Abstract
Accumulating evidence indicates that oxidative stress and inflammation are implicated in brachial plexus avulsion (BPA). Quercetin has anti-inflammatory, anti-oxidant, anti-apoptotic, and neuroprotective properties. This study investigated the therapeutic efficacy of a temperature-sensitive poly(D,L-lactide-co-glycolide)-poly(ethylene-glycol)-poly(D,L-lactide-co-glycolide) (PLGA-PEG-PLGA) hydrogel sustained-release system of quercetin in BPA. In situ injections of the hydrogel loaded with different concentrations of quercetin were conducted in a rat model of BPA. Significantly reduced reactive oxygen species and interleukin-6 levels in the injured spinal cord 24 h post-surgery, increased number of anterior horn motor and functional neurons in the spinal cord 6 weeks post-surgery, thickened biceps muscle fibres and enlarged endplate area with clear structure, reduced demyelinated peripheral nerves, and significantly increased Terzis grooming test scores were found in the groups with 50 or 100 mg/mL quercetin-loaded hydrogels compared with the control and blank hydrogel groups. In conclusion, the temperature-sensitive quercetin loaded PLGA-PEG-PLGA hydrogel sustained-release system can alleviate oxidative damage and inflammation in the spinal cord, increase neuron survival rate, and promote nerve regeneration and motor function recovery in rats with early BPA. The findings suggest that this drug-loaded hydrogel has potential applications in the clinical treatment of BPA.
Introduction
Brachial plexus avulsion (BPA) is a severe injury of the brachial plexus involving the central and peripheral nerves [Citation1]. It can lead to loss of sensory and motor function in the upper limbs, with severe sequelae. In the acute phase, primary lesions and the ensuing cascade of secondary oxidative damage and inflammatory reactions lead to widespread neuronal death, making nerve regeneration difficult [Citation2]. Therefore, effective anti-inflammatory and anti-oxidative treatments in the early stage of injury might alleviate secondary damage and promote nerve regeneration and functional recovery.
Quercetin, a natural flavonoid that was discovered in plants, has neuroprotective effects and promotes neurological recovery in the central nervous system because of its anti-inflammatory, anti-oxidant and anti-apoptotic effects [Citation3,Citation4]. However, quercetin has poor water solubility (10 mg/L in water at ambient temperature) and various metabolic pathways and undergoes rapid elimination, resulting in limited absorption and low bioavailability [Citation5,Citation6]. Therefore, the therapeutic application of quercetin in BPA has been limited. In addition, local blood circulation disorders after BPA impair effective drug delivery via the systemic circulation. Accordingly, studies have attempted to treat BPA by injecting the drug directly into the injury site [Citation7,Citation8], but the irregular lesion cavity after BPA leads to excessive drug loss and low drug concentration in the target cells or tissues. Therefore, the local application of a sustained-release delivery system of drugs, such as quercetin, is especially necessary in the treatment of BPA.
With the rapid development of tissue engineering, implanting drug-loaded biomaterials into injury sites to promote nerve repair has become a research hotspot [Citation9], and polymer stents/catheters and hydrogels are the most widely used drug-loaded biomaterials [Citation10,Citation11]. However, due to the irregular shape of BPA, it is difficult to make the nerve conduit or stent match the morphology of the damage and to implant it into the ideal position. Injectable biodegradable hydrogels have attracted much attention because of their good biocompatibility, safety, injectability, fluidity, gel-forming property and convenience of operation [Citation12–14], and the hydrogel can quickly complete its sol to gel transition based on temperature changes. It is a solution at room temperature, and rapidly converts into a gel at 37 °C, even in a dose- and time-dependent manner [Citation15,Citation16]. Notably, hydrogels including the amphiphilic triblock copolymer poly(D,L-lactide-co-glycolide)-poly(ethylene-glycol)-poly(D,L-lactide-co-glycolide) (PLGA-PEG-PLGA) have been used for the preparation of temperature-sensitive hydrogels as a sustained-release matrix of small molecule hydrophobic and macromolecular drugs in the treatment of tumour and spinal cord injury [Citation12,Citation17–19]. This study prepared a hydrogel sustained-release system loaded with quercetin and based on PLGA-PEG-PLGA to allow the direct action of quercetin in the injury site and used the hydrogel to temporarily fill the irregular cavity after nerve root avulsion to creating favourable conditions for nerve regeneration and functional recovery. In addition, the therapeutic efficacy of the temperature-sensitive PLGA-PEG-PLGA hydrogel sustained-release system of quercetin for BPA was investigated in a rat BPA model.
Materials and methods
Experimental reagents
PEG (Mn = 1500 g/mol), D,L-Lactide (D, L-LA), glycolide (GA), Tin(II) 2-ethylhexanoate (Sn(Oct)2), methyl thiazolyl tetrazolium and quercetin were purchased from Sigma-Aldrich (Germany); sheep anti-rat choline acetyltransferase (ChAT) antibody was purchased from Millipore (Germany); rabbit anti-goat fluorescence 568 antibody and alpha-bungarotoxin (α-BTX) 488 antibody were purchased from Abcam (United States). Fluorescent gold (FG) and interleukin 6 (IL-6) ELISA kit were purchased from Fluorochorome (United States) and Mabtech (Sweden), respectively. All chemical reagents could be used directly without further purification.
Synthesis of PLGA − PEG − PLGA triblock copolymer
As described previously [Citation12,Citation20], PEG was used as a macroinitiator, with Tin(II) 2-ethylhexanoate as a catalyst to polymerize D, L-LA and GA into PLGA-PEG-PLGA triblock polymers by ring-opening polymerisation. Briefly, 10 g of PEG, 18.8 g of D, L-LA, and 2.5 g of GA were dehydrated under vacuum drying for 48 h, followed by incubation with 30 mg of Tin(II) 2-ethylhexanoate at 120 °C for 48 h. The reaction product was sufficiently dissolved in 4 °C cold water, and was then heated to 80 °C for precipitation and purification. Purification was repeated thrice, and the purified sample was dried in a vacuum for later use.
General characterization of PLGA–PEG–PLGA triblock copolymer
The composition and structure of the copolymer were identified by nuclear magnetic resonance with CDCl3 as a solvent and tetramethylsilane (TMS) as an internal standard, and the data were recorded using a Bruker DMX500 spectrometer at 500 MHz. The sol-gel transition temperature of PLGA-PEG-PLGA triblock copolymer was determined by the vial inversion test. Briefly, different weights of PLGA-PEG-PLGA polymer were dissolved in PBS and 0.5 ml of the polymer solution was stored in the vial for 15 min prior to the measurement. The temperature was raised in an increment of 1 °C. The gel was considered formed when the solution did not flow after the bottle was inverted for 30 s.
Release profiles of quercetin from PLGA-PEG-PLGA hydrogels
The PLGA-PEG-PLGA polymer was dissolved in distilled water containing acetone to prepare a solution with the optimal concentration of PLGA-PEG-PLGA. Quercetin was dissolved in acetone and was then added to the PLGA-PEG-PLGA solution, and the organic solvent was removed by evaporation. The quercetin-loaded PLGA-PEG-PLGA was added to a test tube with an inner diameter of 16 mm and incubated at 37 °C for 10 min. After the hydrogels were formed, 5 ml PBS was added to the top of the gels and then placed in a shaking box at 37 °C with continuous shaking at 60 rpm; 1 ml of PBS was taken at each time point while the same volume of PBS was added to the test tube. The amount of quercetin in 1 ml of PBS extract was detected by an ultraviolet spectrophotometer at 373 nm, and the drug release profile was plotted using the obtained absorbance values.
Ethics statement
All animal procedures reviewed and approved by the Institutional Animal Care and Use Committee of Jilin University, China (Ethical Approval No. 2019-91) and were performed in accordance with the guidelines for the Care and Use of Laboratory Animals adopted and promulgated by the USA National Institute of Health [Citation21]. Every effort was made to minimize the number of animals used in the experiments and their pain.
Laboratory animals and grouping
Adult healthy female Sprague-Dawley rats, aged 10–12 weeks and weighing 200–250 g, were provided by the Animal Experimental Centre of Jilin University. They were housed in standard animal rooms at 22 °C, with free access to food (granular feed) and water. The rats were randomly divided into six groups (n = 15/group): control group (group A), blank hydrogel group (group B), 5 mg/mL quercetin-loaded hydrogel group (group C), 10 mg/mL quercetin-loaded hydrogel group (group D), 50 mg/mL quercetin-loaded hydrogel group (group E), and 100 mg/mL quercetin-loaded hydrogel group (group F).
Model preparation and treatment
Rats were anaesthetized using isoflurane (2.5% in a 50:50 air: oxygen mixture) [Citation22]. The BPA model was established according to the GU method [Citation23]. Briefly, the rat neck hair was shaved, and the head was fixed on the sterile operating table. For routine disinfection by iodine volt, a median incision of approximately 3 cm was made from the occiput of the head to the upper corner of the shoulder blade. The second thoracic spinous process (T2) was taken as the bone marker, and the right cervical longus muscle and the cervical semi-spinous muscle were separated layer by layer and pulled and fixed with a small metal hook, which exposed the C4–T2 vertebral plate on the right side of the rat. The C4–C7 right vertebral plates were then removed with a bone forceps, and the C5, C6, and C7 spinal roots of the right brachial plexus nerve were separated with the aid of a surgical microscope. It should be noted that the spinal cord was not injured. The posterior C5, C6, and C7 roots were cut off and raised, and the anterior roots were exposed. The C5–C7 nerve roots were then gently avulsed with a self-made glass hook. The C6 anterior root was resected back to the original position and the posterior root was placed above the anterior root. Rats in group A were given a direct suture incision, and rats in the other groups were injected with 10 μl of quercetin at 0, 5, 10, 50, and 100 mg/mL around the replanted C6 nerve root. The hydrogel was held for 3 min until stabilized, and the incision was then sutured. After surgery, the animals were kept in a clean environment and given anti-infection treatment with an intramuscular injection of cefazolin sodium (50 mg/kg, twice a day; Zhongnuo Pharmaceutical) for 3 consecutive days. On the first and second day post-surgery, the upper limb was graded 0 in the Terzis grooming test (TGT) [Citation24], suggesting a successful generation of the animal model.
Determination of reactive oxygen species (ROS) in the injured spinal cord in the acute phase using immunofluorescence assay
Twenty-four hours post-surgery, 3 rats from each group were randomly selected, and they were administered intraperitoneally with 1 mg/mL dihydroethidium (DHE) (10 mg/kg) [Citation25] and were then anaesthetized with isoflurane (2.5% in a 50:50 air: oxygen mixture) 4 h later. Subsequently, the rats were perfused intracardially with 1× PBS followed by 4% paraformaldehyde (Solarbio, Xiamen Shike Biotechnology Co., Ltd., Fujian, China). The C5–C7 segments of the spinal cord were extracted, dehydrated, sliced using a frozen slicer (transection at a thickness of 10 μm), and observed and photographed under a fluorescence microscope with a 570 nm excitation source. Five 50 × 50 μm regions were randomly selected from the anterior horn of the spinal cord using the Image-Pro image analysis software. The average fluorescence density was measured and quantitatively analyzed and the results were expressed as relative fluorescence intensities.
Spectrophotometry determination of IL-6 levels in the injured spinal cord in the acute phase
Twenty-four hours post-surgery, 3 rats from each group were randomly selected. The C5–C7 segments of the spinal cord (50 mg) were homogenized on ice for 10 min; the tissue homogenate was ultrasonically pulverized for 10 s, subjected to repeated freezing and thawing (-80 °C/37 °C) thrice, and centrifuged 14,000 rpm for 20 min at 4 °C. The supernatant (100 μL) was collected, and 2.9 ml of the enzyme reaction solution was added. The absorbance value was measured at 532 nm to calculate IL-6 levels according to the ELISA kit instructions.
Behavioural test
Between the 2nd and 6th weeks post-surgery, two investigators who did not participate in the pre-modeling performed the TGT once a week to determine the motor function of the biceps muscles of the affected upper limb [Citation24]. For the test, 0–5 ml of water was sprayed onto the neck of the rat with a syringe to elicit grooming behaviour that requires the forelimb. A 0–5 point scale was used to score the test and assess the function of the injured biceps brachii: 0 point, the upper limb does not respond; 1 point, the elbow on the affected side is bent, but the forelimb does not reach the nostrils; 2 points, forelimb on the affected side can reach the nose; 3 points, elbow flexion causes forelimb on the affected side to reach the front; 4 points, the forelimb on affected side can touch the eyes; 5 points, the forelimb on the affected side can reach the ears or beyond. The motor function of the right forelimb was graded separately and recorded by two people who did not participate in the pre-modeling using a blinded method. Divergences of opinion between the two investigators were independently resolved by a third investigator.
Assessment of the spinal anterior horn motor neurons in the C6 segment of the injured side using immunofluorescence assay and FG nerve retrograde labelling
At six weeks post-surgery, 3 rats from each group were randomly selected. The spinal cord was extracted and prepared as described above and was then subjected to ChAT staining, and the biceps muscle was used for subsequent motor endplate (MEP) and hematoxylin-eosin (H&E) staining. One out of every four sections was incubated with primary monoclonal ChAT antibody (1:100; Millipore) at 4 °C overnight and then with the secondary antibody (rabbit anti-goat fluorescence 568 antibody, Abcam) at room temperature for 1.5 h. ChAT-positive spinal anterior horn motor neurons in the injured side and the contralateral C6 segment were counted under a fluorescence microscope to calculate the cell ratio of the injured to the healthy sides.
Three rats were randomly selected from each group. After anaesthesia, an incision was transversely made beneath the right clavicle to cut off the pectoralis major and minor muscles, thereby exposing the musculocutaneous nerve. A 10 μm glass needle was inserted 5 mm proximal to the nerve entry point of the musculocutaneous nerve to the biceps muscle, and 0.8 μl of FG was injected into the musculocutaneous nerve with a microinjection pump. The needle was retained for 10 s until the FG was completely absorbed. After 3 days, the C5–C8 segments of the spinal cord (the C8 segment being used as a marker) were cut into frozen slices by a cryostat (slitting at a thickness of 25 μm), and one out of every four sections was observed under a fluorescence microscope to count the number of FG-labeled neurons.
Detection of musculocutaneous nerve remyelination using a transmission electron microscope
Six weeks post-surgery, 3 rats from each group were randomly selected. Musculocutaneous nerve remyelination was assessed using the method described by Li et al. [Citation26]. The portion of musculocutaneous nerve 5–10 mm proximal to the nerve entry point was extracted and cut into 90-nm thick ultrathin slices. Digital images were captured using the transmission electron microscope with 3% uranyl acetate and 1% lead citrate.
Detection of biceps muscle diameter changes in the injured side using H&E staining and measurement of the content and morphology of MEP neurons in the injured side of biceps muscle using immunofluorescence assay
The remaining biceps muscles were cut and sliced by a frozen slicer (14 μm slitting). One out of every four sections was used for H&E staining. Under the light microscope, 80–100 muscle fibres were randomly selected from each biceps muscle and photographed. The diameter of the muscle fibres was measured by the Image-J software (National Institutes of Health, Bethesda, MD, USA), and the average value was calculated. One out of every four sections was used for endplate staining and was incubated with α-BTX 488 antibody (Abcam) for 30 min. The morphology of the MEP was observed under the fluorescence microscope. Each specimen was randomly photographed with 100 MEPs, and the area was measured by Image-J software [Citation27].
Statistical analysis
Graph-Pad Prism 5.0 software (Graph-Pad Software, Inc., CA, USA) and SPSS 15.0 software (SPSS, Chicago, IL, USA) were used for statistical analyses. Data were analyzed with one-way ANOVA. Measurement results are presented as mean ± standard deviation (SD). p-Value of <.05 was considered statistically significant.
Results
Material characterisation
The composition and structure of the copolymer were examined by 1H NMR spectroscopy. As shown in , the peaks at 4.32 and 3.65 ppm were ascribed to the terminal methylene groups (CH2) of PEG and the CH2 of PEG; the peaks at 1.55 and 5.21 ppm were assigned to the methyl group (CH3) and the methine group (CH) of D, L-LA; the peak at 4.82 ppm was attributed to the CH2 of GA. Studies have shown that the sol-gel transition of the PLGA-PEG-PLGA hydrogel is closely related to its concentration [Citation28]. We used the vial inversion test to investigate the sol-gel transition of the hydrogels (), and a typical sol-gel-precipitation transition was observed at a hydrogel concentration of 20–30%. We found that higher PLGA-PEG-PLGA concentrations had higher gel-precipitation transition temperatures and lower sol-gel transition temperatures. A 30% PLGA-PEG-PLGA hydrogel, which was thought to have the appropriate sol-gel transition temperature, was used for further animal studies.
Figure 1. The 1H NMR spectrum of the PLGA-PEG-PLGA triblock copolymer. The peaks at 4.32 and 3.65 ppm were ascribed to the terminal methylene groups (CH2) of PEG and the CH2 of PEG. The peaks at 1.55 and 5.21 ppm were assigned to the methyl group (CH3) and the methine group (CH) of D, L-LA. The peak at 4.82 ppm was attributed to the CH2 of GA.
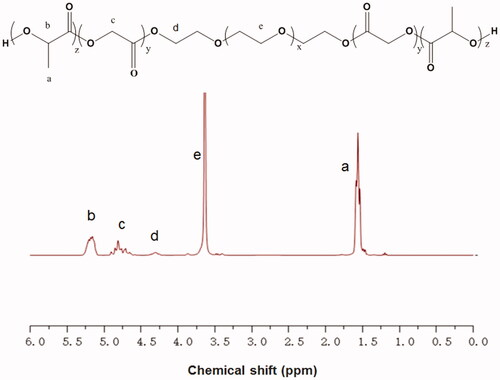
Release profiles of quercetin from PLGA–PEG–PLGA hydrogels
The release profile of quercetin in the PLGA-PEG-PLGA hydrogel is shown in . About 45% quercetin was rapidly released on the 1st day, and about 86% was cumulatively released in 14 days, with a relatively smooth sustained-release trend.
The quercetin-loaded hydrogel sustained-release system reduces the levels of ROS and IL-6 in the spinal cord in acute BPA
The ROS levels after treatment with the quercetin-loaded hydrogel sustained-release system decreased at 24 h post-surgery (), compared with those of the control (group A). The ROS levels were significantly lower in groups E and F than in groups A, B, and C ().
Figure 4. DHE fluorescence and IL-6 levels in the spinal cords in the control group, blank hydrogel group, 5 mg/mL quercetin-loaded hydrogel group, 10 mg/mL quercetin-loaded hydrogel group, 50 mg/mL quercetin-loaded hydrogel group, and 100 mg/mL quercetin-loaded hydrogel group 24 h after surgery. (A-F). DHE fluorescence in the anterior horn in the spinal cords in the 6 groups (red; n = 3, scale bar = 200 μm); (G) DHE fluorescence in the anterior horn in the spinal cord in the 6 groups 24 h after surgery (n = 3, *p < .05, **p < .01); (H) IL-6 levels in the 6 groups 24 h after surgery (n = 3, *p < .05, **p < .01).
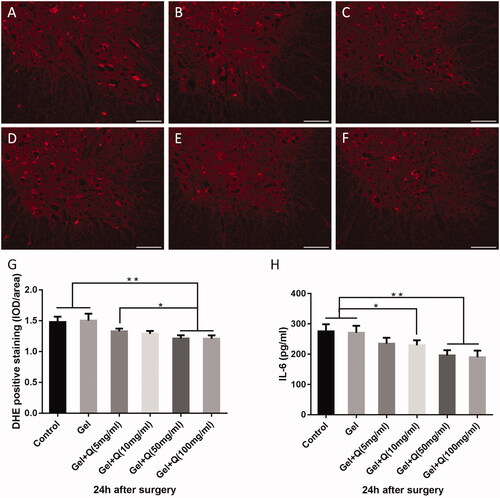
Significantly reduced IL-6 levels in the spinal cord were found 24 h after treatment with the quercetin-loaded hydrogel sustained-release system. The IL-6 levels were significantly lower in groups D, E, and F than in groups A and B, and the decrease of IL-6 levels was more significant in groups E and F compared with groups A and B ().
The quercetin-loaded hydrogel sustained-release system significantly improves the motor function of the injured biceps muscle after nerve root replantation for BPA
The quercetin-loaded hydrogel sustained-release system promoted upper limb motor function after nerve root replantation for BPA. TGT scores 2–6 weeks after surgery were higher in groups C–F than in groups A and B (). After 3 weeks, TGT scores were significantly higher in group F than in other groups. After 4 weeks, TGT scores were significantly higher in groups D, E, and F than in other groups. After 6 weeks (), TGT scores were significantly higher in groups D, E, and F (4.33, 4.50, 4.67, respectively) than in groups A and B (3.33, 3.50, respectively). A higher TGT score was observed in group C than in group A, but the difference was not statistically significant.
Figure 5. TGT scores in the control group, blank hydrogel group, 5 mg/mL quercetin-loaded hydrogel group, 10 mg/mL quercetin-loaded hydrogel group, 50 mg/mL quercetin-loaded hydrogel group, and 100 mg/mL quercetin-loaded hydrogel group 2–6 weeks after surgery (n = 6). (A) TGT scores measured 2–6 weeks after surgery in the 6 groups; (B). TGT scores 6 weeks after surgery in the 6 groups (n = 6, *p < .05).
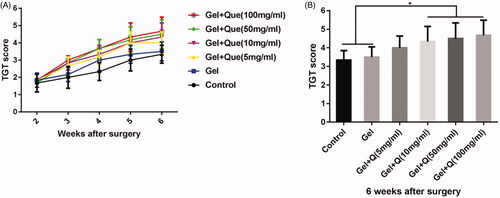
The quercetin-loaded hydrogel sustained-release system significantly increases the survival rate of anterior horn motoneurons and the number of functional motoneurons in the injured side
The volume and number of anterior horn motoneurons after treatment with the hydrogel system were increased in groups C–F compared with groups A and B (). The cell number ratios between the injured and healthy sides were 53.7, 55, 62, 64.7, 70.3, and 72% in groups A–F, respectively (). Groups D, E, and F showed a higher ratios than groups A and B, indicating significantly increased cell numbers in groups D–F.
Figure 6. Survival rates and cell numbers of anterior horn motoneurons in the injured side of the spinal cord 6 weeks after surgery in the control group, blank hydrogel group, 5 mg/mL quercetin-loaded hydrogel group, 10 mg/mL quercetin-loaded hydrogel group, 50 mg/mL quercetin-loaded hydrogel group, and 100 mg/mL quercetin-loaded hydrogel group. (A–F) ChAT (+) neuron staining (red) and nuclear DAPI staining (blue) of the C6 spinal cord anterior horn in the injured side (scale bar = 100 μm); (G–L). The fluorescence side of the C5–C8 spinal anterior horn of the injured side was reversed (n = 3, scale bar = 100 μm); (M) The ratio of C6 spinal anterior horn to contralateral spinal anterior horn motor neurons in the injured side in the 6 groups (n = 3, *p < .05); (N) Number of fluorescent gold reverse neurons in the C5–C8 spinal cord in the injured side in the 6 groups (n = 3, *p < .05).
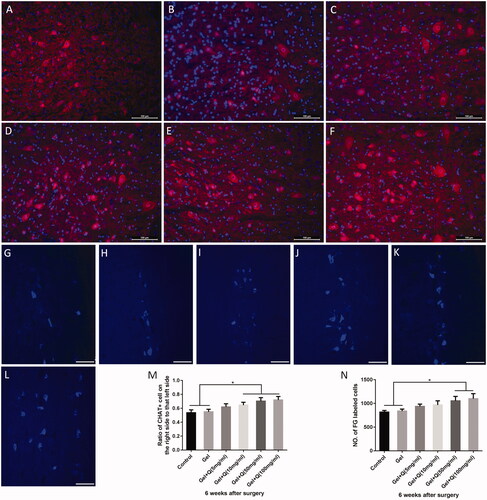
The FG nerve retrograde labelling of the C5–C8 segments showed that the number of functional neurons in the spinal cord increased significantly after treatment with the quercetin-loaded hydrogel system (); the cell number was significantly increased in groups E and F compared with groups A and B ().
The quercetin-loaded hydrogel sustained-release system promotes regeneration of biceps muscle fibres and MEPs in the injured side
Six weeks post-surgery, the area occupied by MEPs in the biceps muscle in the injured side was measured. As the concentration of quercetin increased, the MEP area also increased, and the MEP structure became clear and complex, indicating the functional recovery of MEPs (). The area of MEPs was significantly higher in groups E and F (399.2 and 407.2 μm2) than in groups A and B (327.4 and 338.0 μm2). In addition, the area of MEPs was also higher in groups C and D (364.9 and 376.9 μm2) than groups A and B, but the differences were not statistically significant ().
Figure 7. Fluorescence staining of MEPs and H&E staining of biceps muscle fibres in the injured side 6 weeks after surgery in the control group, blank hydrogel group, 5 mg/mL quercetin-loaded hydrogel group, 10 mg/mL quercetin-loaded hydrogel group, 50 mg/mL quercetin-loaded hydrogel group, and 100 mg/mL quercetin-loaded hydrogel group. (A–F) MEP fluorescence staining (green) of the injured side of the biceps muscle in the 6 groups (scale bar = 20 μm); (G–L) H&E staining of the injured side in the 6 groups (scale bar = 50 μm); (M) The increase of the area of biceps MEPs in the injured side was statistically significant (*p < .05, **p < .01); (N) The diameter of biceps muscle fibres stained by H&E in the injured side was increased (*p < .05, **p < .01).
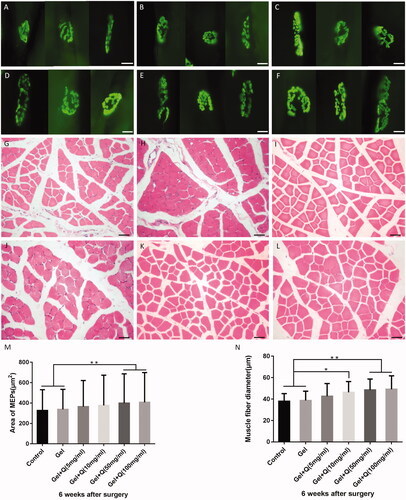
Six weeks post-surgery, the diameter of the injured biceps muscle fibres was significantly increased in groups D–F compared with groups A and B ().
Healthier myelin sheath and axons in the musculocutaneous nerve after treatment with the quercetin-loaded hydrogel sustained-release system
Transmission electron microscope was used to observe the ultrastructural changes of the myelin sheath and axons in the musculocutaneous nerve near the nerve entry point. As shown in , morphological characteristics of the myelin sheath and axons were better in groups C–F than in groups A and B, as indicated by reduced axonal swelling and visible multi-layer structure. In groups E and F, the lamellar structures were more compact, with basic morphology close to normal, although the musculocutaneous myelin sheath was slightly separated.
Figure 8. Electron micrograph of myelin sheath and axons in the musculocutaneous nerve near the nerve entry point 6 weeks after surgery in the control group (A), blank hydrogel group (B), 5 mg/mL quercetin-loaded hydrogel group (C), 10 mg/mL quercetin-loaded hydrogel group (D), 50 mg/mL quercetin-loaded hydrogel group (E), and 100 mg/mL quercetin-loaded hydrogel group (F).
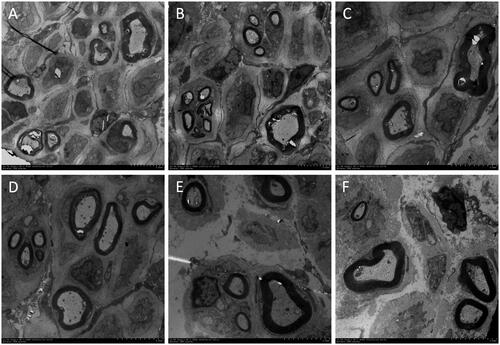
Discussion
BPA occurs in the transitional regions of the peripheral and the central nervous system, and involving the corresponding spinal segments, leading to loss of sensory and motor functions in the dominating region. In 70% of the cases, it also affects the surrounding spinal segments, resulting in a “longitudinal spinal cord lesion” [Citation29]. The primary trauma can produce a mechanical shock, and within a few minutes, an excessive amount of ROS is produced at the injury site, causing an oxidative stress reaction, resulting in irreversible damage to lipids, proteins, and DNA. Meanwhile, inflammatory factors such as IL-6, interleukin-1β (IL-1β), and tumour necrosis factor-α (TNF-α) recruit a large number of inflammatory cells to the injured site after BPA, reaching a peak between 24 and 72 h after injury [Citation30]. The interaction between the inflammatory response and oxidative stress amplifies the cascade reaction and induces extensive degeneration and necrosis of spinal motoneurons, resulting in impaired nerve regeneration and functional recovery [Citation31,Citation32].
Quercetin has anti-inflammatory, anti-oxidant, and neuroprotective effects, which can promote the recovery of nerve function after central nervous system injury. However, it is difficult to achieve an effective therapeutic concentration of quercetin at the injured site due to poor water solubility and low bioavailability [Citation6]. Thermo-sensitive hydrogels such as PLGA-PEG-PLGA hydrogels can rapidly release the loaded hydrophobic small molecule drug during the diffusion control phase, and then sustain the release during the polymer degradation control phase, thereby achieving sustained release of the hydrophobic drug. Therefore, the PLGA-PEG-PLGA hydrogel has been widely used in local drug delivery for targeted therapy [Citation16]. In this study, we found that in situ local delivery of quercetin could promote nerve regeneration and functional recovery in BPA rats.
We successfully synthesized and characterized the PLGA–PEG–PLGA hydrogel in this study. The polymer solution was rapidly transformed into a gel at 37 °C, and the sol-gel transition of the hydrogel with temperature was demonstrated by the vial inversion test. Because of a suitable critical gel temperature, the 30% hydrogel was used as the quercetin-loaded carrier for subsequent experiments. In vitro drug release experiments showed that the drug-loaded system released about 45% of the drug on the 1st day, and at a slower rate between the 2nd and the 14th days. By the 14th day, the cumulative release of quercetin was up to 86%, thus achieving long-term sustained release, and the findings are in line with those in the study by Hoare and Kohane [Citation16]. Notably, inflammatory responses reach a peak within 24–72 h after BPA; therefore, early release of a high dose of quercetin at the injury site might effectively inhibit the release of pro-inflammatory factors, reduce oxidative stress, and improve the survival rate of neuronal cells [Citation33]. At the same time, the PLGA-PEG-PLGA hydrogel has been proved to have a degradation cycle in vivo lasting more than 1 month. The degradation products of the PLGA segment are lactic acid and glycolic acid, which are involved in human metabolism [Citation34,Citation35]. The PEG segment is a hydrophilic segment that is non-toxic, non-antigenic, and non-immunogenic, and cannot damage the nerves in the later stages of treatment. Therefore, the hydrogel has good biocompatibility, high safety, and well-defined release characteristics. It provides a stable drug enrichment in the injury side for a long time, allowing quercetin to exert its anti-inflammatory and anti-oxidation effects to reduce secondary damage in the injured area [Citation36,Citation37]. It also solves the problem of low bioavailability of intraperitoneal or intravenous infusions of quercetin [Citation38].
Twenty-four hours after BPA, DHE immunofluorescence in the injured spinal cord segment of groups E and F was significantly lower than in groups A and B. Meanwhile, the IL-6 levels in the injured spinal cord segment were reduced significantly in groups D, E, and F compared with groups A and B. This indicates that the hydrogel loaded with a larger concentration of quercetin, might better reduce oxidative stress and inflammatory response in secondary injury and better protect nerve tissues in the early stage of nerve injury.
In addition to reducing oxidative stress and inflammatory response, the quercetin-loaded hydrogel sustained-release system might attenuate neuronal autophagy and apoptosis by activating the PI3K/Akt pathway and inhibiting the ERK1/2 pathway [Citation39,Citation40]. Studies have shown that a large number of motor neurons begin to degenerate or die in the injured spinal cord segment 3 weeks after BPA, with a mortality rate of up to 80% 6 weeks after surgery [Citation41]. In this study, after treatment with different concentrations of the quercetin-loaded hydrogel sustained-release system, more motor neurons were retained in the injured spinal cord segment, providing favourable conditions and a basis for subsequent nerve repair. Compared with groups A and B, groups D, E and F displayed stronger neuroprotection, enabling 70% of the motoneurons to survive. Meanwhile, the number of functional neurons in the spinal cord was significantly increased in groups E and F compared with the other groups (as showed by FG reverse labelling). In the comparison between groups E and F, the latter had slightly more motoneurons than the former, but the difference was not statistically significant, indicating the existence of a maximal dose of quercetin in the treatment of BPA. Simply increasing the dose of the therapeutic drug not only wastes the drug, but also aggravate the body metabolic load. This conclusion is consistent with what Li et al.[Citation42] found in the treatment of TBI.
After replantation due to BPA, the axons regenerated from the anterior horn motoneurons of the spinal cord need to pass through the scar tissue of the peri-spinal nerve junction to the peripheral nerve in a distorted manner [Citation26]. This process increases the difficulty of axonal regeneration, prolongs the axonal regeneration time, and increases the probability of neural mismatch, thus resulting in reduced efficiency of axonal regeneration [Citation43,Citation44]. In this study, electron microscopy revealed that the quality of the axons and myelin sheaths near the nerve entry points of the musculocutaneous nerves was improved with increasing concentrations of quercetin. In groups A and B, myelin stratification was common, and axons and myelin sheaths were separated. After treatment with 50 and 100 mg/mL quercetin (groups E and F), the quality of axons and myelin sheaths was notably improved; intermyelin sheath layers were thickened, axonal and myelin sheath separation was decreased, and some morphological characteristics were close to normal.
Therefore, our findings suggest that the PLGA-PEG-PLGA hydrogel loaded with quercetin could promote the intrinsic growth of axons and enhance the recovery of peripheral nerve function. Regenerated axons can enter the muscles through the peripheral nerves and re-innervate the MEPs to achieve functional regeneration. We found that in groups E and F, compared with the other groups, the MEPs of the biceps muscle had a larger area and a clearer structure, and the diameter of muscle fibres was increased. Furthermore, the TGT score increased significantly 3 weeks after surgery in groups C–F compared with groups A and B, and the motor function of the biceps muscle recovered better 6 weeks after surgery in groups D, E, and F than groups A and B. The findings indicate that the temperature-sensitive hydrogel loaded with quercetin improves the ability of the replanted nerve to re-innervate the distal effector (muscle), and the differences were statistically significant.
Conclusions
In this study, we successfully generated an injectable temperature-sensitive PLGA-PEG-PLGA hydrogel composite loaded with quercetin to improve the delivery of therapeutic drugs after BPA. We characterized the composite material and found that the release of quercetin can be sustained throughout the whole stress inflammatory phase after nerve injury. The local release of quercetin can directly affect the damaged tissue, and overcome the shortcomings of quercetin, such as its low water solubility and low bioavailability. Our results showed that the sustained release of hydrogel loaded with different concentrations of quercetin could promote nerve regeneration and functional recovery at the injury site. The hydrogel loaded with 50 and 100 mg/mL quercetin was more effective, but there was no statistical difference between the two concentrations. Therefore, there is an upper limit to the dose of quercetin administered in the treatment of BPA, and increasing the drug concentration above the threshold does not further increase the benefits. In addition, the data from the blank PLGA-PEG-PLGA hydrogel group showed improvement compared with the control group, but not in a statistically significant way. In our previous BPA experiment, the hydrogel could fill the tissue gap and reduce scar tissues at the junction of spinal cord and peripheral nerves, providing a better channel for axonal growth, indicating that the hydrogel has a certain auxiliary effect in the treatment of BPA [Citation11,Citation45]. Although our results show the promising potential of gel and quercetin application in brachial plexus avulsion therapy, the molecular mechanisms governing quercetin’s regulation of functional recovery after BPA have not been fully investigated. Therefore, further studies are needed to investigate the mechanisms underlying the protective role of quercetin treatment in BPA. In summary, this study reveals that the drug-loaded hydrogel sustained-release system could achieve sustained-release of the therapeutic drug via local injection and promote nerve regeneration and functional recovery. The findings shed light on the potential applications of the injectable temperature-sensitive PLGA-PEG-PLGA hydrogel composite in the clinical treatment of BPA.
Author contributions
All authors substantially contributed to the manuscript and approved the final manuscript. Chao Huang and Chuan Fu contributed equally to this work.
Disclosure statement
No potential conflict of interest was reported by the author(s).
Additional information
Funding
References
- Wang L, Yuzhou L, Yingjie Z, et al. A new rat model of neuropathic pain: complete brachial plexus avulsion. Neurosci Lett. 2015;589:52–56.
- Ham TR, Leipzig ND. Biomaterial strategies for limiting the impact of secondary events following spinal cord injury. Biomed Mater. 2018;13(2):024105.
- Chen S, Jiang H, Wu X, et al. Therapeutic effects of quercetin on inflammation, obesity, and type 2 diabetes. Mediators Inflamm. 2016;2016:9340637.
- Suganthy N, Devi KP, Nabavi SF, et al. Bioactive effects of quercetin in the central nervous system: focusing on the mechanisms of actions. Biomed Pharmacother. 2016;84:892–908.
- Li Y, Yao J, Han C, et al. Quercetin, inflammation and immunity. Nutrients. 2016;8(3):167.
- Gao L, Liu G, Wang X, et al. Preparation of a chemically stable quercetin formulation using nanosuspension technology. Int J Pharm. 2011;404(1–2):231–237.
- Chen H-S, Zhou Z-H, Li M, et al. Contribution of brain-derived neurotrophic factor to mechanical hyperalgesia induced by ventral root transection in rats. Neuroreport. 2013;24(4):167–170.
- Fang X-Y, Zhang W-M, Zhang C-F, et al. Lithium accelerates functional motor recovery by improving remyelination of regenerating axons following ventral root avulsion and reimplantation. Neuroscience. 2016;329:213–225.
- Sun H, Cao D, Liu Y, et al. Low molecular weight heparin-based reduction-sensitive nanoparticles for antitumor and anti-metastasis of orthotopic breast cancer. Biomater Sci. 2018;6(8):2172–2188.
- Pan S, Qi Z, Li Q, et al. Graphene oxide-PLGA hybrid nanofibres for the local delivery of IGF-1 and BDNF in spinal cord repair. Artif Cells Nanomed Biotechnol. 2019;47(1):651–664.
- Wang T, Zeng LN, Zhu Z, et al. Effect of lentiviral vector-mediated overexpression of hypoxia-inducible factor 1 alpha delivered by pluronic F-127 hydrogel on brachial plexus avulsion in rats. Neural Regen Res. 2019;14(6):1069–1078.
- Ci T, Chen L, Yu L, et al. Tumor regression achieved by encapsulating a moderately soluble drug into a polymeric thermogel. Sci Rep. 2014;4:5473.
- Yan Q, Xiao L-Q, Tan L, et al. Controlled release of simvastatin-loaded thermo-sensitive PLGA-PEG-PLGA hydrogel for bone tissue regeneration: in vitro and in vivo characteristics. J Biomed Mater Res A. 2015;103(11):3580–3589.
- Nasrollahi P, Khajeh K, Tamjed E, et al. Sustained release of sodium deoxycholate from PLGA-PEG-PLGA thermosensitive polymer. Artif Cells Nanomed Biotechnol. 2018;46:1170–1177.
- Liu H, Cheng Y, Chen J, et al. Component effect of stem cell-loaded thermosensitive polypeptide hydrogels on cartilage repair. Acta Biomater. 2018;73:103–111.
- Hoare TR, Kohane DS. Hydrogels in drug delivery: progress and challenges. Polymer. 2008;49(8):1993–2007.
- Zhao Y-Z, Jiang X, Xiao J, et al. Using NGF heparin-poloxamer thermosensitive hydrogels to enhance the nerve regeneration for spinal cord injury. Acta biomaterialia. 2016;29:71–80.
- Ma H, He C, Cheng Y, et al. Localized co-delivery of doxorubicin, cisplatin, and methotrexate by thermosensitive hydrogels for enhanced osteosarcoma treatment. ACS Appl Mater Interfaces. 2015;7(49):27040–27048.
- Kubinová Š. Biomaterials and magnetic stem cell delivery in the treatment of spinal cord injury. Neurochem Res. 2020;45(1):171–179.
- Zhao J, Guo B, Ma PX. Injectable alginate microsphere/PLGA–PEG–PLGA composite hydrogels for sustained drug release. RSC Adv. 2014;4(34):17736–17742.
- National Research, Council, Promising, Interventions. The National Academies Collection: Reports funded by National Institutes of Health[J]. National Academies Press; 2009.
- Torres-Espín A, Forero J, Fenrich KK, et al. Eliciting inflammation enables successful rehabilitative training in chronic spinal cord injury. Brain. 2018;141(7):1946–1962.
- Gu H-Y, Chai H, Zhang J-Y, et al. Survival, regeneration and functional recovery of motoneurons in adult rats by reimplantation of ventral root following spinal root avulsion. Eur J Neurosci. 2004;19(8) :2123–2131.
- Bertelli JA, Mira J-C. Behavioral evaluating methods in the objective clinical assessment of motor function after experimental brachial plexus reconstruction in the rat. J Neurosci Methods. 1993;46(3) :203–208.
- Zhang B, Bailey WM, McVicar AL, et al. Age increases reactive oxygen species production in macrophages and potentiates oxidative damage after spinal cord injury. Neurobiol Aging. 2016;47:157–167.
- Li H, Wong C, Li W, et al. Enhanced regeneration and functional recovery after spinal root avulsion by manipulation of the proteoglycan receptor PTPσ. Sci Rep. 2015;5:14923.
- Wu H, Mei L. Morphological Analysis of Neuromuscular Junctions by Immunofluorescent Staining of Whole-Mount Mouse Diaphragms. Methods in Molecular Biology. 2013;1018:277–285.
- Liu P, Guo B, Wang S, et al. A thermo-responsive and self-healing liposome-in-hydrogel system as an antitubercular drug carrier for localized bone tuberculosis therapy. International journal of pharmaceutics. 2019;558:101–109.
- Carlstedt T, Havton L. The longitudinal spinal cord injury: lessons from intraspinal plexus, cauda equina and medullary conus lesions. In: Handbook of clinical neurology. Elsevier; 2012. p. 337–354.
- Naugler WE, Sakurai T, Kim S, et al. Inflammation, HCC and sex: IL-6 in the centre of the triangle. J Hepatol. 2008;48(2):380–381.
- Papastefanaki F, Matsas R. From demyelination to remyelination: the road toward therapies for spinal cord injury. Neural Inj Func Reconstruct. 2015;63(7):1101–1125.
- Cregg JM, DePaul MA, Filous AR, et al. Functional regeneration beyond the glial scar. Exp Neurol. 2014;253(1):197–207.
- Blits B, Carlstedt TP, Ruitenberg MJ, et al. Rescue and sprouting of motoneurons following ventral root avulsion and reimplantation combined with intraspinal adeno-associated viral vector-mediated expression of glial cell line-derived neurotrophic factor or brain-derived neurotrophic factor. Exp Neurol. 2004;189(2):303–316.
- Yu L, Zhang Z, Zhang H, et al. Biodegradability and biocompatibility of thermoreversible hydrogels formed from mixing a sol and a precipitate of block copolymers in water. Biomacromolecules. 2010;11(8):2169–2178.
- Martina KL, Ulrica E, Ann-Christine A. Covalent grafting of poly(L-lactide) to tune the in vitro degradation rate. Biomacromolecules. 2007;8(8) :2492.
- Lee JK, Kwak HJ, Piao MS, et al. Quercetin reduces the elevated matrix metalloproteinases-9 level and improves functional outcome after cerebral focal ischemia in rats. Acta neurochirurgica. 2011;153(6):1321–1329.
- Selvakumar K, Bavithra S, Krishnamoorthy G, et al. Polychlorinated biphenyls-induced oxidative stress on rat hippocampus: a neuroprotective role of quercetin. TheScientificWorldJournal. 2012;2012:980314.
- Cai X, Fang Z, Dou J, et al. Bioavailability of quercetin: problems and promises. Current Med Chem. 2013;20(20):2572–2582.
- Du G, Zhao Z, Chen Y, et al. Quercetin attenuates neuronal autophagy and apoptosis in rat traumatic brain injury model via activation of PI3K/Akt signaling pathway. Neurol Res. 2016;38(11):1012–1019.
- Yao R-Q, Qi D-S, Yu H-L, et al. Quercetin attenuates cell apoptosis in focal cerebral ischemia rat brain via activation of BDNF-TrkB-PI3K/Akt signaling pathway. Neurochem Res. 2012;37(12):2777–2786.
- Eggers R, Tannemaat MR, Ehlert EM, et al. A spatio-temporal analysis of motoneuron survival, axonal regeneration and neurotrophic factor expression after lumbar ventral root avulsion and implantation. Exp Neurol. 2010;223(1):207–220.
- Li X, Wang H, Wen G, et al. Neuroprotection by quercetin via mitochondrial function adaptation in traumatic brain injury: PGC-1α pathway as a potential mechanism. J Cell Mol Med. 2017;22(2):883–891.
- Zuo J, Hernandez YJ, Muir D. Chondroitin sulfate proteoglycan with neurite‐inhibiting activity is up‐regulated following peripheral nerve injury. J Neurobiol. 1998;34(1):41–54.
- Lang EM, Schlegel N, Sendtner M, et al. Effects of root replantation and neurotrophic factor treatment on long-term motoneuron survival and axonal regeneration after C7 spinal root avulsion. Exp Neurol. 2005;194(2):341–354.
- Ding L, Zhy Z, Wang Y, et al. LINGO-1 shRNA loaded by Pluronic F-127 promotes functional recovery after ventral root avulsion. Tissue Engineering Part A. 2019;25(19–20):1381–1395.