Abstract
Vulvar intraepithelial neoplasia (VIN) is associated with human papillomavirus (HPV) infection. Curcumin is a natural bioactive compound with antineoplastic properties. The use of nanoparticles containing curcumin could allow a better performance of this compound in therapies. So, VIN biopsies were collected and HPV DNA detection was performed by PCR, positive samples were genotyped by Restriction Fragment Length Polymorphism (RFLP) and HPV-16 variants were determined by sequencing. HPV-16 positive vulva carcinoma cells (A431) were transduced with E-P and E-350G HPV-16 E6 variants. The viability of the transduced cells treated with nanoemulsions was determined by MTT assay. Besides, apoptosis was evaluated by enzymatic activity of Caspase-3/7. The cell viability assay showed that both the empty nanoemulsion (NE-V) and the nanoemulsion of curcumin (NE-CUR) had little effect on cell viability as compared to control cells. Additionally, we observed that cells irradiated in the presence of NE-CUR presented 90% of cell death. The apoptosis assay further revealed a significant increase in the activity of caspases 3 and 7 in A431 cells expressing both HPV-16 E6 variants after treatment with NE-CUR. Finally, we submitted the HPV transduced A431 cells to organotypic cultures and observed that the combination of treatments affected tissue architecture with evident signals of tissue damage. We concluded that nanoemulsions attain good biocompatibility, since no cytotoxicity was observed and NE-CUR associated with photoactivation showed promising results, leading to death only in cells subjected to irradiation. This drug delivery system associated with photodynamic therapy may become promising in the treatment of vulva lesions.
Introduction
Vulvar intraepithelial neoplasia (VIN) is characterised by a squamous intraepithelial lesions highly associated with the development of invasive carcinoma. Two types of VIN can be considered precursors of vulvar cancer: the usual type (uVIN), normally characterised by low-grade lesions associated with human papillomavirus (HPV) infection, and the differentiated type (dVIN), characterised by high-grade lesions associated with chronic lichen, squamous cell hyperplasia or untreated lichen sclerosus [Citation1].
High-risk HPVs, mostly HPV-16, have a main role in the development of HPV related VIN [Citation2]. Worldwide HPV-16 variants are differently distributed and share over 98% of nucleotide identity [Citation3]. Despite the high similarity, some specific HPV-16 variants were shown to have an increased oncogenic potential in vitro and to be epidemiologically more associated to the risk for cervical carcinoma [Citation4]. Particularly, the European variant enclosing a non-synonym alteration, T350G E6, has been associated with a higher risk for high-grade cervical lesions, although data is still controversial [Citation4–6].
VIN treatment options include antiviral therapies such as cidofovir and intralesional or intramuscular interferon-α, as well as topical imiquimod application in the case of exophytic warts [Citation7]. Additionally, vulvar lesions can also be removed surgically, by simple or radical vulvectomy. More advanced lesions can be treated with chemotherapy and radiotherapy and laser therapy may also be used in the treatment of VIN [Citation8]. Unfortunately, adverse effects such as pain, irritation, superficial ulceration and hypersensitivity have been reported [Citation9]. Since there is currently no effective therapeutic measure established, surgical removal, including both excision and ablation, is still recommended in more severe VIN cases. However, this intervention is still associated with high morbidity and high recurrence rates [Citation8].
Several studies indicate that natural compounds, like curcumin, have anti-HPV and anti-cancer activity [Citation10,Citation11]. However, the clinical use of curcumin is still limited because of the low solubility of this drug in water and its low bioavailability when administered orally due to this compound rapid degradation and excretion [Citation12]. To counteract these problems, several drug delivery systems, such as nanostructured materials, have been designed to improve the solubility and bioavailability of hydrophobic compounds, especially for those that present fast clearance rates [Citation13–15]. Nanoemulsions are a class of stable emulsions formed by a monolayer of phospholipids composed of a surfactant that has an important role in nanoemulsions stabilisation [Citation16]. These emulsions have been used in some studies associated to photoactive compounds fort topical or systemic application.
Curcumin effects are potentiated when associated with visible light and can be used as a photosensitizer agent in photodynamic therapy (PDT), [Citation17–20]. PDT is a therapeutic approach that can be applied in the treatment of several types of tumours [Citation21–23]. In fact, PDT in combination with natural drugs can represent an alternative non-invasive treatment modality for VIN with the advantage of minimal healthy tissue harm and low side effects [Citation24]. In the present study we aimed to evaluate the effects of PDT and curcumin nanoemulsion in vulva cell lines grown in monolayer and organotypic cell cultures. We also investigated the effect of HPV-16 genes in cells response upon treatment with nanoemulsions with curcumin and PDT.
Materials and methods
Clinical samples
The present prospective, observational study was conducted at Sao Paulo State University in Sao Jose do Rio Preto, SP, Brazil and was approved by the Research Ethics Committee of the Sao Paulo State University in Sao Jose do Rio Preto and of the Clinical Hospital of the Ribeirão Preto Medical School (931.918). Patients enrolled attended the University of São Paulo study centre at Ribeirão Preto Medical School from August 2014 till November 2015. Informed written consent was obtained from all participants. VIN was diagnosed by vulvar biopsy.
Viral DNA amplification and genotyping
DNA was extracted using the QIAamp DNA Micro kit (Qiagen Inc., Hilden, Germany). HPV genotyping was performed by Polymerase Chain Reaction (PCR) using generic primers PGMY09/11 [25]. PCR was performed using 2.5 μL Buffer (10X), 4.0 mM of MgCl2, 0.32 mM of μL PGMY09/11 primers, 0.2 mM of dNTP, 2.5 U of High Fidelity Enzyme Mix (Fermentas), 80 ng of DNA and Mili-Q to a final volume of 25 μL. Reaction was then submitted to one cycle of 95 °C for 13 min, followed by 40 cycles of 95 °C for 1 min, 55 °C for 1 min and 72 °C for 1 min. A final extension cycle was performed at 72 °C for 5 min. Positive samples were genotyped by RFLP (Restriction Fragments Length Polymorphism [26]. All PCR reactions included a negative control consisting of the reaction mixture, but without DNA.
HPV-16 E6 variant characterisation
The E6 gene from HPV-16 positive samples was amplified with specific primers to genotype molecular variants (Forward: 5′-TAAAACTAAGGGCGTAACCG-3′ – Reverse: 5′-TCTATTTCATCCTCCTCCTCTG-3′). PCR was performed using 5 μL Buffer (10X) 1.5 mM μL MgCl2 (25 mM), 1 mM of complementary primers for the E6 region (20 mM), 0.5 mM 6 μL dNTP (2 mM), 1.5 U of High Fidelity Enzyme Mix (Fermentas), 500 ng μL DNA and milli-Q water q.s.p 50 μL final reaction volume. The cycling conditions were: 1 cycle of 95 °C for 5 min followed by 40 cycles of 95 °C for 1 min, 50 °C for 1 min and 72 °C for 2 min and one final extension cycle of 72 °C for 8 min. Following purification with the TOPO XL Gel Purification Kit (Invitrogen, California, USA), PCR products were cloned using the pCR XL-TOPO Vector (Invitrogen, California, USA). Four clones from each specimen were purified using the GeneJET™ Plasmid Miniprep Kit (Fermentas Life Sciences, Ontario, Canada). Sequencing reactions were performed in an ABI 3130XL sequencer (Applied Biosystems, California, USA) using the BigDye® Terminator v3.1 Cycle Sequencing Kit (Applied Biosystems, California, USA). Electropherograms obtained in the sequencing of E6 region were analysed by the quality analysis programme Eletropherogram Quality Analysis available online at http://www.biomol.unb.br/phph (EWING et al. 1998). Comparisons to identity the sequences were performed using the BLAST programme (Basic Local Alignment Search Tool) available at http://www.ncbi.nlm.nih.gov/BLAST (ALTSCHUL et al. 1990). Subsequently, variants detected in genotyping were identified by comparison of the sequences with reference sequences of HPV-16 variants (E-P – GenBank number K02718), HPV-42 (GenBank n° GQ472847.1), HPV-59 (GenBank n° EU918767) and HPV-61 (GenBank n° U31793.1). Alignment and sequence correction were performed using the Bio Edit 7.0.5.3 programme [27].
Synthesis of curcumin nanoemulsions: preparation and characterisation
Nanoemulsions were synthesised by the “Photobiology and Photomedicine Group” at the Centre of Nanotechnology and Tissue Engineering of São Paulo University, in Ribeirão Preto, Brazil. Curcumin Nanoemulsions (NE-Cur) were prepared by curcumin solubilisation in oil phase followed by aqueous phase homogenisation with a hydrophilic emulsifier. The same procedure was used for empty nanoemulsion (NE), except the addition of curcumin. Further details on the formulation and preparation of nanoemulsions are in process of patent application and should be protected in accordance with the regulation of the Brazilian patent agency (Patent: Privilege of Innovation, Registration number: PI07063210).
Particle size, polydispersity index (IPd) and Zeta potential were evaluated using the Zetasizer Nano system ZS (Malvern-UK), employing a 4 mW He-Ne laser that operates in 633 nm wavelength, allowing non-invasive measurements by backscatter optics (NIBS). This methodology allows evaluating particle size in the range of 2 nm to 3 μm. Readings were performed according to the manufacturer instructions and analysis was performed using Zetasizer software version 6.01.
The stability of the liposomal formulations was studied by evaluating the size of the vesicles every 15 days, during a period of 90 days. Analysis was performed in triplicates at 25 °C. Samples were stored at room temperature protected from light.
Generation of cell lines transducing HPV-16 E6 variants
The E6 gene from HPV-16 variants E-P and E-350G was cloned into the retroviral expression vector pLXSN (Clontech Laboratories). Briefly, the E6 gene was amplified and cloned into the pLXSN vector (Genebank n° M28248.1). Plasmidial DNA was extracted using the GeneJET™ Plasmid Miniprep Kit (Fermentas Life Sciences, Canada) following the manufacturer’s instructions, and direct sequencing was performed to confirm genotype the nucleotide sequence. Retroviral vectors were transfected by electroporation in Bosc-23 ecotropic cells, and supernatants were used to infect Am-12 amphotropic cells [Citation28]. Infected cells were selected with 500 μg/mL G418 (Life Technologies) for 14 days. A431 cells (Genebank n° HTB-117), derived from a HPV-negative vulvar carcinoma (Sigma Aldrich), were cultured in DMEM medium supplemented with 10% foetal bovine serum and 100 U/mL penicillin, and further infected by retroviruses produced by Am-12 infected cells. Selection was performed with medium containing G418 (Life Technologies) at 600 μg/ml for another 14 days. HaCat control cells, (spontaneously immortalised keratinocyte cell line) also were cultured in DMEM medium supplemented with 10% foetal bovine serum and 100 U/mL penicillin.
RNA extraction and qPCR
Total RNA was isolated using TRIzol (Life Technologies, Carlsbad, USA) according to the manufacturer’s instructions. qPCR was used to assess HPV-16 E6 expression in A431 cells transduced with the different variants of HPV-16. Gene-specific primers for qPCR were described elsewhere [Citation29]. Quantitative real-time PCR was performed using an ABI prism 7300 sequencer detector system and SybrGreen PCR Core Reagent (Applied Biosystems, Foster City, CA, USA) following the manufacturer’s protocol. In brief, the reaction mixture (20 µL final volume) included 25 ng of cDNA, gene-specific forward and reverse primers for each gene, and 10 ml of 2x Quantitative Sybr Green PCR Master Mix (Applied Biosystems, Foster City, USA). Relative quantification was given by CT values, conducted in triplicate reactions and glyceraldehyde-3-phosphate dehydrogenase (GAPDH) amplification was used as an endogenous control. The relative expression of each gene was calculated using the formula: R¼(E target)ΔCt target (control – sample)/(E endogenous) ΔCt endogenous (control – sample), as previously described [Citation30]. The cut-off for analysis of gene expression was ≥2 increased or decreased expression.
Cellular uptake of curcumin-loaded nanoemulsions
A431 cells transducing E6 from the different HPV-16 variants and human immortalised keratinocytes HaCaT cells were plated in monolayers and incubated with 80 μM of NE-Cur for 24 h. After replacing the media, cells were analysed by fluorescence microscopy (Zeiss, Göschwitz, Germany) at 480 nm excitation spectrum, blue laser, 415 ± 15 nm, FITC/GFP.
MTT assay
2 × 104 cells were plated in 96-well plates and incubated at 37 °C in 5% CO2. After 24 h, cells were treated with 20, 40, 80, 100 or 120 μM of free curcumin and equivalent doses of NE-Cur or NE for three hours after which media was replaced. MTT assays were performed after 24 h following media replacement with no nanoparticles. Experiments were performed in triplicates in at least three independent assays and readings were conducted at 570 nm. Obtained values were normalised with the control (cells submitted to the same conditions without the addition of the formulation under study) expressed in percentage of viability calculated according to the following relation: Viable cells = (O.D. Sample/O.D Control) x 100%.
Phototoxicity assay
Cells were seeded at 1 × 104 density in 96-well plates 24 h before to treatment, and followed incubation with 80 µM of NE and NE-Cur for three hours. Nanoemulsions were removed and cells were carefully washed with PBS, and 150 μL/well of DMEM medium without foetal calf serum (FCS) or phenol red. Cultures were then subjected to laser irradiation at 50 J/cm2 for 4 min. The irradiation source comprised a higher potency LED apparatus, model Vet Light of DMC Enterprise (São Carlos, SP, Brazil) operating at 447 (± 10) nm, with 420 mW of power at 23 mm and 2.52 W of total power, for 209 W/cm2 of irradiancy and 80 J/cm2 of fluency, set at 6.4 s/application.
After irradiation, the medium was replaced by culture medium without phenol or FCS and 24 h later cells were once more photoactivated. After 24 h the MTT assay was performed and cell viability was evaluated.
Caspase-3/7 enzymatic activity measurement
Caspase-3/7 activity was evaluated using the Caspase-Glo® 3/7 Assay Kit (Promega, USA), following the manufacturer’s instructions. Briefly, 2.5 × 104 cells were seeded in 96-well plates. After 24 h, cells were incubated with 80 μM NE-Cur or only culture medium. After 3 h, cells were washed with PBS. Fresh media without FCS or Phenol red was added before cultures were subjected to laser irradiation at 50 J/cm2 for 4 min. Media was then replaced by fresh media without FCS or Phenol red. Readings were performed in a luminometer and normalised relative to to control cells. Percentage of caspase activity was calculated according to the following relation: Activity of caspase: (UL sample/UL control) X 100%.
Organotypic cultures of A431 cell lines
Organotypic cultures were performed as described elsewhere [Citation31]. Briefly, 2 × 105 A431 cells expressing E6 from the different HPV-16 variants were seeded in a dermal equivalent constituted of a collagen type 1 matrix and J2 fibroblasts (feeders) in a proportion of 105 J2 cells per 0.75 mL collagen matrix. After 24 h dermal equivalent with cell attached was transferred to a steel grid on a 60 mm diameter Petri dish. Organotypic cultures were maintained at 37 °C and 5% CO2 with 5 mL of raft medium per plate to allow cell stratification in a medium/air interface for 11 days before treatment. Organotypic cultures were divided into three treatment groups: 80 μM NE-Cur + PDT; Control 1 (DMEM + PDT) and Control 2 (DMEM). At 3 h after treatment, cultures were washed with PBS and media was replaced. With an exception of Control 2, all cultures were irradiated for 4 min as described before. Cultures were then incubated again with NE-Cur for 3 h, and a new irradiation dose was performed. After 24 h cultures were fixed in 10% buffered formalin to be processed for histology. 4 μm sections were stained with haematoxylin/eosin and slides were evaluated in an Olympus BX-60 microscope coupled to a digital camera. Image analyser system – Image-Pro-Plus©Media Cybernetics was used for photodocumentation.
Statistical analysis
Statistical analyzes were performed using the GraphPad Prism 5 software and the statistical significance of the differences between the results obtained was determined by the One-way ANOVA analysis of variance followed by the Tukey t-test test for multiple comparisons (* p < .001). All analyzes were done in triplicates.
Results
HPV detection and genotyping in vulvar intraephitelial neoplasia samples (VIN)
Whereas in cervical carcinoma, HPV DNA can be found in up to 99.7% of samples [Citation32], HPV is present in 65–75% of VIN. High-risk HPVs, such as HPV-16 and HPV-18 are responsible for over 50% of vulvar cancers [Citation33]. We aimed to investigate the prevalence of HPV and HPV-16 variants in VIN 24 samples collected in Ribeirão Preto Medical School of the University of Sao Paulo, Brazil, between August 2014 and November 2015. In this collection, 25% (6) of the patients were aged between 18 and 28 years, 20.8% (5) were between 29 and 39 years and 54.2% (13) of patients had over 40 years in the collection date. The average age of patients analysed was 41 years, being 18 years the minimum age and 78 years the maximum age. Patients presenting HPV + HIV coinfection (17%) developed more serious lesions such as cervical intraepithelial neoplasia (CIN). HPV DNA was detected in 50% (12/24) of the samples; HPV-16 was the most prevalent type, detected in six samples (50%), followed by HPV-6 (25%). The other three positive samples were each infected with HPVs 42, 59 or 61 (). HPV-16 variants analysis showed that most HPV-16 samples contained the E-P variant, while the E-350G variant was detected in one sample ().
Figure 1. HPV detection in VIN biopsies. Positivity and genotyping of human papillomavirus (HPV) detected in vulvar neoplasia samples (VIN).
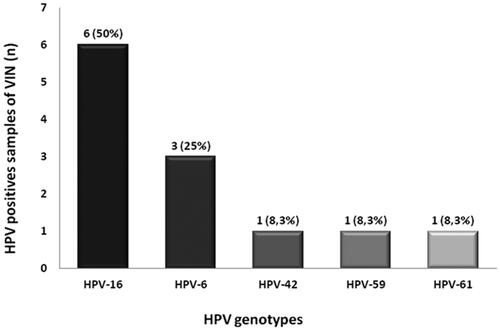
Table 1. HPV-16 E6 genetic variability.
A431 cell line infection with retroviral vectors
Once the European and 350 G HPV-16 variants were detected in the analysed samples, retroviral vectors expressing E6 were then constructed to infect the A431 HPV negative vulva carcinoma line. Confirmation of the transcriptional expression of E6 in A431/E6E and A431/E6350G was obtained by qPCR (Supplementary Figure 1).
Particle size, polydispersity and zeta potential analysis of nanoparticles containing curcumin
Particle size is known to affect drug release kinetics from nanoparticles. It also affects the amount of nanoparticles that can be absorbed by the cells and how it will interact with the target tissue based on the zeta potential. We performed a physical- chemical characterisation of empty nanoemulsions (NE) and nanoemulsions containing curcumin (NE-Cur). The average particle size was 180 nm in NE and 195 nm in NE-Cur seems to be in the range of biological active nanomaterial. In addition, polydispersity index value of the formulations was less than 0.2, an indicative of a homogeneous particle size distribution. Zeta potential, which measures the effective electric charge on nanoparticle surface, confirmed that theses nanoemulsions presented negative surface charge of -46.3 in NE and -53.7 in NE-Cur (), which allows the perfect interaction with the target tissue. We also confirmed that nanoemulsions were stable over a period of 90 days while stored at 25 °C (Supplementary Figure 2). These results indicate that NE-cur are suitable for further applications.
Table 2. Characteristics of nanoparticles used in this study.
Cellular uptake and cytotoxicity of curcumin-loaded nanoemulsions
Curcumin absorption spectrum consists in only one characteristic band in the visible light region (400–420 nm). Based on the fact that curcumin presents natural fluorescence in the range 450–700 nm with excitation fixed at 440 nm, we evaluated the internalisation of nanoemulsions by human immortalised keratinocytes (HaCat) and A431 cells using fluorescence microscopy. After 3 h of incubation with 80 μM of NE-Cur, cells displayed cytoplasmic green fluorescence (). We also observed that A431 cells expressing E6 from the different HPV-16 variants efficiently uptake NE-Cur nanoemulsions in levels comparable to non-transduced parental cells. HPV-16 E6 expression from both E-P and E-350G variants in A431 transduced cells was confirmed by qPCR (Supplementary Figure 1).
Figure 2. Cellular uptake and cytotoxicity of curcumin-loaded nanoemulsions. (a) Fluorescence microscopy images of cells A431, HaCat, A431 transduced with HPV-16 E6350G and E6E. NE-Cur was incubated in a concentration of 80 μM and pictures were taken after 3 h incubation period. Magnification 40X. (b) MTT assay to evaluate empty nanoemulsion (NE), curcumin nanoemulsion (NE-Cur) and free curcumin (Free-Cur) toxicity. A431/E6350G, A431/E6E, A431/empty and HaCat cells were treated for 24 h with 20 μM, 40 μM, 80 ΜM, 100 μM and 120 μM of preparations.
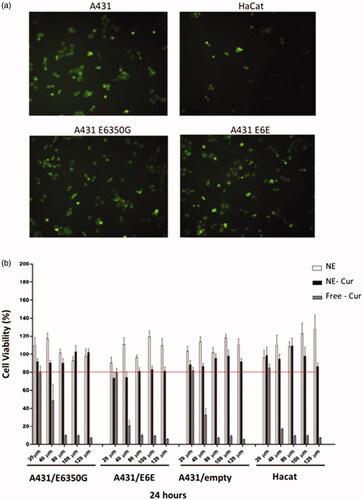
We next evaluated the effect of NE, NE-Cur and free curcumin upon cell viability of HaCat, A431 transduced with retroviral particles expressing E6 from two different HPV-16 variants or the PLXSN empty vector. We observed that 24 h following treatment NE did not interfere with cell viability, even in the highest concentration used (120 μM) (). Viability of all cell lines tested dropped slightly when NE-cur was used even though no dose-dependent effect was observed. In contrast, treatment with non-encapsulated curcumin had a strong negative effect upon cell viability; in concentrations higher than 80 μM, free curcumin was extremely toxic and cell viability was as low as 10% for all cell types tested. Taken together, our results indicate that NE-Cur are well tolerated and are much less toxic for cells as compared to free curcumin.
Phototoxicity of curcumin-containing nanoemulsions
Curcumin effects can be potentiated by exposure to 430 nm light, and it has been shown that when used as a photosensitizer for PDT a strong production of cytotoxic intracellular ROS associated to increased cell death is observed. Therefore, we evaluate the cytotoxic potential of NE-Cur in A431 HPV-16 E6 transduced cells and in HaCat. Our results show that PDT of NE treated cells did not affect cell viability, even after two irradiation doses of 50 J/cm2 for 4 min, performed 3 h and 24 h after treatment (). On the other hand, NE-Cur treatment had a strong effect upon cell viability when associated with PDT: for all the cell lines analysed viability dropped over 85% after two cycles of photoirradiation, independent of the cells analysed (). These results indicate that photoactivation strongly potentiates the effect of curcumin.
Figure 3. Phototoxicity assay in A431 and HaCaT cell lines. (a) Viability (%) of cells treated for 3 h and 24 h with NE and NE-cur (80 μM) followed by light irradiation (430 nm). Results are representative of 3 independent experiments (*p < .001). (b) Enzymatic activity of caspase -3 and -7 of cell lines treated with NE-cur followed by photoactivation shown as a percentage in relation to the control samples (100%). Results are representative of 3 independent experiments (*p < .001).
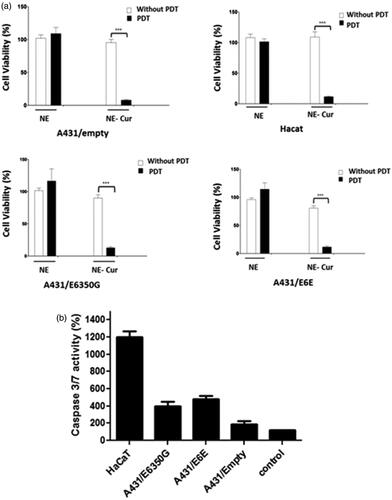
We next evaluated if PDT Ne-Cur activation influences apoptosis. Our results show that HPV-16 E6 transduced cells treated with NE-Cur and photoactivated after 3 h following treatment attain increased in caspase3/7 activity () as compared to non PDT exposed cells, in contrast to A431 cells infected with the empty vector. Interestingly, HaCat cell line displayed the highest caspase activation upon combined treatment of PDT and NE-Cur (). Altogether, these results indicate that PDT in the presence of NE-Cur can ultimately affect cell viability irrespective the cell type. Besides, the observations made in cells expressing HPV-16 E6 suggest that cell death can be triggered at different rates depending on the cell background or HPV status.
Effect of nanoemulsions-containing curcumin in organotypic cultures
Photodynamic therapy treatment was approved for use of mainly superficial skin lesions [Citation34]. Cells grown in organotypic cultures are able to form stratified cell layers, closely resembling epithelial tissues. Therefore, we sought to evaluate the effect of NE-Cur in organotypic cultures of A431 cells transduced with E6E350G and pLXSN control vector. Photoactivation was performed 3 h and 24 h after treatment. Controls in which cultures were only photoactivated (C1) or did not receive any type of treatment (C2) were also included. Our results show that, upon photoactivation, tissues treated with NE-Cur display a fragmented morphology, a strong indication of tissue damage. C1 was slightly affected by treatment, while C2 displayed no further alterations (). In summary, our data show that nanoemulsions-containing curcumin, when combined with PDT, are able to partially destroy epithelial tissues.
Figure 4. Organotypic raft cultures of vulvar carcinoma cells (A431). A431 expressing pLXSN (empty vector) and HPV-16 variant E6350G treated with 80 μM of nanoemulsion containing curcumin (NE-Cur) and doubly photoactivated. C1: treated only with photoactivation; C2: without any treatment. Haematoxylin and eosin staining. Arrowheads indicate areas in which it is possible to observe epithelial fragmentation. Scale bar 50 μm.
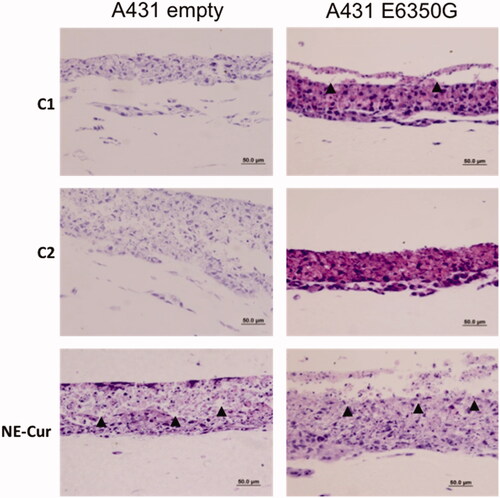
Discussion
We evaluated the prevalence of HPV and HPV-16 variants in VIN. Our results showed that biopsy of 12 out of the 24 patients analysed were positive for HPV. This result is in line with previous results indicating that HPV has a prevalence of 50% in VIN [Citation33]. The average age sampled in the cases of uVIN and dVIN was 38.4 and 44.9 years, respectively. In comparison to the work of Nieuwenhof et al. [Citation35], in which was observed a median age of 47.8 years for uVIN group and 67 years for dVIN group, our results showed that in our study population dVIN appeared in much younger women. The reason behind this result is unknown and should be further investigated. We also found HPV-16 in 45.5% of our samples. Previous studies also identified HPV-16 as the most prevalent type in VIN. However, in contrast to our results, the prevalence of this particular type was on average 30% higher [Citation36,Citation37]. Besides HPV-16, other genotypes like 6, 42, 59 and 61 were detected. These types are not common in cases of VIN and this may reflect differences in geographical distribution of genotypes. We also identified HPV-16 variants in our study. Six European variants were identified and among them one from the genotype E6-350G. This genotype is frequently found in cervical cancer patients and is associated with increased chance for malignant progression [Citation38].
Compared to traditional chemotherapy and radiotherapy, PDT offers a minimally invasive treatment with very low systemic toxicity [Citation34,Citation39]. Curcumin potential as a photosensitizer agent is being explored for several applications including carcinoma cells and mouth cells [Citation40,Citation41]. Besides, curcumin has been used to treat many types of disease due to its anti-inflammatory, antimicrobial and anticancer properties [Citation42]. However, clinical applications for curcumin are limited due to low aqueous solubility and bioavailability, as well as, rapid metabolism and systemic elimination [Citation12]. Considering this, the use of curcumin encapsulated in nanoparticles brings new therapeutic options offering increased biocompatibility and less cellular toxicity [Citation43]. Our results show that NE-Cur are stable at room temperature, have similar size irrespective of their content and have a good polydispersity index (close to 0.2). Distribution values lower than 0.25 are known to increase nanoemulsion stability [Citation44]. This particular formulation, with an oil-covered surface, also helps to maintain particles well separated and zeta potential have little effect on their stability [Citation45,Citation46]. Our cytotoxicity assay showed that, in comparison with free-curcumin, nanoemulsion containing compound are well tolerated with viability reaching 80% in comparison to approximately 15% using the non-encapsulated drug. This data is supported by other studies showing that anti-cancer drugs, such as doxorubicin, also present decreased cytotoxicity when encapsulated in polymer nanoparticle systems [Citation47].
Intracellular uptake assay revealed that curcumin is efficiently internalised into the cells and HPV E6 expression did not affect drug uptake. Likewise, breast carcinoma cell lines and murine melanoma efficiently internalises curcumin when it is associated with nanoliposomes or when encapsulated in micelle systems [Citation48,Citation49]. Our phototoxicity assay also revealed that the photosensitising properties of curcumin could be used to potentiate the effect of the drug. After photoactivation, cells treated with encapsulated curcumin experienced a significant drop in viability. This result was also obtained by studies evaluating the effect of curcumin photoactivation in different carcinoma cell lines [Citation50,Citation51]. We also evaluated if curcumin activation by light could induce apoptosis. Interestingly, our results revealed that HPV-16 E6 expressing cells seemed to be more affected by the treatment than the cells treated only with empty nanoparticles. On the other hand, the immortalised HaCat cells showed a strong caspase-3/7 activation. It could indicate that HPV-16 expressing cells and immortalised keratinocytes could be more prone to die than cells not expressing E6. Further investigations could help to understand such differences, especially because curcumin is able to induce apoptosis in malignant cells by mechanisms that are dependent and independent on mitochondria [Citation52]. Considering the fact that A431 cell line is able to form stratified epithelia when grown in in organotypic cultures we tested the effect of combining NE-cur with phosphodynamic therapy. Our results showed that the combination of treatment strongly affected tissue architecture with evident signals of tissue damage, especially in the upper layers of the epithelia. Interestingly, organotypic cultures of cervical cancer cell line Caski treated with curcumin displayed a dramatic reduction in cell growth and epithelial thickness [Citation53]. Our work brings a new perspective of treatment for VIN, especially those associated to HPV infection. In the future it is possible that nanoemulsions containing curcumin could be combined to PDT to offer more specific treatment options, with much less side effects.
Supplemental Material
Download MS Word (104.8 KB)Supplemental Material
Download MS Word (243 KB)Disclosure statement
No potential conflict of interest was reported by the author(s).
Correction Statement
This article has been republished with minor changes. These changes do not impact the academic content of the article.
Additional information
Funding
References
- Preti M, Scurry J, Marchitelli CE, et al. Vulvar intraepithelial neoplasia, best practice and research. Clin Obstet Gynaecol. 2014;28:1051–1062.
- Vuyst HD, Clifford GM, Nascimento MC, et al. Prevalence and type distribution of human papillomavirus in carcinoma and intraepithelial neoplasia of the vulva, vagina and anus: a meta-analysis. Int J Cancer. 2009;124:1626–1636.
- de Villiers EM, Fauquet C, Broker TR, et al. Classification of papillomaviruses. Virology. 2004;324(1):17–27.
- Londesborough P, Ho L, Terry G, et al. Human papillomavirus genotype as a predictor of persistence and development of high-grade lesions in women with minor cervical abnormalities. Int J Cancer. 1996;69(5):364–368.
- Grodzki M, Besson G, Clavel C, et al. Increased risk for cervical disease progression of French women infected with the human papillomavirus type 16 E6-350G variant, Cancer epidemiology, biomarkers and prevention: a publication of the American Association for Cancer Research. Am Soc Prevent Oncol. 2006;15:820–822.
- Villa LL, Sichero L, Rahal P, et al. Molecular variants of human papillomavirus types 16 and 18 preferentially associated with cervical neoplasia. J General Virol. 2000;81(12):2959–2968.
- Rivera A, Tyring SK. Therapy of cutaneous human Papillomavirus infections. Dermatol Ther. 2004;17(6):441–448.
- Nelson EL, Stockdale CK. Vulvar and vaginal HPV disease. Obstet Gyn Clin N Am. 2013;40(2):359–376.
- Lacey CJ. Therapy for genital human papillomavirus-related disease. J Clin Virol. 2005;32(1):82–90. Suppl
- Singh M, Tyagi S, Bhui K, et al. Regulation of cell growth through cell cycle arrest and apoptosis in HPV 16 positive human cervical cancer cells by tea polyphenols. Invest New Drugs. 2010;28(3):216–224.
- Garcia-Zepeda SP, Garcia-Villa E, Diaz-Chavez J, et al. Resveratrol induces cell death in cervical cancer cells through apoptosis and autophagy. Eur J Cancer Prev. 2013;22:577–584.
- Anand P, Kunnumakkara AB, Newman RA, et al. Bioavailability of curcumin: problems and promises. Mol Pharmaceutics. 2007;4(6):807–818.
- Ma Z, Haddadi A, Molavi O, et al. Micelles of poly(ethylene oxide)-b-poly(epsilon-caprolactone) as vehicles for the solubilization, stabilization, and controlled delivery of curcumin. J Biomed Mater Res. 2008;86:300–310.
- Ganta S, Amiji M. Coadministration of paclitaxel and curcumin in nanoemulsion formulations to overcome multidrug resistance in tumor cells. Mol Pharmaceutics. 2009;6(3):928–939.
- Shaikh J, Ankola DD, Beniwal V, et al. Nanoparticle encapsulation improves oral bioavailability of curcumin by at least 9-fold when compared to curcumin administered with piperine as absorption enhancer. Eur J Pharm Sci. 2009;37(3–4):223–230.
- Gupta A, Eral HB, Hatton TA, et al. Nanoemulsions: formation, properties and applications. Soft Matter. 2016;12(11):2826–2841.
- Primo FL, de Paula LB, de Siqueira-Moura MP, et al. Photobiostimulation on wound healing treatment by ClAlPc-nanoemulsion from a multiple-wavelength portable light source on a 3D-human stem cell dermal equivalent. CMC. 2012;19(30):5157–5163.
- Franchi LP, Amantino CF, Melo MT, et al. In vitro effects of photodynamic therapy induced by chloroaluminum phthalocyanine nanoemulsion. Photodiagnosis Photodynamic Ther. 2016;16:100–105.
- de Jesus PD, Saeki SI, Tedesco AC. An ex vivo study of photobiostimulation in the treatment of skin pathologies. J Biophoton. 2016;9(11–12):1189–1198.
- Pellosi DS, De Jesus P, Tedesco AC. Spotlight on the delivery of photosensitizers: different approaches for photodynamic-based therapies. Expert Opin Drug Del. 2017;14(12):1395–1406.
- Buggiani G, Troiano M, Rossi R, et al. Photodynamic therapy: off-label and alternative use in dermatological practice. Photodiagnosis Photodyn Ther. 2008;5(2):134–138.
- MacCormack MA. Photodynamic therapy in dermatology: an update on applications and outcomes. Semin Cutan Med Surg. 2008;27(1):52–62.
- Agostinis P, Berg K, Cengel KA, et al. Photodynamic therapy of cancer: an update. CA Cancer J Clin. 2011;61:250–281.
- Lieder A, Khan MK, Lippert BM. Photodynamic therapy for recurrent respiratory papillomatosis. Cochrane Database Syst Rev. 2014;5(6):CD009810.
- Gravitt PE, Peyton CL, Alessi TQ, et al. Improved amplification of genital human papillomaviruses. J. Clin. Microbiol. 2000;38(1):357–361.
- Bernard HU, Chan SY, Manos MN, et al. Identification and assessment of known and novel human papillomaviruses by Polymerase Chain Reaction amplification, restriction fragment lenght polymorphisms, nucleotide sequence and phylogenetic algorithms. J. Infect. Dis. 1994;170:1077–1085.
- Hall TA. Bioedit: A User-Friendly Biological Sequence Alignment Editor and Analysis Program for Windows 95/98/NT. Nucleic Acids Symposium Series. 1999;41:95–98.
- Boccardo E, Manzini Baldi CV, Carvalho AF, et al. Expression of human papillomavirus type 16 E7 oncoprotein alters keratinocytes expression profile in response to tumor necrosis factor-alpha. Carcinogenesis. 2010;31(3):521–531.
- Calmon MF, Sichero L, Boccardo E, et al. HPV16 E6 regulates annexin 1 (ANXA1) protein expression in cervical carcinoma cell lines. Virology. 2016;496:35–41.
- Pfaffl MW. A new mathematical model for relative quantification in real-time RT-PCR. Nucleic Acids Res. 2001;29(9):e45–45.
- Boccardo E, Noya F, Broker TR, et al. HPV-18 confers resistance to TNF-alpha in organotypic cultures of human keratinocytes. Virology. 2004;328(2):233–243.
- Walboomers JM, Jacobs MV, Manos MM, et al. Human papillomavirus is a necessary cause of invasive cervical cancer worldwide. J Pathol. 1999;189(1):12–19.
- Insinga RP, Liaw KL, Johnson LG, et al. A systematic review of the prevalence and attribution of human papillomavirus types among cervical, vaginal, and vulvar precancers and cancers in the United States, Cancer epidemiology, biomarkers and prevention: a publication of the American Association for Cancer Research, cosponsored by the. Am Soc Prevent Oncol. 2008;17:1611–1622.
- Wan MT, Lin JY. Current evidence and applications of photodynamic therapy in dermatology. Clin Cosmet Investig Dermatol. 2014;7:145–163.
- van de Nieuwenhof HP, Massuger LF, van der Avoort IA, et al. Vulvar squamous cell carcinoma development after diagnosis of VIN increases with age. Eur J Cancer. 2009;45(5):851–856.
- de Sanjose S, Alemany L, Ordi J, et al. Worldwide human papillomavirus genotype attribution in over 2000 cases of intraepithelial and invasive lesions of the vulva. Eur J Cancer. 2013;49:3450–3461.
- Smith JS, Backes DM, Hoots BE, Kurman RJ, et al. Human papillomavirus type-distribution in vulvar and vaginal cancers and their associated precursors. Obstet Gynecol. 2009;113:8.
- Rapose A. Human papillomavirus and genital cancer. Indian J Dermatol Venereol Leprol. 2009;75(3):236–243.
- Deda DK, Budu A, Cruz LN, et al. Strategies for development of antimalarials based on encapsulated porphyrin derivatives. Mini Rev Med Chem. 2015;14(13):1055–1071.
- Banerjee S, Prasad P, Hussain A, et al. Remarkable photocytotoxicity of curcumin in HeLa cells in visible light and arresting its degradation on oxovanadium(IV) complex formation. Chem Commun. 2012;48(62):7702–7704.
- Araujo NC, Fontana CR, Gerbi ME, et al. Overall-mouth disinfection by photodynamic therapy using curcumin. Photomed Laser Sur. 2012;30:96–101.
- Feng YJ, Blackburn DC, Liang D, et al. Phylogenomics reveals rapid, simultaneous diversification of three major clades of Gondwanan frogs at the Cretaceous-Paleogene boundary. Proc Natl Acad Sci USA. 2017;114(29):E5864–E5870.
- Jiang B, Li C, Dag O, et al. Mesoporous metallic rhodium nanoparticles. Nat Commun. 2017;8(1):15581.
- Yilmaz E, Borchert HH. Design of a phytosphingosine-containing, positively-charged nanoemulsion as a colloidal carrier system for dermal application of ceramides. Euro J Pharm Biopharm. 2005;60(1):91–98.
- Park HJ. Kong M. Stability investigation of hyaluronic acid based nanoemulsion and its potential as transdermal carrier. Carbohydr Polym. 2011;83:8.
- Rachmawati H, Budiputra DK, Mauludin R. Curcumin nanoemulsion for transdermal application: formulation and evaluation. Drug Dev Ind Pharm. 2015;41(4):560–566.
- Lv Y, Yang B, Jiang T, et al. Folate-conjugated amphiphilic block copolymers for targeted and efficient delivery of doxorubicin. Colloids Surf B Biointerfaces. 2014;115:253–259.
- Patra S, Roy E, Madhuri R, et al. The next generation cell-penetrating peptide and carbon dot conjugated nano-liposome for transdermal delivery of curcumin. Biomater Sci. 2016;4(3):418–429.
- Kumari P, Swami MO, Nadipalli SK, et al. Curcumin delivery by Poly(Lactide)-Based Co-Polymeric micelles: an in vitro anticancer study. Pharm Res. 2016;33(4):826–841.
- Ahn JC, Kang JW, Shin JI, et al. Combination treatment with photodynamic therapy and curcumin induces mitochondria-dependent apoptosis in AMC-HN3 cells. Int J Oncol. 2012;41(6):2184–2190.
- Yan D, Geusz ME, Jamasbi RJ. Properties of lewis lung carcinoma cells surviving curcumin toxicity. J Cancer. 2012;3:32–41.
- Bush JA, Cheung KJ, Jr., Li G. Curcumin induces apoptosis in human melanoma cells through a Fas receptor/caspase-8 pathway independent of p53. Exp Cell Res. 2001;271(2):305–314.
- Maher DM, Bell MC, O’Donnell EA, et al. Curcumin suppresses human papillomavirus oncoproteins, restores p53, Rb, and PTPN13 proteins and inhibits benzo[a]pyrene-induced upregulation of HPV E7. Mol Carcinog. 2011;50(1):47–57.