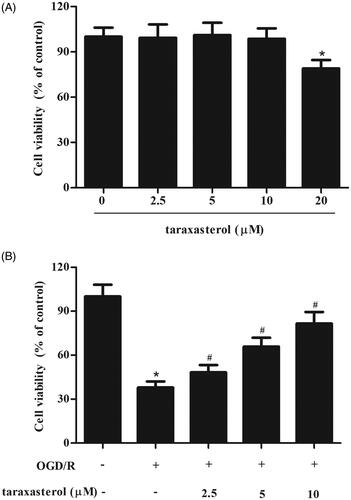
Taraxasterol ameliorated OGD/R-induced oxidative stress in hippocampal neurons
Previous studies have shown that OGD/R could induce oxidative stress. In order to investigate the effect of taraxasterol on OGD/R-induced oxidative stress, the ROS generation was measured. As shown in , as compared with the control group, OGD/R significantly induced ROS production in hippocampal neurons. This effect was reversed by taraxasterol. Similarly, taraxasterol also markedly suppressed OGD/R-induced MDA generation in hippocampal neurons ().
Figure 2. Anti-oxidative effect of taraxasterol on OGD/R-induced hippocampal neurons. Hippocampal neurons were cultured with 2.5, 5 or 10 µM taraxasterol for 2 h and then subjected to OGD/R stimulation. (A) Effect of taraxasterol on ROS generation. (B) Effect of taraxasterol on MDA production. Data are expressed as mean ± SD. n = 5. *p < .05 vs. control hippocampal neurons; #p < .05 vs. OGD/R-induced hippocampal neurons.
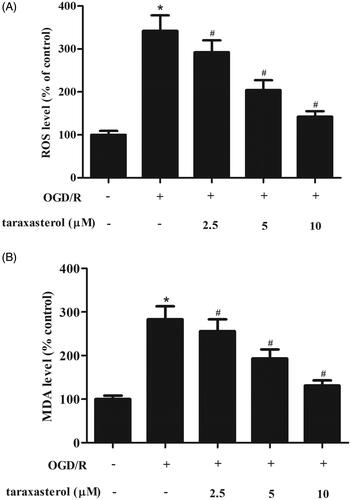
Taraxasterol inhibited OGD/R-induced cell apoptosis in hippocampal neurons
To evaluate the effect of taraxasterol on cell apoptosis of hippocampal neurons, western blot analysis was performed to detect the expressions of bax and bcl-2. The results indicated that the expression of bax was increased, and bcl-2 expression was decreased in OGD/R-induced hippocampal neurons. However, the OGD/R-caused changes of bax and bcl-2 expressions were mitigated by taraxasterol (). The increased caspase-3 activity in OGD/R-induced hippocampal neurons was dose-dependently ameliorated by taraxasterol (.
Figure 3. Anti-apoptotic effect of taraxasterol on OGD/R-induced hippocampal neurons. Hippocampal neurons were incubated for 2 h with the pre-treatment of 2.5, 5 or 10 µM prior to OGD/R stimulation. (A) Effect of taraxasterol on expressions of bax and bcl-2. (B and C) Quantification analysis of bax and bcl-2. (D) Effect of taraxasterol on caspase-3 activity. Data are expressed as mean ± SD. n = 5. *p < .05 vs. control hippocampal neurons; #p < .05 vs. OGD/R-induced hippocampal neurons.
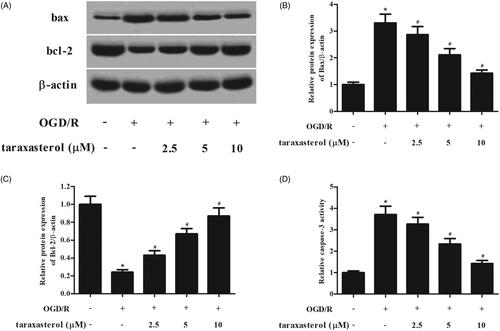
Taraxasterol increased the nuclear accumulation of Nrf2 in hippocampal neurons under OGD/R condition
Nrf2 pathway is an important signalling pathway which is involved in oxidative stress. Therefore, the nuclear level of Nrf2 was determined using western blot. Compared with the control group, a significant increase in nuclear level of Nrf2 was observed in OGD/R-induced hippocampal neurons. Pre-treatment with taraxasterol induced the accumulation of nuclear Nrf2 expression as compared to the OGD/R-induced hippocampal neurons ().
Figure 4. Effect of taraxasterol on Nrf2 signalling in OGD/R-induced hippocampal neurons. Hippocampal neurons were incubated with the pre-treatment of 2.5, 5 or 10 µM for 2 h prior to OGD/R stimulation. (A) The nuclear level of Nrf2 was measured using western blot. (B) Quantification analysis of Nrf2/lamin B1. Data are expressed as mean ± SD. n = 4. *p < .05 vs. control hippocampal neurons; #p < .05 vs. OGD/R-induced hippocampal neurons.
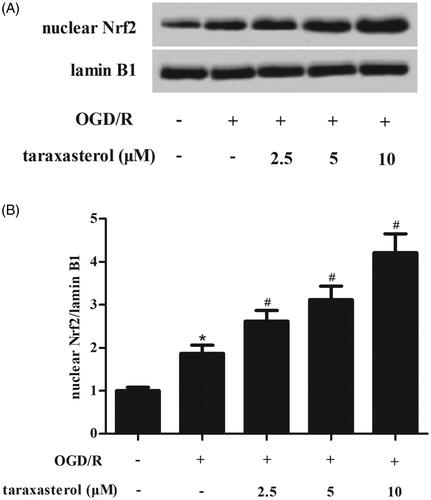
Taraxasterol regulated the downstream pathway protein expression of Nrf2 pathway in hippocampal neurons under OGD/R condition
Next, we attempted to determine whether taraxasterol affected the expressions of downstream proteins including HO-1, NQO-1 and GPx-3. As shown in , western blot analysis demonstrated that OGD/R induced the expressions of HO-1, NQO-1 and GPx-3. However, the increased levels of these proteins were enhanced by taraxasterol.
Figure 5. Effect of taraxasterol on the expressions of Nrf2 downstream proteins in OGD/R-induced hippocampal neurons. Hippocampal neurons were incubated with the pre-treatment of 2.5, 5 or 10 µM for 2 h prior to OGD/R stimulation. (A) The protein expression levels of HO-1, NQO-1 and GPx-3 were determined using western blot. (B–D) Quantification analysis of HO-1, NQO-1 and GPx-3. Data are expressed as mean ± SD. n = 5. *p < .05 vs. control hippocampal neurons; #p < .05 vs. OGD/R-induced hippocampal neurons.
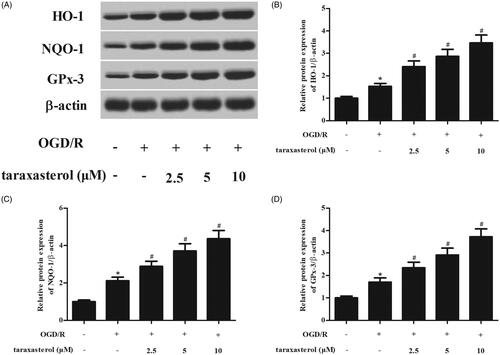
ML385 reversed the effects of taraxasterol in hippocampal neurons under OGD/R condition
In order to further assess the role of Nrf2 pathway in the effect of taraxasterol, we used ML385 to inhibit the Nrf2 pathway. The results demonstrated that ML385 reversed the protective effect of taraxasterol on hippocampal neurons viability (). The taraxasterol-caused decrease in ROS and MDA productions were suppressed by ML385 (). Additionally, a significant increase in caspase-3 activity was observed in ML385-treated hippocampal neurons when compared with taraxasterol-treated hippocampal neurons ().
Figure 6. Effect of ML385 on the roles of taraxasterol in OGD/R-induced hippocampal neurons. Hippocampal neurons were pre-treated with 10 µM taraxasterol and/or 5 μM ML385 for 2 h, followed by OGD/R stimulation. (A) Effect of ML385 on cell viability. (B and C) Effects of taraxasterol on ROS generation and MDA production. (D) Effect of taraxasterol on caspase-3 activity. Data are expressed as mean ± SD. n = 4. *p < .05.
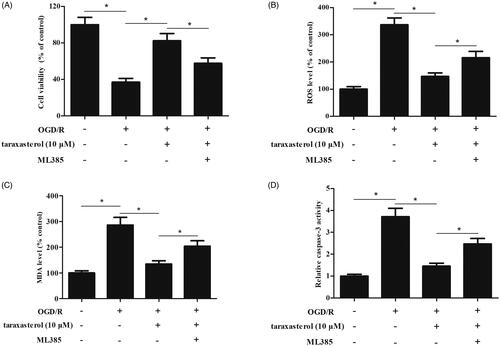
Discussion
In this study, our results showed that taraxasterol significantly inhibited OGD/R-induced oxidative stress and cell apoptosis in hippocampal neurons. The underlying mechanism is associated with its regulatory effect on Nrf2 pathway in hippocampal neurons.
It has been demonstrated that ROS is an important intracellular signalling molecule in I/R injury [Citation13,Citation14]. Its involvement in mediating oxidative damage during IR injury has been extensively documented. In the normal physiologic state, multiple antioxidant enzymes such as SOD, GPx, and catalase (CAT) are important to counteract the effect of ROS [Citation5]. During the process of I/R injury, excessive ROS is produced, and these antioxidant systems are overwhelmed, thus resulting in an imbalance between the production of ROS and the capability of cells to detoxify them, which is called oxidative stress [Citation15]. In addition, there is a solid body of literature supporting the role of ROS in regulating intracellular signalling. ROS has been demonstrated to activate the NF-κB-dependent gene transcription and a number of inflammatory signalling cascades [Citation16]. Thus, ROS mediates the production of various inflammatory cytokines such as interleukin (IL)-1 and tumour necrosis factor (TNF)-α, which initiates inflammatory response. Besides, these inflammatory cytokines may activate cell death signalling and induce cell apoptosis [Citation5]. Collectively, ROS-mediates oxidative stress, inflammatory response, cell apoptosis, thereby resulting in I/R injury. Our results demonstrated that taraxasterol improved OGD/R-caused decrease in cell viability of hippocampal neurons. Taraxasterol suppressed ROS production in OGD/R-induced hippocampal neurons, indicating that taraxasterol attenuated OGD/R-induced oxidative stress. Taraxasterol resulted in a significant decrease in caspase-3 activity and bcl-2 expression, as well as increase in bax expression, which suggested an anti-apoptotic activity of taraxasterol. Taken together, taraxasterol mitigated OGD/R-induced oxidative stress and cell apoptosis in hippocampal neurons.
Nrf2 is a transcription factor that controls the expression of an array of anti-oxidative and detoxification enzymes [Citation17]. The Nrf2 pathway is considered as one of the most important cellular Defence and survival pathway against oxidative stress [Citation18–20]. Under a basal condition, Nrf2 interacts in the cytosol with a protein called kelch-like erythroid cell-derived protein with CNC homology-associated protein 1 (Keap1) [Citation21]. In response to oxidative stimuli, Keap1 loses its binding ability with Nrf2 and escapes from degradation. Therefore, Nrf2 enters the nucleus and binds to the antioxidant response element (ARE), thereby regulates the expressions of downstream genes, such as HO-1, NQO-1 and GPx-3 [Citation22]. Accumulating evidence suggest that Nrf2 signalling is involved in a wide variety of human diseases related to oxidative stress, including cerebral I/R injury [Citation23–25]. Our results showed that taraxasterol induced the Nrf2 nuclear accumulation and expressions of HO-1, NQO-1 and GPx-3 in OGD/R-induced hippocampal neurons. Inhibition of Nrf2 signalling reversed the protective effects of taraxasterol on OGD/R-induced hippocampal neurons injury. Interestingly, a prior study showed that taraxasterol protects mice from ethanol-induced liver injury via regulating Nrf2/HO-1 signalling with increased expressions of Nrf2 and HO-1 [Citation9]. These findings suggest that taraxasterol exerted protective effects on OGD/R-induced hippocampal neurons injury via regulating the Nrf2 signalling.
In conclusion, these findings demonstrated that taraxasterol protected hippocampal neurons from OGD/R-induced oxidative stress and cell apoptosis via regulating the Nrf2 signalling pathway.
Disclosure statement
The authors declare that they have no conflicts of interest to disclose.
References
- Randolph SA. Ischemic stroke. Workplace Health Saf. 2016;64(9):444.
- Sarkar S, Chakraborty D, Bhowmik A, et al. Cerebral ischemic stroke: cellular fate and therapeutic opportunities. Front Biosci. 2019;24:435–450.
- Barow E, Thomalla G. Acute treatment of ischemic stroke: current standards. Nervenarzt. 2019;76:641–649.
- Rodrigo R, Fernandez-Gajardo R, Gutierrez R, et al. Oxidative stress and pathophysiology of ischemic stroke: novel therapeutic opportunities. CNS Neurol Disord Drug Targets. 2013;12(5):698–714.
- Zhang J, Wang X, Vikash V, et al. ROS and ROS-mediated cellular signaling. Oxid Med Cell Longev. 2016;2016:1–4350965.
- Wu MY, Yiang GT, Liao WT, et al. Current mechanistic concepts in ischemia and reperfusion injury. Cell Physiol Biochem. 2018;46(4):1650–1667.
- Piao T, Ma Z, Li X, et al. Taraxasterol inhibits IL-1beta-induced inflammatory response in human osteoarthritic chondrocytes. Eur J Pharmacol. 2015;756:38–42.
- Srivastava P, Mohanti S, Bawankule DU, et al. Effect of Pluchea lanceolata bioactives in LPS-induced neuroinflammation in C6 rat glial cells. Naunyn-Schmiedebergs Arch Pharmacol. 2014;387(2):119–127.
- Xu L, Yu Y, Sang R, et al. Protective effects of taraxasterol against ethanol-induced liver injury by regulating CYP2E1/Nrf2/HO-1 and NF-kappaB signaling pathways in mice. Oxid Med Cell Longev. 2018;2018:8284107–8284133.
- Zhang X, Xiong H, Liu L. Effects of taraxasterol on inflammatory responses in lipopolysaccharide-induced RAW 264.7 macrophages. J Ethnopharmacol. 2012;141(1):206–211.
- Xueshibojie L, Duo Y, Tiejun W. Taraxasterol inhibits cigarette smoke-induced lung inflammation by inhibiting reactive oxygen species-induced TLR4 trafficking to lipid rafts. Eur J Pharmacol. 2016;789:301–307.
- Zeng Y, Shen Y, Hong L, et al. Effects of single and repeated exposure to a 50-Hz 2-mT electromagnetic field on primary cultured hippocampal neurons. Neurosci Bull. 2017;33(3):299–306.
- Francis A, Baynosa R. Ischaemia-reperfusion injury and hyperbaric oxygen pathways: a review of cellular mechanisms. Diving Hyperb Med. 2017;47(2):110–117.
- Inauen W, Suzuki M, Granger DN. Mechanisms of cellular injury: potential sources of oxygen free radicals in ischemia/reperfusion. Microcirc Endothelium Lymphatics. 1989;5(3–5):143–155.
- Weidinger A, Kozlov AV. Biological activities of reactive oxygen and nitrogen species: oxidative stress versus signal transduction. Biomolecules. 2015;5(2):472–484.
- Thannickal VJ, Fanburg BL. Reactive oxygen species in cell signaling. Am J Physiol Lung Cell Mol Physiol. 2000;279(6):L1005–1028.
- Ahmed SM, Luo L, Namani A, et al. Nrf2 signaling pathway: pivotal roles in inflammation. Biochim Biophys Acta Mol Basis Dis. 2017;1863(2):585–597.
- Zhang H, Davies KJA, Forman HJ. Oxidative stress response and Nrf2 signaling in aging. Free Radic Biol Med. 2015;88:314–336.
- Johnson JA, Johnson DA, Kraft AD, et al. The Nrf2-ARE Pathway: an indicator and modulator of oxidative stress in neurodegeneration. Ann NY Acad Sci. 2008;1147(1):61–69.
- Buendia I, Michalska P, Navarro E, et al. Nrf2-ARE pathway: an emerging target against oxidative stress and neuroinflammation in neurodegenerative diseases. Pharmacol Ther. 2016;157:84–104.
- Guo Y, Yu S, Zhang C, et al. Epigenetic regulation of Keap1-Nrf2 signaling. Free Radic Biol Med. 2015;88:337–349.
- Tu W, Wang H, Li S, et al. The anti-inflammatory and anti-oxidant mechanisms of the Keap1/Nrf2/ARE signaling pathway in chronic diseases. Aging Dis. 2019;10(3):637–651.
- Wang P, Liang X, Lu Y, et al. MicroRNA-93 downregulation ameliorates cerebral ischemic injury through the Nrf2/HO-1 defense pathway. Neurochem Res. 2016;41(10):2627–2635.
- Pan H, He M, Liu R, et al. Sulforaphane protects rodent retinas against ischemia-reperfusion injury through the activation of the Nrf2/HO-1 antioxidant pathway. PLoS One. 2014;9(12):e114186.
- Zhang W, Wei R, Zhang L, et al. Sirtuin 6 protects the brain from cerebral ischemia/reperfusion injury through NRF2 activation. Neuroscience. 2017;366:95–140.