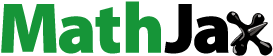
Abstract
Increasing number of literatures highlighted lncRNA maternally expressed gene 3 (MEG3) as an emerging target for hypoxic-ischaemic brain damage (HIBD). This study attempted to assess the role of MEG3 in a cell model of HIBD. Expression of MEG3 in PC12 cells was suppressed by siRNA-mediated transfection, after which the cells were subjected to hypoxia. Cell viability, apoptosis, migration and the expression of related proteins were assessed. Furthermore, the downstream gene of MEG3 and its downstream signalling pathways were explored. We found that, down-regulation of MEG3 prevented hypoxic injury in PC12 cells, as hypoxia induced viability loss, apoptosis and migration repression were attenuated by transfection with MEG3 siRNA. Meanwhile, MEG3 acted as a miR-21 sponge. The neuroprotective functions of MEG3 silence were flattened when miR-21 was suppressed. Moreover, the deactivation of PI3K/AKT pathway and the activation of NF-κB pathway induced by hypoxia were attenuated by MEG3 silence. As expected, the effects of MEG3 silence on these two signalling were via miR-21. In conclusion, the neuroprotective effects of MEG3 silence on PC12 cells injured by hypoxia were observed in this study. Mechanistically, the neuroprotective effects of MEG3 silence on PC12 cells were via sponging miR-21 and thus regulating PI3K/AKT and NF-κB pathways.
MEG3 is highly expressed in PC12 cells following hypoxic injury;
Silence of MEG3 prevents hypoxia-induced cell damage in PC12 cells;
MEG3 acts as a miR-21 sponge;
MEG3 sponges miR-21 to regulate PI3K/AKT and NF-κB pathways.
HIGHLIGHTS
Introduction
The human brain requires a certain concentration of oxygen to maintain normal physiological function. Deprivation of enough oxygen in the brain may lead to the hypoxic-ischaemic brain damage (HIBD). It is a catastrophic disease as its high mortality rate as well as neurological and intellectual impairment in the surviving infants. HIBD is the main reason for neonatal death, chronic nervous system damage and brain retardation. Recently, hypothermia therapy has been recommended for use as an efficiency strategy for treating HIBD [Citation1]. However, it cannot recover brain damage occurred during oxygen deprivation [Citation2]. Thus, novel therapies for preventing brain damage are required to be developed.
Long non-coding RNAs (lncRNAs) are defined as an important group of transcripts that are longer than 200 nt and have no protein-coding capabilities. In recent years, multiple studies have indicated lncRNAs as vital regulators in a variety of diseases associated with neurological, autoimmune, and cardiovascular conditions [Citation3–5]. Moreover, substantial evidence suggests that lncRNAs can regulate cellular growth, death, or other phenotypes [Citation6]. One important mechanism of lncRNAs’ function is owing to theirs binding effects with microRNAs (miRNAs), which are able to regulate gene expression post-transcriptionally [Citation7]. Maternally expressed gene 3 (MEG3), one of the most important cancer-associated lncRNAs, also known as GTL2, was initially identified on chromosome 12 of the distal mouse. MEG3 is localised on human chromosome 14q32 and is approximately 1.6 kb in length, producing alternative spliced transcripts. Similar to its mouse homologues, the expression and regulation of MEG3 is crucial for embryonic development and growth. Increasing number of literatures highlight MEG3 as an emerging target for HIBD, as high expression level of MEG3 has been found in various kind of brain injury model, like rat middle cerebral artery occlusion (MCAO) [Citation8], oxygen-glucose deprivation and reoxygenation (OGD/R)-injured N2a cells [Citation9], and OGD-injured HT22 cells [Citation10]. Moreover, silence of MEG3 exerted protective functions during HIBD, and MEG3 down-regulation mediated by the specific shRNA has been considered as a new strategy for this disease [Citation11].
The mechanism for MEG3’s function in brain damage has been studied accordingly. It is believed that silence of MEG3 protects neurons against various stimulations associated with hypoxic or ischaemic conditions through regulating miRNAs, like miR-129-5p [Citation12], miR-21 [Citation9], and miR-181b [Citation10]. Among these miRNAs, miR-21 has been widely known as a neuroprotective factor during ischaemic-induced brain damage [Citation13,Citation14]. However, studies are still required to see whether MEG3 silence exerted neuroprotective function via miR-21 in other experimental models of HIBD. Besides this, the downstream signalling of miR-21 is required to be revealed to provide a further explanation for MEG3’s function. Thereby, this study investigated the function of MEG3 and miR-21 in a cell model of HIBD made by stimulating PC12 cells with hypoxia. Furthermore, the involvement of MEG3 and miR-21 in the activation of PI3K/AKT and NF-κB pathways was explored.
Materials and methods
Cells
PC12 cells are rat adrenal medulla pheochromocytoma differentiated cell lines, with the general characteristics of neuroendocrine cells, widely used in neurophysiological and neuropharmacological studies. PC12 cells (ATCC, Manassas, VA) were routinely cultured at a density of 1 × 104 cells/mL in RPMI-1640 medium (ATCC). 10% horse serum (Gibco, Grand Island, NY) and 5% foetal bovine serum (Gibco) were added to make complete growth medium. The cells were maintained at 37 °C in a humidified incubator with 5% CO2. To make hypoxic injury, cells were incubated in a hypoxic condition (3% O2) for 24 h in complete growth medium [Citation15].
Transfection
siRNA specific for MEG3 (si-MEG3) and its non-targeting control (si-NC) as a negative control for si-MEG3 were obtained from Genechem Co., LTD. (Shanghai, China). MiR-21 mimic, miR-21 inhibitor and the negative controls (NC mimic and NC inhibitor) were from GenePharma (Shanghai, China). Transfection was done by using lipofectamine RNAimax (Invitrogen, Carlsbad, CA) for 48 h, according to the manufacturer’s instructions. The final dosage of mimic, inhibitor, negative controls and vectors was 50 nM, 200 nM, 100 nM and 2 μg/mL, respectively.
qRT-PCR
The extracted RNA from transfected cells using Trizol reagent (Invitrogen) was subjected to cDNA synthesis by BeyoRT™ II First Strand cDNA Synthesis Kit (Beyotime, Shanghai, China) or Taqman MicroRNA Reverse Transcription Kit (Applied Biosystems, Foster City, CA). PCR procedure was carried out by using BeyoFast™ SYBR Green qPCR Mix (Beyotime) or Taqman Universal Master Mix II (Applied Biosystems), according to the manufacturer’s instructions. Relative MEG3 and miR-21 expression was normalised to glyceraldehyde 3-phosphate dehydrogenase (GAPDH) and U6 respectively.
Primer sequences of MEG3, miR-21, and internal reference (GAPDH and U6), as follows:
MEG3 (forward): 5′-CTGCCCATCTACACCTCACG-3′;
MEG3 (reverse): 5′-CTCTCCGCCGTCTGCGCTAGGGGCT-3.
GAPDH (forward): 5′-TGCACCACCAACTGCTTAGC-3′;
GAPDH (reverse): 5′-GGCATGCACTGTGGTCATGAG-3′.
MiR-21 (forward): 5′-GCGGCGGTAGCTTATCAGACTG-3′;
MiR-21 (reverse): 5′-ATCCAGTGCAGGGTCCGAGG-3′;
U6 (forward): 5′-CTCGCTTCGGCAGCACA-3′;
U6 (reverse): 5′-AACGCTTCACGAATTTGCGT-3′.
Cell viability
The transfected cells in 24-well plates (2 × 105 cells/well) were suffered from hypoxia for 24 h. The viability of cells was tested by trypan blue exclusion, according to this article [Citation16]. Specific steps were as follows: firstly, the gained cell suspension was centrifuged at 100 A ∼ g for 5 min, and the supernatant of tubes was discarded. Then, precipitated cells were resuspended with 1 ml serum-free complete medium. Next, 0.4% trypan blue was added into above cell suspension to get a mixture, and which was placed for 4 min at room temperature. Subsequently, the trypan blue/cell mixture was drop onto a haemacytometer and which was placed on the stage of a binocular microscope and focus on the cells. Finally, counting the unstained (viable) and stained (nonviable) cells separately in the haemacytometer. To obtain the total number of viable cells per mL of aliquot, multiply the total number of viable cells by 2 (the dilution factor for trypan blue). To obtain the total number of cells per ml of aliquot, add up the total number of viable and nonviable cells and multiply by 2.
Calculate the percentage of viable cells as follows:
Apoptosis assay
The transfected cells in 6-well plates (5 × 105 cells/well) were suffered from hypoxia for 24 h. The apoptosis of PC12 cells was detected by using the Annexin V-FITC/PI Apoptosis Detection kit (Becton-Dickinson, San Jose, CA). After PC12 cells were treated, cells were collected and suspended with 500 μL binding buffer containing 5 μL Annexin V-FITC and 5 μL PI. Then, they were hatched for 30 min without light. The FACS can (Beckman Coulter, Fullerton, CA, USA) was used to compute the number of apoptotic cells (Annexin V-FITC-positive and PI-negative) and FlowJo software (Tree Star, Ashland, OR, USA) was used to analyse data.
Migration assay
The transfected cells were suspended in non-serum culture medium (200 ml) and placed in the upper side of a 24-well transwell system (Costar-Corning, New York). The lower side was filled with the complete growth medium (600 ml). Following 24 h of incubation at hypoxic condition at 37 °C, the cells mixed with methanol in the lower side was stained by crystal violet (Beyotime, Shanghai, China) at room temperature for 30 min. Finally, the utilisation of optical microscope (Leica Microsystems) was to count the stained cells.
Luciferase reporter assay
MEG3-wt for expressing wide-type of MEG3 containing the predicted miR-21 binding site was constructed by amplification of MEG3 sequences and insertion into a reporter pmirGlO Dual-luciferase miRNA Target Expression Vector (Promega, Madison, WI). MEG3-mt for expressing MEG3 containing the mutated binding site of miR-21 was constructed and used as a negative control. The reporter plasmids were transfected into cell together with miR-21 mimic or NC mimic. Dual-Luciferase Reporter Assay System (Promega) was then used for testing the luciferase activity.
Western blot
Cellular proteins were extracted by using cell lysis buffer (Beyotime). The extracted cells were quantified via the BCA™ Protein Assay Kit (Pierce, Appleton, WI, USA), according to manufacturers’ instruction. Then, all proteins separated by sodium dodecyl sulfate-polyacrylamide gel electrophoresis (SDS-PAGE) were transferred onto the Polyvinylidene Difluoride (PVDF, Millipore, MA, USA) membranes. Next, the membranes were blocked with 5% BSA for 1 h. Proteins were carried out by using primary antibodies specific for CyclinD1 (orb77046), p53 (orb178524), p21 (orb38089), Bcl-2 (orb378569), Bax (orb378566), pro-caspase-3 (orb13278), cleaved-caspase-3 (orb310032), PTEN (orb39110), PI3K (orb395443), p-PI3K (orb106105), AKT (orb29949), p-AKT (orb344403), IκBα (orb13487), p-IκBα (orb338946), p65 (orb344389), p-p65 (orb106211), and β-actin (orb378579, all from Biorbyt, San Francisco, CA). Following by probing with secondary antibodies, target bands were developed by BeyoECL Plus (Beyotime). The intensity of bands was measured by Image Lab™ Software (Bio-Rad, Hercules, CA).
Statistic
Data presented as mean ± SD from three independent experiments. SPSS 19.0 software (Chicago, IL) was utilised for statistical analysis. Comparison between groups was done by using Student t-test or ANOVA followed by Duncan post-hoc. p-value of <.05 was considered as significance.
Results
Silence of MEG3 prevents hypoxic injury
First of all, PC12 cells were suffered from hypoxia for 24 h to make an experimental model of HIBD. Data in showed that, hypoxic condition significantly declined PC12 cells viability, enhanced apoptotic rate and repressed migration rate as compared to normoxic control (all p < .05). This phenomenon was coupled with the down-regulated expression of CyclinD1, Bcl-2 and the up-regulated expression of p53, p21, Bax, and cleaved caspase-3 in hypoxic cells (all p < .05, ). Those data confirmed the cell damage evoked by hypoxia in PC12 cells.
Figure 1. PC12 cells are injured by hypoxia. PC12 cells were incubated in a hypoxic condition (3% O2) for 24 h to mimic hypoxic injury model. The cells in normoxic condition served as control (CTRL). (A) Cell viability, (B,C) expression of proteins associated with cell growth, (D) apoptotic rate, (E,F) expression of proteins associated with apoptosis, and (G) migration rate of PC12 cells were detected by trypan blue exclusion, western blot, apoptosis assay and migration assay. n = 3. *p < .05 represents a comparison with CTRL groups.
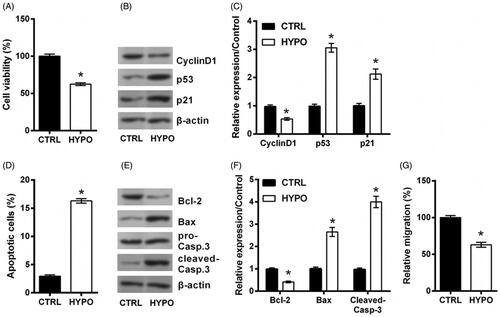
Increasing number of literatures highlighted MEG3 as an emerging target for HIBD. So, the qRT-PCR was conducted to measure the expression of MEG3 following hypoxic incubation. displayed a significant up-regulation of MEG3 in hypoxic cells (p < .05), indicating MEG3 as a sensitive lncRNA in response to hypoxia injury. To investigate the effect of MEG3 on the proliferation, apoptosis and migration of hypoxic PC12 cells, we performed a detection of effects of MEG3 silence on PC12 cells injured by hypoxia were then studied. To this end, MEG3 expression was suppressed by siRNA-mediated silencing. qRT-PCR data in showed a significant down-regulation of MEG3 expression in si-MEG3 transfected cells (p < .05), indicating MEG3 was silenced successfully. As compared to si-NC transfection, si-MEG3 transfection significantly enhanced cell proliferation, reduced apoptosis, and promoted migration in hypoxic cells, including increased viability (p < .05, ) and CyclinD1 expression, and decreased p53 and p21 expression (all p < .05, ), reduced apoptotic rate (p < .05, ) and Bax and Cleaved-Casp-3 expression, and increased Bcl-2 expression (all p < .05, ), and up-regulated migration rate (p < .05, ). Above experimental results revealed that the damage of cells evoked by hypoxia was attenuated by transfecting si-MEG3.
Figure 2. Silence of MEG3 prevents hypoxic injury. (A) The expression of MEG3 in PC12 cells following hypoxia and its expression in cells incubated at normoxic condition served as control (CTRL) was tested by qRT-PCR. (B) The expression of MEG3 in PC12 cells following transfection with MEG3 siRNA (si-MEG3) or its non-targeting control (si-NC)and in cells transfected with nothing served as control (CTRL) was tested by qRT-PCR. Following transfection of si-MEG3 or si-NC, cells were incubated in hypoxia for 24 h. The cells without transfection in normoxic condition served as control (CTRL). (C) Cell viability, (D,E) expression of proteins associated with cell growth, (F) apoptotic rate, (G,H) expression of proteins associated with apoptosis, and (I) migration rate of PC12 cells were detected by trypan blue exclusion, western blot, apoptosis assay and migration assay. n = 3. *p < .05 represents a comparison of marked groups.
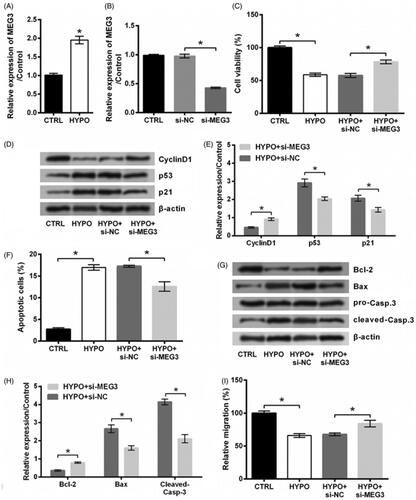
MiR-21 is a target of MEG3
MiR-21 has long been known as a target of MEG3 [Citation17–19]. This fact was also confirmed in PC12 cells. As shown in , the expression of miR-21 was increased in cells transfected by si-MEG3 as relative to si-NC transfected controls (p < .05). Besides this, relative luciferase activity was declined by transfecting with MEG3-wt and miR-21 mimic in comparison with the group transfected with MEG3-wt and NC mimic (p < .05, ). And there was no change of relative luciferase activity by transfecting with MEG3-mt and miR-21 mimic rather than transfecting with MEG3-mt and NC mimic (p > .05, ). These data revealed that MEG3 could repress miR-21 expression through directly binding effects. Then, qRT-PCR data displayed that, miR-21 level was lowly expressed in PC12 cells which are stimulated by hypoxia (p < .05, ), suggesting miR-21 as a downstream gene of MEG3.
Figure 3. miR-21 is a target of MEG3. (A) The expression of miR-21 in PC12 cells following transfection with MEG3 siRNA (si-MEG3) or its non-targeting control (si-NC) and in cells transfected with nothing served as control (CTRL) was tested by qRT-PCR. (B) Relative luciferase activity of PC12 cells co-transfected with the reporter vectors (MEG3-wt or MEG3-mt) and miR-21 mimic/NC mimic and in cells transfected with reporter vectors alone served as control was tested by luciferase reporter assay. (C) The expression of miR-21 in PC12 cells following hypoxia and in cells incubated at normoxic condition served as control (CTRL) was tested by qRT-PCR. n = 3. ns, no significant; *p < .05 represents a comparison of marked groups.
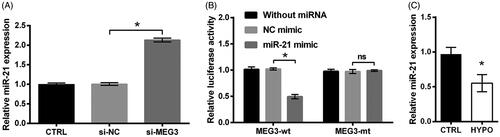
Silence of MEG3 prevents hypoxic injury via targeting miR-21
To examine the role of miR-21 in PC12 cells under hypoxic condition, the expression of miR-21 in cell was overexpressed by transfection with miR-21 mimic. Data in displayed that, miR-21 expression was high elevated by miR-21 mimic (p < .05, ). This suggested our transfection of miR-21 mimic was effective. Through the detection of above index of proliferation, apoptosis and migration, following data revealed that miR-21 mimic exhibited cardioprotective effects similar to MEG3 silence. As compared to transfection with NC mimic, transfection of cells with miR-21 mimic significantly increased cell growth, suppressed apoptosis and boosted migration in hypoxic cells (all p < .05, ).
Figure 4. miR-21 overexpression prevents hypoxic injury. (A) The expression of miR-21 in PC12 cells following transfection with miR-21 mimic or NC mimic and in cell transfected with nothing served as control (CTRL) was tested by qRT-PCR. PC12 cells were transfected with miR-21 mimic or NC mimic, and then incubated in hypoxia for 24 h. The cells without transfection in normoxic condition served as control (CTRL). (B) Cell viability, (C,D) expression of proteins associated with cell growth, (E) apoptotic rate, (F,G) expression of proteins associated with apoptosis, and (H) migration rate of PC12 cells were detected by trypan blue exclusion, western blot, apoptosis assay and migration assay. n = 3. *p < .05 represents a comparison of marked groups.
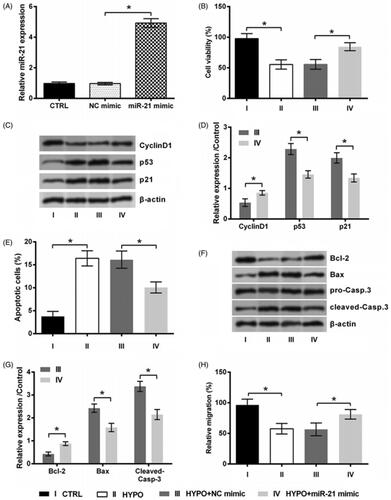
Following experiments aimed to reveal whether MEG3 silence protected PC12 cells against hypoxic injury via miR-21. To this end, miR-21 expression was suppressed by inhibitor transfection. Transfection efficiency was tested by qRT-PCR and results displayed a significant miR-21 down-regulation (p < .05, ). This suggested our transfection of miR-21 mimic was effective. We found that, si-MEG3 did not protect PC12 cells against hypoxic injury when miR-21 inhibitor was co-transfected into cells. To be specific, co-transfection of cells with si-MEG3 and miR-21 inhibitor significantly reversed above changes of cell growth, apoptosis and migration-associated index induced by si-MEG3 (all p < .05, ). These results illustrated that silence of MEG3 prevents hypoxic injury via targeting miR-21
Figure 5. Silence of MEG3 prevents hypoxic injury via targeting miR-21. (A) The expression of miR-21 in PC12 cells following transfection with miR-21 inhibitor or NC inhibitor and in cell transfected with noting served as control (CTRL) was tested by qRT-PCR. PC12 cells were co-transfected with si-MEG3/si-NC and miR-21 inhibitor/NC inhibitor, and then incubated in hypoxia for 24 h. The cells without transfection in normoxic condition served as control (CTRL). (B) Cell viability, (C,D) expression of proteins associated with cell growth, (E) apoptotic rate, (F,G) expression of proteins associated with apoptosis, and (H) migration rate of PC12 cells were detected by trypan blue exclusion, western blot, apoptosis assay and migration assay. n = 3. *p < .05 represents a comparison of marked groups.
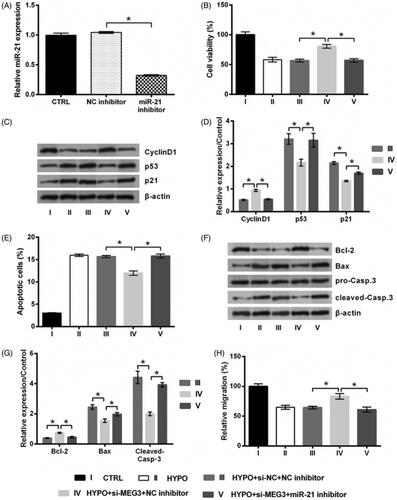
Silence of MEG3 activates PI3K/AKT pathway while deactivates NF-κB pathway via miR-21
Finally, the PI3K/AKT and NF-κB signalling pathways involved in hypoxic injury were studied to further expand our understanding of MEG3. showed that, the expression of PTEN and the phosphorylation of IκBα and p65 were increased by hypoxia stimulation, while the phosphorylation of PI3K and AKT was suppressed accordingly (all p < .05). The aberrant expression of these proteins induced by hypoxia was attenuated by transfection with si-MEG3 (all p < .05). However, si-MEG3 could not that significantly attenuate hypoxia-induced protein deregulation when miR-21 was silenced (all p < .05). Thus, these results explained that the silence of MEG3 could induce the activation of PI3K/AKT pathway and the inactivation of NF-κB pathway through the up-regulation of miR-21.
Figure 6. Silence of MEG3 activates PI3K/AKT pathway while deactivates NF-κB pathway via targeting miR-21. PC12 cells were co-transfected with si-MEG3/si-NC and miR-21 inhibitor/NC inhibitor, and then incubated in hypoxia for 24 h. The cells without transfection in normoxic condition served as control (CTRL). The expression of proteins involved in (A,B) PI3K/AKT and (C,D) NF-κB pathways was tested by western blot. n = 3.*p < .05 represents a comparison of marked groups.
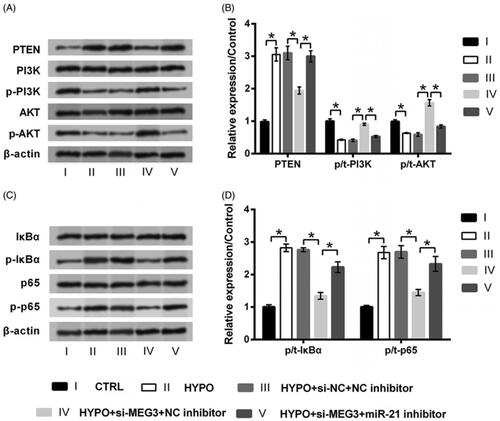
Discussion
Brain is a hypoxia-sensitive organ. The serious anoxia of brain leads to irreversible injuries including impairment on cognitive, language, action capabilities and so forth, which even can be life-threatening. The brain anoxia is often caused by hypoxia in plateau environment and ischaemia. In the present work, a cell model of HIBD was made by stimulating PC12 cells with hypoxia as previous studies performed [Citation20,Citation21]. Down-regulation of MEG3 by siRNA-mediated silencing significantly prevented hypoxia induced injury in PC12 cells. Besides this, MEG3 was found to be a miR-21 sponge, the neuroprotective function of MEG3 silence was flattened when miR-21 was silenced simultaneously. Furthermore, MEG3 silence activated PI3K/AKT pathway while deactivated NF-κB pathway via regulating miR-21 expression.
Apoptosis is the main way of neuron death caused by HIBD. Preventing hypoxic or ischaemic conditions induced neuron apoptosis is an area of ongoing research. Multiple lncRNAs have been reported to be associated with neuron apoptosis. As the selected examples, lncRNA MALAT1 [Citation22] and lncRNA H19 [Citation23] contributed to the apoptosis of hippocampal neurons. The present work illustrated that silence of MEG3 prevented hypoxia-induced cell viability loss and apoptosis. Mechanistically, silence of MEG3 exerted protective functions possible via regulating proteins associated with proliferation and apoptosis, like CyclinD1, p53, p21, Bcl-2, Bax and caspase-3. These findings were consistent with previous studies, in which the neuroprotective effect of MEG3 silence against various stimulates has been reported [Citation8–10], except hypoxia.
It is widely established that the central nervous system is almost incapable of self-repair and regeneration. Thus, neurons migrate to the injury sites might be an effective strategy for recovery of nerve function [Citation24]. Nowadays, research of lncRNAs provides new insights into neuron migration. Liu et al., reported that, overexpression of lncRNA NEAT1 promoted the migration of Schwann cells [Citation25]. Likewise, suppressing the expression of lncRNA ANRIL aggravated H2O2−induced migration impairment in PC12 cells [Citation26]. Our study for the first time illustrated that, silence of MEG3 promoted the migratory capacity of PC12 cells. The result indicated that, targeting MEG3 not only could prevent hypoxia-induced cell death, but also could promote neuroregeneration by controlling of neurons migration.
miRNAs have been considered as key mediators in the pathogenesis of HIBD [Citation27]. During HIBD, significant changes of miRNA expression are occurred, and they modulate various aspects of pathogenic processes, including atherosclerosis [Citation28], plaque rupture [Citation29], and stroke [Citation30]. miR-21 is one of such miRNAs that attenuates OGD induced neuron death [Citation14]. Besides this, miR-21 was reported to be a target of MEG3, via which silence of MEG3 exerted its neurological functions during HIBD [Citation9]. In line with those findings, the present study demonstrated that MEG3 acted as a miR-21 sponge. Further experiments demonstrated that, silence of MEG3 could not that significantly attenuated hypoxia-induced PC12 cell damage when miR-21 was suppressed. We for the first time illustrated the cardioprotective effects of MEG3 silence possible via sponging miR-21.
PI3K/AKT is a classic signalling pathway determines the survival and apoptosis of cells. PTEN is a phosphatase that antagonises the activity of PI3K by dephosphorylating PIP3 to PIP2. Since its regulatory role in cell fate, PI3K/AKT signalling pathway serves as a neuroprotective function at the early stage of ischaemic damage [Citation31]. NF-κB, a key transcription factor involved with immune and inflammatory responses as well as cell growth, is regulated primarily through interaction with an inhibitor protein known as IκBα. Activation of NF-κB pathway regulates genes involved in apoptosis and inflammation. During HIBD, NF-κB pathway is activated and contributes to irreversible brain damage [Citation32]. Based on these facts, it seems that activation of PI3K/AKT pathway and inactivation of NF-κB pathway may be promising candidates for HIBD treatment. Herein, the deactivated PI3K/AKT pathway and the activated NF-κB pathway induced by hypoxia were found to be attenuated by MEG3 silence. Besides this, the effects of MEG3 silence on these two signalling were flattened when miR-21 was suppressed. These finding collectively suggested that MEG3 silence prevent hypoxia injury in PC12 cells possibly through targeting miR-21 and thereby regulating the activation of PI3K/AKT and NF-κB pathways.
Recently, an increasing amount of literatures have reported the importance of lncRNAs in regulating the pathophysiology of post-stroke brain damage [Citation33]. Thus, the clinic value of lncRNAs in patient with HIBD was also studied. For instance, a large amount of lncRNAs were differently expressed in stroke patients as relative to normal controls, suggesting the potential of lncRNAs as stroke biomarkers [Citation34]. Likewise, a clinical study revealed MEG3 as one of such biomarker, and its expression was associated with the risk factors of HIBD, such as hypertension, total cholesterol, and triglyceride [Citation35]. Besides that, targeting MEG3 has potent therapeutic potentials, as in vitro experiments have demonstrated the cardioprotective effects of MEG3 silence. However, if targeting MEG3 can be used as a treatment application in HIBD remains further experiments. And several limitations need to be solved before clinical practice, like lncRNA delivery and the deep mechanism of lncRNAs’ function.
Conclusion
In conclusion, the neuroprotective effects of MEG3 silence on PC12 cells injured by hypoxia were observed in this study. Silence of MEG3 protected PC12 cells through preventing apoptosis and promoting migration. Mechanistically, MEG3 acted as a miR-21 sponge, MEG3 silence activated PI3K/AKT pathway and deactivated NF-κB pathway in a miR-21-dependent way.
Disclosure statement
No potential conflict of interest was reported by the author(s).
References
- Silveira RC, Procianoy RS. Hypothermia therapy for newborns with hypoxic ischemic encephalopathy. J Pediatr. 2015;91(6):S78–S83.
- Gowda R, Jaffa M, Badjatia N. Thermoregulation in brain injury. Handb Clin Neurol. 2018;157:789–797.
- Chen Y, Zhou J. LncRNAs: macromolecules with big roles in neurobiology and neurological diseases. Metab Brain Dis. 2017;32(2):281–291.
- Wu GC, Pan HF, Leng RX, et al. Emerging role of long noncoding RNAs in autoimmune diseases. Autoimmun Rev. 2015;14(9):798–805.
- Uchida S, Dimmeler S. Long noncoding RNAs in cardiovascular diseases. Circ Res. 2015;116(4):737–750.
- Rafiee A, Riazi-Rad F, Havaskary M, et al. Long noncoding RNAs: regulation, function and cancer. Biotechnol Genet Eng Rev. 2018;3:1–28.
- Cao MX, Jiang YP, Tang YL, et al. The crosstalk between lncRNA and microRNA in cancer metastasis: orchestrating the epithelial-mesenchymal plasticity. Oncotarget. 2017;8(7):12472–12483.
- You D, You H. Repression of long non-coding RNA MEG3 restores nerve growth and alleviates neurological impairment after cerebral ischemia-reperfusion injury in a rat model. Biomed Pharmacother. 2019;111:1447–1457.
- Yan H, Rao J, Yuan J, et al. Long non-coding RNA MEG3 functions as a competing endogenous RNA to regulate ischemic neuronal death by targeting miR-21/PDCD4 signaling pathway. Cell Death Dis. 2017;8(12):3211.
- Liu X, Hou L, Huang W, et al. The mechanism of long non-coding RNA MEG3 for neurons apoptosis caused by hypoxia: mediated by miR-181b-12/15-LOX signaling pathway. Front Cell Neurosci. 2016;10:201.
- Shen J, Zhao Z, Shang W, et al. Fabrication of a nano polymer wrapping Meg3 ShRNA plasmid for the treatment of cerebral infarction. Artif Cells Nanomed Biotechnol. 2018;46(sup2):894–903.
- Zhou XM, Liu J, Wang Y, et al. Silencing of long noncoding RNA MEG3 enhances cerebral protection of dexmedetomidine against hypoxic-ischemic brain damage in neonatal mice by binding to miR-129-5p. J Cell Biochem. 2018;120(5):7978–7988.
- Zhang Z, Xu P, Yu H, et al. Luteolin protects PC-12 cells from H2O2-induced injury by up-regulation of microRNA-21. Biomed Pharmacother. 2019;112:108698.
- Chen R, Tai Y, Zhang Y, et al. MicroRNA-21 attenuates oxygen and glucose deprivation induced apoptotic death in human neural stem cells with inhibition of JNK and p38 MAPK signaling. Neurosci Lett. 2019;690:11–16.
- Li R, Wang Y, Yang Z, et al. Hypoxia-inducible factor-1alpha regulates the expression of L-type voltage-dependent Ca(2+) channels in PC12 cells under hypoxia. Cell Stress Chaperones. 2015;20(3):507–516.
- Strober W. Trypan blue exclusion test of cell viability. Curr Protoc Immunol. 2015;111(1):A3.b.1–3.
- Wu Z, He Y, Li D, et al. Long noncoding RNA MEG3 suppressed endothelial cell proliferation and migration through regulating miR-21. Am J Transl Res. 2017;9(7):3326–3335.
- Zhou X, Yuan P, Liu Q, et al. LncRNA MEG3 regulates imatinib resistance in chronic myeloid leukemia via suppressing microRNA-21. Biomol Ther. 2017;25(5):490–496.
- Zhang LL, Hu D, Zou LH. Low expression of lncRNA MEG3 promotes the progression of oral squamous cell carcinoma by targeting miR-21. Eur Rev Med Pharmacol Sci. 2018;22(23):8315–8323.
- Zhang YH, Zhao ZY, Wang BJ, et al. Protective effect of Schisandra chinensis lignans on hypoxia-induced PC12 cells and signal transduction. J Toxicol Environ Health A. 2018;81(24):1224–1230.
- Yu W, Lv Z, Zhang L, et al. Astragaloside IV reduces the hypoxia-induced injury in PC-12 cells by inhibiting expression of miR-124. Biomed Pharmacother. 2018;106:419–425.
- Wu Q, Yi X. Down-regulation of long noncoding RNA MALAT1 protects hippocampal neurons against excessive autophagy and apoptosis via the PI3K/Akt signaling pathway in rats with epilepsy. J Mol Neurosci. 2018;65(2):234–245.
- Han CL, Ge M, Liu YP, et al. Long non-coding RNA H19 contributes to apoptosis of hippocampal neurons by inhibiting let-7b in a rat model of temporal lobe epilepsy. Cell Death Dis. 2018;9(6):617.
- Kaneko N, Herranz-Perez V. New neurons use Slit-Robo signaling to migrate through the glial meshwork and approach a lesion for functional regeneration. Sci Adv. 2018;4(12):eaav0618.
- Liu X, Yu X, He Y, et al. Long noncoding RNA nuclear enriched abundant transcript 1 promotes the proliferation and migration of Schwann cells by regulating the miR-34a/Satb1 axis. J Cell Physiol. 2019;234(9):16357–16366.
- Li R, Yin F, Guo YY, et al. Knockdown of ANRIL aggravates H2O2-induced injury in PC-12 cells by targeting microRNA-125a. Biomed Pharmacother. 2017;92:952–961.
- Rink C, Khanna S. MicroRNA in ischemic stroke etiology and pathology. Physiol Genomics. 2011;43(10):521–528.
- Price NL, Rotllan N, Canfran-Duque A, et al. Genetic dissection of the impact of miR-33a and miR-33b during the progression of atherosclerosis. Cell Rep. 2017;21(5):1317–1330.
- Li S, Lee C, Song J, et al. Circulating microRNAs as potential biomarkers for coronary plaque rupture. Oncotarget. 2017;8(29):48145–48156.
- Lv B, Cheng X, Sharp FR, et al. MicroRNA-122 mimic improves stroke outcomes and indirectly inhibits NOS2 after middle cerebral artery occlusion in rats. Front Neurosci. 2018;12:767.
- Li X, Zhang J, Zhu X, et al. Progesterone reduces inflammation and apoptosis in neonatal rats with hypoxic ischemic brain damage through the PI3K/Akt pathway. Int J Clin Exp Med. 2015;8(5):8197–8203.
- Ridder DA, Schwaninger M. NF-kappaB signaling in cerebral ischemia. Neuroscience. 2009;158(3):995–1006.
- Vemuganti R. All’s well that transcribes well: non-coding RNAs and post-stroke brain damage. Neurochem Int. 2013;63(5):438–449.
- Dykstra-Aiello C, Jickling GC, Ander BP, et al. Altered expression of long noncoding RNAs in blood after ischemic stroke and proximity to putative stroke risk loci. Stroke. 2016;47(12):2896–2903.
- Han X, Zheng Z, Wang C, et al. Association between MEG3/miR-181b polymorphisms and risk of ischemic stroke. Lipids Health Dis. 2018;17(1):292.