Abstract
Schisandrin B (Sch B) and miR-101 family members play critical roles in the pathogenesis of liver fibrosis. However, the relationship between them has not been reported yet. Thus, this study aims to fill this research gap. Results showed that Sch B significantly upregulated the expression of miR-101-5p in HSC-T6 cells. Sch B also increased the expression of miR-101-5p by combined administration of TGF-β1 and Sch B. Using miR-101-5p inhibitor, we demonstrated that Sch B can target miR-101-5p through the TGF-β signalling pathway to regulate the proliferation and activation of HSC-T6 cells. A rat model of carbon tetrachloride-induced liver fibrosis was established, and results indicated that Sch B can attenuate liver fibrosis by upregulating the expression of miR-101-5p. In conclusion, Sch B can directly target miR-101 to suppress liver fibrosis. Sch B or miR-101-5p may be used as a therapeutic approach for the prevention and treatment of liver fibrosis.
Introduction
Liver fibrosis is generally associated with chronic hepatic inflammation and injury. In this process, hepatic stellate cells (HSCs) are activated and secrete excess extracellular matrix (ECM) in the liver, resulting in fibrosis [Citation1]. Several signalling pathways, including TGF-β and PDGF signalling, participate in the activation of HSCs [Citation2]. However, the underlying fine molecular mechanism remains unclear.
MicroRNAs (miRNAs) are small endogenous noncoding RNAs with a length of 19–25 nt that play negative regulatory roles in gene expression at the post-transcriptional gene regulation level [Citation3]. Recent studies have indicated that miRNAs, including miR-29b, miR-378, miR-21, miR-942, and miR-101 [Citation2,Citation4–12], participate in regulating HSC activation, making them ideal targets for hepatic fibrosis treatment. Tu et al. demonstrated that miR-101 family members prevent liver fibrosis by targeting the TGF-β signalling pathway [Citation7]. However, how to regulate miR-101 in suppressing HSC activation and liver fibrosis progression remains unclear to date.
As the main active ingredient of Schisandra chinensis, Schisandrin B (Sch B) exhibits various biological activities, such as anti-tumor, anti-oxidative, anti-inflammatory, and hepatoprotective [Citation13,Citation14]. A recent study has reported that Sch B attenuates liver fibrosis by regulating the Nrf2-ARE and TGF-β/smad signalling pathways [Citation1]. However, the detailed molecular mechanism by which Sch B regulates liver fibrosis needs further elaboration. Thus, this study aims to fill this research gap. In this work, we selectively discovered the relationship between miR-101 and Sch B and found for the first time that Sch B targets miR-101-5p to prevent liver fibrosis by regulating the TGF-β signalling pathway.
Materials and methods
Materials
Sch B was purchased from China Pharmaceutical Biological Products Analysis Institute (Shanghai, China). HSC-T6 cell line was purchased from American Tissue Culture Collection (ATCC) (Rockville, MD, USA). Dulbecco’s Modified Eagle’s Medium (DMEM) and foetal bovine serum (FBS) were obtained from GIBCO Life Technologies (Gaithersburg, MD, USA). miR-101-5p inhibitor and the primer sequences were purchased from Shanghai GenePharma Co., Ltd. (Shanghai, China) and are shown in . α-SMA, Collagen I, TGF-β1, and GAPDH antibodies were purchased from Abcam Company (Cambridge, MA, UK). MTT assay kit and carbon tetrachloride (CCl4) were obtained from Sigma–Aldrich (Saint Louis, USA). Male SD rats (total of 32, 8 for each group, 180–220 g, 6–8 weeks old) were purchased from Hunan SJA Laboratory Animal Co., Ltd. (Hunan, China).
Table 1. The primer sequences used in RT-PCR assay.
Animals experiment design
Male SD rats were acclimatised in a ventilated, temperature-controlled room (25°C ± 2 °C and 12-h light/dark cycle) with free access to water and food. All animal procedures were performed in accordance with the Guide for the Care and Use of Laboratory Animals and approved by Hunan Province, China. Rats were randomly divided into four groups (n = 8 per group): sham control group, model group, 20 mg/kg Sch B group, and 40 mg/kg Sch B group. The model group was treated with CCl4 (50% CCl4/olive oil; 2 ml/kg) thrice a week for 8 weeks to induce liver fibrosis. In the Sch B-treated group, the rats were treated with CCl4 for 4 weeks and then administered with Sch B at a dose of 20 or 40 mg/kg daily for 28 days [Citation1]. At the 8th week, the animals were sacrificed, and part of the liver tissue was fixed. The embedded liver tissue was sectioned after paraffin embedding, and the liver tissue samples were stored at −80 °C for later use.
Histological assays
Liver tissue was stained with haematoxylin/eosin (H&E) and Sirius red, fixed in 10% formalin, paraffin embedded and then sectioned. For H&E staining, the sections were dewaxed and dehydrated by alcohol gradient. Distilled water was transferred to the dyeing solution. After haematoxylin staining, 1% hydrochloric acid was used for separation and washing. The sections were stained with eosin and dehydrated by alcohol gradient. For Sirius red staining, liver tissue was first stained with Celestin blue for 10 min, rinsed with distilled water, and then dyed with Sirius red for 20 min. All sections were imaged under a microscope (Olympus, Tokyo, Japan).
Cell transfection and treatment
HSC-T6 cells were cultured in DMEM with 10% foetal bovine serum and then incubated at 37 °C and 5% CO2. The cells were seeded in 6-well plates and cultured to a cell density of 60–70%. Transfection experiments were carried out using Lipofectamine 2000 in accordance with the manufacturer’s instructions. Then, miR-101-5p was transfected in accordance with the instructions of GenePharma.
MTT assay
The cells were seeded in 96-well plates and then cultured for 24, 48, and 72 h. A 20 μL aliquot of MTT solution (5 mg/mL) was added to each well and then incubated for another 4 h. The culture solution was discarded, and then 150 μL of DMSO was added to the plates. The plates were oscillated for 10 min until the purple crystals completely dissolved. The absorbance of each well was measured at 570 nm by a microplate reader. Three duplicates were prepared for each sample.
Real-time PCR analysis
Total RNA was extracted using the TRIzol reagent (15596026; Invitrogen) in accordance with the manufacturer′s protocol. Total RNA (2 μg) was used to synthesise first-strand cDNA by the GoScript™ Reverse Transcription system (Promega Corporation, Madison, WI, USA). mRNA expression was quantified with SYBR Green Master mix (Qiagen, Hilden, Germany) using the Bio-Rad CFX96 PCR system (Bio-Rad Laboratories, Inc., Hercules, CA, USA). Real-time PCR analysis was conducted under the following conditions: one cycle of 95 °C for 2 min and 40 cycles of 95 °C for 15 s, 60 °C for 15s, and 68 °C for 20 s. GAPDH and U6 were amplified as reference genes for mRNA and miRNA, respectively. The primer sequences used in PCR are shown in . The expression levels were measured in terms of the cycle threshold (Ct) and then normalised to GAPDH or U6 expression using the 2−ΔΔCt method.
Western blot
Protein was lysed with the lysis buffer (50 mmol Tris, 150 mmol NaCl, 1% Triton X-100, 1% sodium deoxycholate, and 0.1% SDS) containing protease inhibitors (Roche Diagnostics, Basel, Switzerland). Protein concentrations were determined using a BSA kit (Tiangen Biotech Co., Ltd., Beijing, China). The samples were separated by 12% SDS-PAGE and then transferred onto nitrocellulose membranes (GE Healthcare Life Sciences, Little Chalfont, UK). After being blocked with 5% non-fat milk for 1.5 h, the membranes were incubated with primary antibodies at 4 °C overnight and then with the secondary antibodies for 2 h at room temperature. Protein expression was imaged by ImageQuant TL version 1.1 (GE Healthcare Life Sciences).
Statistical analysis
All data are presented as mean ± SEM. Differences between groups were determined by a two-tailed Student’s t-test in GraphPad Prism 6.01. Statistical significance was considered at p < .05.
Results
Sch B suppresses HSC activation and upregulates the expression of miR-101-5p
HSC-T6 cells were treated with three concentrations of Sch B (10, 20, and 40 µM) for 24, 48, and 72 h, and cell viability was measured by MTT. Results indicated that Sch B significantly inhibited HSC-T6 cell proliferation at three-time points (). Collagen synthesis and deposition are related to the activation of HSCs. Thus, the mRNA and protein expression levels of TGF-β1, α-SMA, and collagen I significantly decreased after Sch B treatment (). The expression of miR-101-5p was also detected. As shown in , miR-101 was constantly upregulated as the concentration of Sch B was increased, indicating that Sch B can promote the expression level of miR-101-5p.
Figure 1. Sch B suppresses HSC activation and up-regulates the expression of miR-101-5p. (A) Effects of Sch B on HSC-T6 cells proliferation and viability. Cell viability was measured by MTT. (B) Effects of Sch B on the relative mRNA expression levels of miR-101-5p, TGF-β1, α-SMA and collagen I. (C, D) Effects of Sch B on the protein expression levels of TGF-β1, α-SMA, and collagen I. Data are mean ± SEM of three independent experiments; *p < .05, **p < .01.
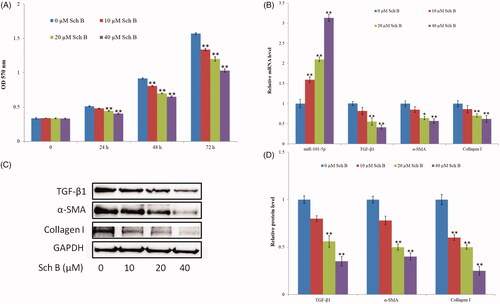
Sch B inhibits TGF-β1-induced HSC activation and increases the expression of miR-101-5p
HSC-T6 cells were treated with TGF-β1 for 24 h after serum starvation, and cell viability was measured by MTT. Results indicated that TGF-β1 (10 ng/mL) significantly promoted HSC-T6 cell proliferation, but this process was significantly inhibited by Sch B treatment (). TGF-β1 (10 ng/mL) significantly increased the mRNA and protein levels of α-SMA and collagen I. However, they significantly decreased after treatment with Sch B, especially at the concentration of 40 µM (). The expression level of miR-101-5p was almost unchanged in the TGF-β1 treatment group, but it greatly increased in the Sch B treatment groups ().
Figure 2. Sch B inhibits TGF-β1-induced HSC activation and increases the expression of miR-101-5p. (A) Effects of Sch B on TGF-β1-induced HSC-T6 cells proliferation and viability. Cell viability was measured by MTT. (B) Effects of Sch B on the relative mRNA expression levels of miR-101-5p, α-SMA and collagen I. (C,D) Effects of Sch B on the protein expression levels of α-SMA and collagen I under TGF-β1-induced HSC-T6 activation. Data are mean ± SEM of three independent experiments; *p < .05, **p < .01.
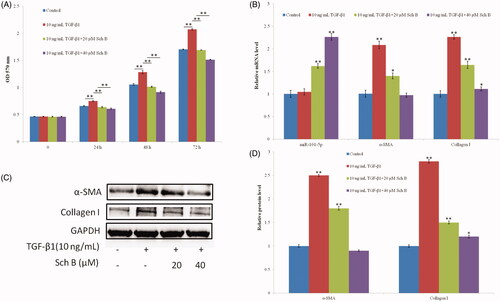
Sch B targets miR-101-5p to suppress HSC activation
Sch B can effectively upregulate the expression of miR-101-5p. Thus, it possibly targets miR-101-5p to suppress the activation of HSC-T6. Compared with inhibitor NC (negative control), administration of miR-101-5p inhibitor greatly increased the proliferation of HSC-T6 cells () and the expression of TGF-β1, α-SMA, and collagen I (). This result indicates that miR-101-5p inhibits HSC-T6 cell proliferation. Sch B can significantly inhibit the activation of HSC-T6, but simultaneous administration of Sch B and miR-101-5p inhibitor can significantly increase HSC-T6 cell proliferation and the expression of TGF-β1, α-SMA, and collagen I (). This result suggests that Sch B targets miR-101-5p to suppress HSC activation.
Figure 3. Sch B targets miR-101-5p to suppress HSC activation. (A) Effects of Sch B and miR-101-5p inhibitor on HSC-T6 cells proliferation and viability, cell viability was measured by MTT. (B) Effects of Sch B and miR-101-5p inhibitor on the relative mRNA expression levels of miR-101-5p, TGF-β1, α-SMA and collagen I. (C, D) Effects of Sch B and miR-101-5p inhibitor on the protein expression levels of TGF-β1, α-SMA, and collagen I. Data are mean ± SEM of three independent experiments; *p < .05, **p < .01.
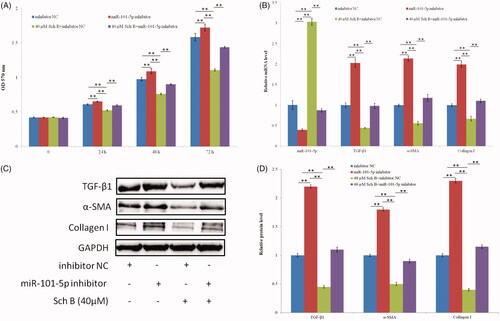
Sch B upregulates miR-101-5p and suppresses CCl4-induced liver fibrosis in rats
The protective effect of Sch B on liver fibrosis induced by CCl4 in rats was evaluated. H&E staining indicated that Sch B treatment can effectively improve the pathological damage induced by CCl4, and Sirius Red staining showed that Sch B treatment can visibly reduce collagen accumulation in the liver. The phenomenon became more obvious as the concentration of Sch B was increased (). Subsequently, the mRNA and protein levels of TGF-β1, α-SMA, and collagen I were also detected. As shown in ), they markedly increased in the CCl4-treated rats but significantly decreased in the Sch B-treated rats. These findings are consistent with previous studies in rats. Furthermore, the expression of miR-101-5p was greatly downregulated in the CCl4-treated rats, but it was gradually increased in the Sch B-treated groups. These results suggest that Sch B can reduce CCl4-induced liver fibrosis in rats, and it is likely caused by Sch B targeting and upregulating miR-101-5p through the TGF-β signalling pathway.
Figure 4. Sch B up-regulates miR-101-5p and ameliorates CCl4-induced liver fibrosis in rats. (A) Liver fibrosis assessed by H&E and Sirius Red staining. (B) The mRNA levels of miR-101-5p, TGF-β1, α-SMA, and collagen I. (C,D) The protein levels of TGF-β1, α-SMA, and collagen I. Data are mean ± SEM (n = 8); *p < .05, **p < .01.
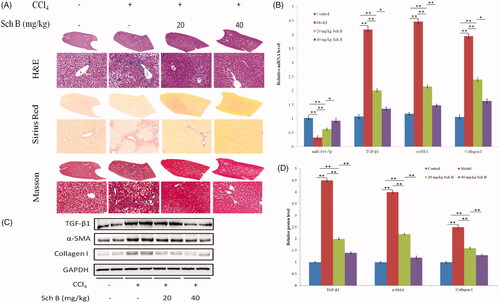
Discussion
In this study, we discovered that miR-101-5p expression was upregulated by the administration of Sch B in in vitro HSC-T6 cells and in vivo CCl4-treated rats. Thus, miR-101-5p as a novel target of Sch B can attenuate liver fibrosis by regulating the TGF-β signalling pathway. This study demonstrated for the first time that Sch B can regulate the transcription level of miR-101-5p.
So far, few reports about the role of Sch B in the regulation of miRNAs are available, but these events are involved in different biological functions. Ji et al. demonstrated that Sch B can reduce LPS-induced sepsis inflammation by miR-17-5p downregulating TLR4 [Citation15]. Sch B also inhibits cell proliferation by regulating the related microRNAs [Citation16,Citation17]. Ba et al. reported that Sch B exerts neuroprotective effect in 6-OHDA-induced Parkinson’s disease by involving the negative regulatory mechanism of miR-34a on Nrf2 pathways [Citation18]. Although Sch B serves multiple biological functions, including anti-inflammatory, anti-oxidative stress, and anti-tumor properties [Citation13,Citation14], the detailed molecular mechanisms need further elaboration. Chen et al. found that Sch B attenuates liver fibrosis by regulating the Nrf2-ARE and TGF-β/smad signalling pathways [Citation1], but the direct molecules that Sch B interacts with remain unknown. MiR-101 suppresses the proliferation of cancer cells, including hepatocellular carcinoma (HCC), by inducing apoptosis [Citation19]. Recent studies have found that miR-101 plays an important role in tissue fibrosis. The expression of miR-101 is downregulated in cardiac fibrosis [Citation20]. Further study demonstrated that miR-101 can suppress fibrotic programming and fibrosis following trans-aortic constriction in vivo through the TGF-β signalling pathway [Citation21]. Tu et al. found that miR-101 can attenuate CCl4-induced liver fibrosis in rats by inhibiting the TGF-β signalling pathway [Citation7]. Subsequently, researchers also found that miR-101 suppresses liver fibrosis by downregulating the PI3K/Akt/mTOR signalling pathway [Citation22]. MiR-101 can also repress hypoxia-induced fibrosis of bladder after BOO (Bladder outlet obstruction) and attenuate pulmonary fibrosis by inhibiting fibroblast proliferation and activation [Citation23,Citation24]. However, the relationship between Sch B and miR-101 in attenuating liver fibrosis is poorly understood. In the present study, we show that Sch B can upregulate the expression level of miR-101-5p and that miR-101-5p is a novel target of Sch B in HSC-T6 cells.
In conclusion, liver fibrosis, a chronic liver injury disease, can gradually develop into liver cirrhosis and HCC [Citation25,Citation26]. Therefore, revealing the pathogenesis of liver fibrosis and finding measures to treat it are highly important. Sch B, a new molecule, can reduce CCl4-induced liver fibrosis in rats [Citation1], and miR-101 can target the TGF-β signalling pathway to suppress liver fibrosis [Citation7]. The present study is the first to demonstrate that miR-101-5p is a direct target of Sch B and the interaction between miR-101-5p and Sch B can significantly reduce HSC-T6 activation and liver fibrosis in rats. The results provide detailed information for using Sch B to treat liver fibrosis.
Author contributions
Yinyan Jiang made substantial contributions to the conception of the present study. Cuiqiong Wang performed the experiments and wrote the manuscript; Chao Xu and Xinling Fu made substantial contributions to the design of the present study and interpreted the data. All authors read and approved the final version of the manuscript for publication
Disclosure statement
There is no conflict of interest in this manuscript.
Additional information
Funding
References
- Chen Q, Zhang H, Cao Y, et al. Schisandrin B attenuates CCl4-induced liver fibrosis in rats by regulation of Nrf2-ARE and TGF-beta/Smad signaling pathways. DDDT. 2017;11:2179–2191.
- Caviglia JM, Yan J, Jang MK, et al. MicroRNA-21 and Dicer are dispensable for hepatic stellate cell activation and the development of liver fibrosis. Hepatology. 2018;67(6):2414–2429.
- Su Q, Kumar V, Sud N, et al. MicroRNAs in the pathogenesis and treatment of progressive liver injury in NAFLD and liver fibrosis. Adv Drug Deliv Rev. 2018;129:54–63.
- Roderburg C, Urban GW, Bettermann K, et al. Micro-RNA profiling reveals a role for miR-29 in human and murine liver fibrosis. Hepatology. 2011;53(1):209–218.
- Hyun J, Wang S, Kim J, et al. MicroRNA-378 limits activation of hepatic stellate cells and liver fibrosis by suppressing Gli3 expression. Nat Commun. 2016;7(1):10993.
- Tao L, Xue D, Shen D, et al. MicroRNA-942 mediates hepatic stellate cell activation by regulating BAMBI expression in human liver fibrosis. Arch Toxicol. 2018;92(9):2935–2946.
- Tu X, Zhang H, Zhang J, et al. MicroRNA-101 suppresses liver fibrosis by targeting the TGFbeta signalling pathway. J Pathol. 2014;234(1):46–59.
- Zou Y, Li S, Li Z, et al. MiR-146a attenuates liver fibrosis by inhibiting transforming growth factor-β1 mediated epithelial-mesenchymal transition in hepatocytes. Cell Signal. 2019;58:1–8.
- You K, Li SY, Gong J, et al. MicroRNA-125b promotes hepatic stellate cell activation and liver fibrosis by activating RhoA signaling. Mol Ther Nucleic Acids. 2018;12:57–66.
- Yu F, Lu Z, Cai J, et al. MALAT1 functions as a competing endogenous RNA to mediate Rac1 expression by sequestering miR-101b in liver fibrosis. Cell Cycle. 2015;14(24):3885–3896.
- Huang YH, Kuo HC, Yang YL, et al. MicroRNA-29a is a key regulon that regulates BRD4 and mitigates liver fibrosis in mice by inhibiting hepatic stellate cell activation. Int J Med Sci. 2019;16(2):212–220.
- Xing Hea YS, Lei N, Fan X, et al. MicroRNA-351 promotes schistosomiasis-induced hepatic fibrosis by targeting the vitamin D receptor. Proc Natl Acad Sci USA. 2018;115:180–185.
- Chun JN, Cho M, So I, et al. The protective effects of Schisandra chinensis fruit extract and its lignans against cardiovascular disease: a review of the molecular mechanisms. Fitoterapia. 2014;97:224–233.
- He JL, Zhou ZW, Yin JJ, et al. Schisandra chinensis regulates drug metabolizing enzymes and drug transporters via activation of Nrf2-mediated signaling pathway. Drug Des Devel Ther. 2015;9:127–146.
- Ji ZR, Xue WL, Zhang L. Schisandrin B attenuates inflammation in LPS-induced sepsis through miR-17-5p downregulating TLR4, inflammation. 2019;42:731–739.
- Jiang Y, Zhang Q, Bao J, et al. Schisandrin B inhibits the proliferation and invasion of glioma cells by regulating the HOTAIR-micoRNA-125a-mTOR pathway. Neuroreport. 2017;28(2):93–100.
- Zhang XY, Zhang LX, Guo YL, et al. Schisandrin B inhibits the proliferation of airway smooth muscle cells via microRNA-135a suppressing the expression of transient receptor potential channel 1. Cell Biol Int. 2016;40(7):742–749.
- Ba Q, Cui C, Wen L, et al. Schisandrin B shows neuroprotective effect in 6-OHDA-induced Parkinson’s disease via inhibiting the negative modulation of miR-34a on Nrf2 pathway. Biomed Pharmacother. 2015;75:165–172.
- Su H, Yang JR, Xu T, et al. MicroRNA-101, down-regulated in hepatocellular carcinoma, promotes apoptosis and suppresses tumorigenicity. Cancer Res. 2009;69(3):1135–1142.
- Zhenwei Pan XS, Shan H, Wang N, et al. MicroRNA-101 inhibited postinfarct cardiac fibrosis and improved left ventricular compliance via FBJ osteosarcoma oncogene/transforming growth factor-β1 pathway. Circulation. 2012;126:840–850.
- Zhou Y, Shiok TC, Richards AM, et al. MicroRNA-101a suppresses fibrotic programming in isolated cardiac fibroblasts and in vivo fibrosis following trans-aortic constriction. J Mol Cell Cardiol. 2018;121:266–276.
- Lei Y, Wang QL, Shen L, et al. MicroRNA-101 suppresses liver fibrosis by downregulating PI3K/Akt/mTOR signaling pathway. Clin Res Hepatol Gastroenterol, 2019:43(5):575–584.
- Wang N, Duan L, Ding J, et al. MicroRNA-101 protects bladder of BOO from hypoxia-induced fibrosis by attenuating TGF-β-smad2/3 signaling. IUBMB Life. 2019;71(2):235–243.
- Zhao X, Wang K, Liao Y, et al. MicroRNA-101a inhibits cardiac fibrosis induced by hypoxia via targeting TGFbetaRI on cardiac fibroblasts. Cell Physiol Biochem. 2015;35(1):213–226.
- Zhang DY, Friedman SL. Fibrosis-dependent mechanisms of hepatocarcinogenesis. Hepatology. 2012;56(2):769–775.
- Schuppan D, Kim YO. Evolving therapies for liver fibrosis. J Clin Invest. 2013;123(5):1887–1901.