Abstract
Although concentrated growth factor (CGF) is known to promote gingival regeneration and improve the outcomes of clinical treatment, the mechanisms underlying its effects remain unknown. Therefore, this study aimed to elucidate the effects of CGF on gingival thickening. To this end, gingival mesenchymal stem cells (GMSCs) were treated with different concentrations of CGF, and the effects of CGF on cell proliferation and migration; collagen-1 (Col-1), fibronectin (FN), vascular endothelial growth factor (VEGF), and angiopoietin-1 (Ang-1) expression; and the AKT, Wnt/β-catenin, and Yes-associated protein (YAP) signalling pathways were investigated. The effects of CGF in vivo were also investigated in a rat buccal gingival injection model. GMSCs cultured with CGF showed improved cell proliferation and migration. Moreover, CGF treatment improved the levels of FN, Col-1, VEGF, and ANG-1. These effects of CGF were mediated by the AKT/Wnt and YAP pathways, with the AKT pathway possibly functioning upstream of the Wnt/β-catenin and YAP pathways. YAP was also shown to be overexpressed in the in vivo model. Thus, CGF can promote gingival regeneration, and YAP transport into the nucleus may be a key factor underlying this activity, which provides a novel perspective for gingival regeneration and further promotion of the clinical application of CGF.
Introduction
Patients with thin gingiva biotype often experience disappointing outcomes such as gingival recession, poor postoperative stability, and unexpected aesthetic effects during orthodontic and orthognathic treatment. Hence, the gingival graft procedure is the current “gold standard” for the treatment [Citation1]. However, this approach has various drawbacks such as donor-site damage, limited palatal mucosal tissue, and poor postoperative outcome [Citation2–4]. Gingival regeneration is a complex developmental process, which involves the use of growth factors to establish a fully functional vascular microenvironment for tissue survival [Citation5]. A previous study has confirmed that concentrated growth factor (CGF), a third-generation platelet concentrate derived from autologous blood, is crucial for tissue regeneration[Citation6]. Hitherto, the function of CGF has been rarely reported with respect to gingival regeneration.
CGF is obtained by centrifugation of autologous venous blood without any anticoagulant and thrombin additives during blood harvesting. CGF contains a variety of autologous growth factors, including platelet-derived growth factors (PDGF), transforming growth factors-β (TGF-β), vascular endothelial growth factors (VEGF), and insulin-like growth factors (IGF), tumour necrosis factor-α (TNF-α), which are of crucial importance in tissue regeneration [Citation7]. It has been reported that CGF could not only promote the proliferation and osteogenic differentiation of mesenchymal stem cells in vitro but also enable excellent healing of bone defects of critical sizes in vivo [Citation8]. Moreover, a previous study has revealed that CGF regulates the synthesis of the extracellular matrix of auricular chondrocytes and enhances cartilage regeneration in microtia patients via the insulin-like growth factor 1 receptor (IGF-1R)/PI3K/AKT signalling pathway [Citation9]. Interestingly, various types of platelet extracts, including CGF, have been used in preclinical and clinical studies on topics such as teeth implantation, bone fracture healing, and hair replantation [Citation10–12]. However, the potential mechanisms of CGF-mediated gingival regeneration are yet to be elucidated. Moreover, the intricate regulatory networks and complex signalling mechanisms in the CGF microenvironment are of great importance to gingival tissue regeneration, which requires further clarification.
In addition to the classical signalling pathways, the YAP signalling pathway controls cell proliferation, migration, and tissue regeneration in many organs [Citation13–15]. When the signalling pathway is active, the phosphorylation of upstream molecules including MSTs and LATS leads to the degradation of YAP in the cytoplasm, which inhibits its transcriptional activity [Citation16]. Conversely, the inactivation of this signalling pathway increases YAP nuclear accumulation. Subsequently, YAP interacts with many transcription factors in addition to TEAD/TEF family factors to activate the target genes controlling cell proliferation, migration, and apoptosis, and tissue regeneration [Citation17]. An increasing number of studies have highlighted that YAP is the nexus and integrator of multiple prominent signalling cascades with vital roles in the regulation of cell function and tissue regeneration [Citation18,Citation19]. Previous studies have also revealed that YAP interacted with other signalling pathways, including the mechanotransduction, Wnt, G protein-coupled receptor, epidermal growth factor (EGF), and Notch pathways [Citation20–23]. A recent study provided evidence that RASSF1A-induced pS127-YAP plays an active role in controlling the balance between pluripotency and differentiation of stem cells [Citation24]. A previous in vitro study demonstrated that YAP accelerates cell cycle, inhibits apoptosis, and delays senescence in human periodontal ligament stem cells by influencing the members of the mitogen-activated protein kinase (MAPK) and B-cell lymphoma-2 (Bcl-2) families [Citation25]. However, whether the YAP signalling pathway interacts with other signalling pathways involved in the regulation of the proliferation, migration, and differentiation of gingival mesenchymal stem cells (GMSCs) treated with CGF remained largely unclear.
In the present study, we demonstrated that the treatment with CGF membrane could increase the thickness of gingiva and amplify the postoperative stability after periodontal accelerated osteogenic orthodontics (PAOO). Based on these findings and those of previous studies mentioned above, we hypothesised that CGF could promote the proliferation, migration, and differentiation of GMSCs isolated from gingival tissues surrounding the third molar sockets. Furthermore, we found that CGF exerted strong effects on cell proliferation and migration as well as the expression of angiogenic and fibroblast-specific factors in a concentration-dependent manner. Moreover, the activation of the AKT and Wnt/β-catenin signalling pathways and transportation of YAP to the nucleus might be the mechanisms underlying the effects of CGF treatment on GMSCs. Finally, in this study, a gingival buccal injection model was used to investigate the regulatory effect of CGF on gingival regeneration ability in vivo. The results of this study showed the advantages of CGF as a potential source for tissue engineering as it is easily accessible and applicable and a less invasive approach for harvest, which makes it a promising choice for gingival tissue regeneration.
Materials and methods
Periodontal accelerated osteogenic orthodontics
During orthodontic treatment, PAOO was performed in 10 patients who were healthy and had good oral hygiene. These patients received treatment at the Department of Oral and Cranio-maxillofacial Surgery, Ninth People’s Hospital, Shanghai Jiao Tong University School of Medicine. The treatment involved the use of the CGF membrane to increase the alveolar bone mass to accelerate tooth movement (Supplementary Figure 1 and ). The following patients were included: (1) Male or female patients aged 18–35 years; gender is not limited; (2) patients with dental or skeletal type III malocclusion who needed orthodontic compensation treatment or decompensated orthodontic treatment; (3) patients with thin gingiva accompanied by bone cracking and bone opening; and (4) patients with tooth movement who wanted to shorten treatment time and desired increased orthodontic stability. The following patients were excluded: (1) Patients with periodontal disease or severe gingival recession; (2) patients with abnormal blood and coagulation functions; (3) patients allergic to implants; (4) patients with long-term use of corticosteroids and bisphosphonates; (5) patients with long-term use of non-steroidal anti-inflammatory drugs; (6) patients with diseases of the heart, lung, brain, kidney, and other important organs; and (7) patients with mental illness. This study was approved by the Ethics Committee of Shanghai Ninth People’s Hospital, Shanghai Jiao Tong University School of Medicine (SH9H-2018-164-T122).
Collection, culture, and identification of GMSCs
Gingival tissues surrounding the third molar sockets from four 16- to 20-year-old healthy individuals were collected immediately after tooth extraction for gingiva-derived cell isolation, culture, and subsequent investigations. Informed consent was obtained from all individuals for the use of their gingival tissues. Briefly, the gingival tissues were rinsed three times with phosphate-buffered saline (PBS) containing 5% penicillin/streptomycin (Invitrogen, Carlsbad, CA, USA). Then, the gingival tissues were physically minced into <1 mm3 pieces and fixed with a coverslip in 10-cm2 dishes and cultured with Dulbecco’s modified Eagle’s medium (DMEM; Gibco, Carlsbad, CA, USA) containing 20% foetal bovine serum (FBS; Gibco, Carlsbad, CA, USA) and 1% penicillin/streptomycin. All primary cultured cells were subjected to immunofluorescence to observe the expression of cytokeratin and vimentin. To prove the ability of multipotent differentiation, human GMSCs were cultured in osteogenic, adipogenic, and chondrogenic media (Cyagen, Santa Clara, CA, USA). Both media were refreshed at 3-day intervals. After incubation for 10 days, the expression of the osteogenic, adipogenic, and chondrogenic genes was analysed by quantitative real-time PCR (qRT-PCR). The primer sequences are listed in Supplementary Table 1. After incubation for 7 days, the cells were subjected to alkaline phosphatase (ALP) staining. After incubation for 14 days, the cells were subjected to Alican blue and toluidine blue staining. After incubation for 3 and 4 weeks, the cells were subjected to Alizarin Red and Oil O staining, respectively.
Preparation of the CGF extract
An equivalent of 36 ml of venous blood was withdrawn into four sterile vacuette tubes by using a 24-gauge needle from three healthy male volunteers (age range: 18–25 years) who provided informed consent. Then, the four tubes were subjected to centrifugation in a Medifuge 2000 centrifuge (Silfradent, London, UK) by acceleration for 30 s and centrifugation for 2 min at 2700 rpm, for 4 min at 2400 rpm, for 4 min at 2700 rpm, for 3 min at 3000 rpm, and finally deceleration for 36 s. After centrifugation, three separate fractions were obtained in the tubes: (1) an uppermost blood plasma fraction without fibrinogen and coagulation factors; (2) a CGF layer containing various kinds of growth factors; and (3) the lowermost red blood cell layer. Every CGF layer was extracted carefully and diluted with DMEM separately according to volume for subsequent experiments.
Live and dead cell assay
To investigate the biocompatibility of CGF, GMSCs treated with different concentrations of CGF were evaluated using a live-dead staining assay. Briefly, the live cells were stained with 2 µmol/L Calcein-AM (Dojindo, Kumamoto, Japan), and dead cells were stained with 4 µmol/L propidium iodide (Sigma-Aldrich, St Louis, Mo, USA) . The images were captured using a fluorescence microscope (Olympus Co., Tokyo, Japan).
Cell proliferation assay
To determine the optimal concentrations of CGF for the subsequent experiments, DMEM containing different concentrations of CGF was used. The GMSCs were seeded in 96-well plates at a density of 3 × 103 cells/well in triplicate. After cell attachment, the culture medium was replaced by the medium supplemented with various concentrations of CGF. Then, a cell counting kit-8 (CCK-8, Kumamoto, Japan) was used to detect the ability of proliferation on days 1, 4, and 7. Absorbance was measured using a microplate reader at 450 nm.
Cell migration assay
Twenty-four-well plates containing Transwell filter inserts (Corning Inc., Corning, NY, USA) were used to detect the migratory capacity of GMSCs after treatment with CGF. Briefly, 1 × 105 cells harvested in 200 μL of serum-free culture medium were seeded in the upper chambers. The lower chambers consisted of 700 μL medium/well with different concentrations of CGF in 24-well plates. Thirty-six hours after culture, the cells were fixed and stained with 5% crystal violet. Then, the migrated cells were photographed and counted using an inverted microscope (Olympus IX71, Japan) by two observers.
qRT-PCR
The expression of fibronectin (FN), collagen-1 (Col-1), vascular endothelial growth factor (VEGF), and angiopoietin-1 (Ang-1) messenger RNAs (mRNAs) was determined by qRT-PCR. Briefly, total cellular RNA was extracted with TRIzol reagent (Invitrogen, Carlsbad, CA, USA), and cDNA was synthesised from total RNA by using the PrimeScript RT reagent kit (Takara Bio, Inc., Shiga, Japan) according to the manufacturer’s instructions. The qRT-PCR analysis was performed using the Bio-Rad real-time PCR system (Bio-Rad, USA). The qRT-PCR programme was as follows: 40 cycles each involving 5 s of denaturation at 95 °C and 34 s of amplification at 60 °C. All PCR amplifications were performed in triplicate. The primer sequences for GMSCs are listed in Supplementary Table 1.
Western blot
Protein concentration was measured using the Pierce™ BCA Protein Assay kit (Thermo Scientific, USA). Total protein (20 μg) was transferred to polyvinylidene difluoride (PVDF) membranes (0.22 μm, Merck Millipore, USA). The membranes were blocked with 5% non-fat milk for 1 h, followed by incubation with primary antibodies overnight at 4 °C and with horseradish peroxidase (HRP)-linked secondary antibodies for 1 h at room temperature. All protein expression was normalised to that of the internal control. The protein bands were then visualised using the enhanced chemiluminescence (ECL) substrate kit (Merck Millipore, USA) and quantified using ImageJ software.
Immunofluorescence staining
After fixation and permeabilization following respective treatments, the slides were subsequently blocked and incubated with primary antibodies overnight. Then, the slides were incubated with the secondary antibodies in the dark for 1 h at room temperature. 4′,6-Diamidino-2-phenylindole (DAPI) was used to stain the cell nuclei. Finally, the slides were photographed, and the images were analysed using a fluorescence microscope.
Signalling pathway inhibition
LY294002 and CA3, which are highly selective inhibitors of AKT and YAP respectively, were purchased from Selleck (China) and dissolved in dimethyl sulfoxide (DMSO) at a stock concentration of 10 mM according to the manufacturer’s protocol. To confirm the involvement of the AKT and YAP pathways in CGF-mediated regeneration, GMSCs were pre-treated with the recommended concentrations of LY294002 (10 μM) and CA3 (1 μM) for 60 min and subsequently stimulated with CGF for another 60 min. The western blotting assay, Transwell assay, and immunofluorescence staining were then conducted as described above.
Transfection assay
According to the manufacturer’s protocol, an equivalent of 1 × 105 GMSC cells/well were plated in 6-well plates at passage 3 and cultured to 70% confluency. Then, the cells were transfected with YAP-overexpressing plasmids packaged using Lipofectamine™ 3000 transfection reagent (Invitrogen, CA). The cells transfected with empty plasmids were used as controls. Meanwhile, GMSCs at 60%–70% confluence were transfected with YAP siRNA or the negative control by using Opti-MEM (Invitrogen, CA) medium according to the manufacturer’s instructions. All the plasmids, siRNA, and the negative control were purchased from RiboBio (Guangzhou, China). The transfection efficiency of YAP was measured by qPCR and western blot. The CCK-8 and Transwell assays were performed to investigate the function of YAP in promoting the proliferation and migration of GMSCs. Moreover, the mRNA and protein expression of FN, Col-1, VEGF, and Ang-1 for each group was detected by qRT-PCR and western blot, respectively. All experiments were repeated three times.
Animal study design
Ten male Sprague–Dawley (SD) rats were obtained from the Shanghai Jiao Tong University School of Medicine Laboratory Animal Centre. The blood of four SD rats was collected by aorta ventralis puncture, without anticoagulants, and subjected to centrifugation for 13 min. The other rats were randomly divided into two groups to establish the buccal gingival injection model (Supplementary Figure 5). Briefly, these rats were anaesthetised by intraperitoneal injection of pentobarbital, and then, vascular forceps and tweezers were used to expose the buccal visual field of mandibular molars. Subsequently, 300 µL of CGF was injected into the submucosal layer of mandibular gingiva in the sagittal direction of the alveolar bone by using articaine adrenal injective syringe. The other side of the gingiva was injected with the same amount of PBS as that injected in the control group. This experimental treatment was performed once a week for 4 weeks. After another 4 weeks, the animals were sacrificed, and the mandibular gingiva was subjected to gross examination, hematoxylin-eosin (HE) staining, and histology.
HE staining and immunohistochemistry
The gingival tissue of the rats in each group was harvested, fixed in formalin, embedded in paraffin, and cut into 4-µm slices for HE staining and immunohistochemistry. Briefly, the sections were incubated with YAP, Ki67, and CD31 antibodies overnight at 4 °C following deparaffinization, hydration, and endogenous peroxidase blocking. The mean thickness of the gingival epithelium was calculated from the thickness of the basal layer to the granule of the gingival mucosa. Microscopic examination of the tissue samples subjected to immunohistochemical staining was performed by two persons.
Statistical analysis
All data are expressed as mean ± standard deviation values. Differences between two groups were evaluated by two-tailed Student’s t-test using SPSS 17.0 software (SPSS Inc., USA). All experiments were performed at least three times, and p < .05 was considered statistically significant.
Results
Clinical observation of gingival thickness after PAOO
The average gingival thickness at the cemento-enamel junction of six anterior teeth was measured by cone-beam computed tomography (CBCT). Incidentally, CBCT at 6 months postoperatively demonstrated a significant increase in the gingival thickness when the CGF membrane was used during surgery (). Detailed information about the age, sex and preoperative and postoperative gingival thickness of 10 patients is shown in Supplementary Table 2. These results prompted us to isolate and culture GMSCs from gingival tissues and explore the underlying mechanisms.
Characterisation of human GMSCs obtained from gingival tissue
GMSCs have specific characteristics of self-renewal and multi- or pluripotency. In addition, they can be induced to differentiate into osteogenic, chondrogenic, and adipogenic lineages. Extensive studies have demonstrated the regenerative capability of MSCs both in vitro and in vivo [Citation26,Citation27]. Therefore, GMSCs are a promising alternative cell source for tissue regeneration. In our study, the first adherent cells displayed a spindle-shaped, fibroblast-like morphology between 4 and 7 days after tissue preparation. Typically, primary cell cultures are obtained over 12–15 days at 70–90% confluence (). In immunofluorescence analyses, all primary cultured cells expressed vimentin, while no cytokeratin expression was detected (). When the cells were cultured in an osteoinductive medium, ALP staining revealed mineralisation at 7 days. GMSCs also formed mineralised nodules after osteogenic induction for 21 days, and lipid-rich vacuoles within cells were detected by Oil Red O staining. Moreover, Alican blue and toluidine blue staining demonstrated that GMSCs could be induced to undergo chondrogenic differentiation (), and qPCR analyses suggested that the expression levels of osteopontin (OPN), runt-related transcription factor 2 (RUNX2), Col-1, peroxisome proliferator-activated receptor, collagen-2 (Col-2), and aggrecan (ACAN) were higher than those in the control group in GMSCs following 10 days of osteogenic, adipogenic, and chondrogenic induction (). These data indicate that cells derived from gingival tissues possess the ability to show adherent growth, self-renewal, and multipotent differentiation.
Figure 2. Characterisation of human GMSCs obtained from gingival tissue. (A) Representative images of primary cells grown from human gingival tissue and at days 7, 11, and 15. Scale bars: 100 μm. (B) Representative images of gingival tissue primary cells subjected to immunofluorescence staining. Cells were negative for cytokeratin and positive for vimentin (green) expression; nuclei were stained with DAPI (blue). Scale bars: 100 μm. (C) Representative images of cells cultured with normal and inductive medium and stained with ALP, Alizarin Red S, Oil Red O, alican blue and toluidine blue. Scale bars: 100 μm. (D) qRT-PCR analysis for the expression levels of Col-1, Runx2, OPN,PPARγ, Col-2 and ACAN treated with normal and inductive medium, respectively. Scale bars: 100 μm. *p < .05.
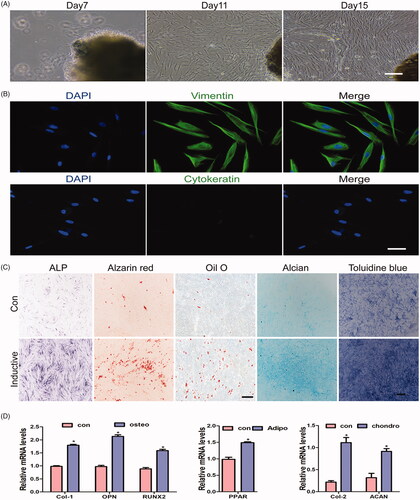
CGF promotes proliferation, migration and differentiation of GMSCs in vitro
The live and dead assay found >90% viable cells irrespective of the CGF concentration (). Therefore, CGF treatment was associated with excellent cell viability and biocompatibility in the present culture conditions. GMSC proliferation after treatment with different concentrations of CGF was higher than that in the control group at every time point (). Importantly, the results demonstrated that the proliferation ability was elevated when the cells were cultured with 4%, 8%, and 10% CGF at days 4 and 7. However, no significant difference was detected among 4%, 8%, and 10% CGF. After culturing for 36 h, CGF can promote the migration of GMSCs in a concentration-dependent manner (). Furthermore, the expression of FN, Col-1, VEGF, and ANG-1 was upregulated after incubation in CGF for 24 h, as detected by the qRT-PCR assay (Supplementary Figure 2). In addition, western blot demonstrated that CGF enhances the expression of FN, Col-1, ANG-1, and VEGF proteins in comparison with their expression levels in normal medium alone (). Interestingly, the expression of VEGF protein was maximal when the cells were cultured in 4% CGF, while the expression of other proteins increased in a concentration-dependent manner. Consequently, 10% of CGF was chosen as the appropriate concentration for immunofluorescence staining, which demonstrated markedly increased cytoplasmic expression of FN and Col-1 (). These data suggested that CGF promotes proliferation, migration, and differentiation of GMSCs, which is essential for gingival regeneration.
Figure 3. CGF promotes proliferation, migration, and differentiation of GMSCs. (A) Live/dead staining assay for GMSCs cultured in different concentrations of CGF. Scale bars: 100 μm. (B) CCK8 assay for GMSCs cultured in different concentrations of CGF at days 1, 4, and 7. (C) Transwell assay for GMSCs cultured in different concentrations of CGF. Scale bars: 100 μm. (D) Western blot assay to assess the expression of Col-1, FN, VEGF, and ANG-1 proteins in GMSCs treated with different concentrations of CGF at 48 h. (E) Representative images of GMSCs treated with different concentration of CGF were subject to immunofluorescence to assess the expression of FN and Col-1. Scale bars: 100 μm. *p < .05; #p < .01.
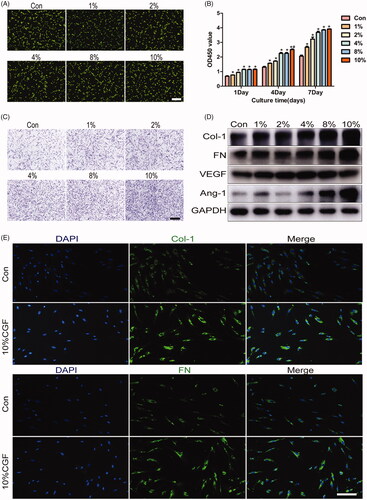
CGF promotes gingival regeneration via activation of AKT/Wnt and YAP signalling pathways
The AKT/Wnt and YAP pathways are common pathways in the process of tissue regeneration [Citation28–30]. Our study aimed to explore whether the AKT/Wnt and YAP signalling pathways are involved in regulating the proliferation, migration, and differentiation of GMSCs treated with CGF. To explore the potential mechanisms by which CGF regulates the biological processes of GMSCs, we examined the signalling pathways regulated by CGF treatment. The findings showed found that AKT and GSK3β were phosphorylated at 5 min, followed by a decrease after 30 min. However, the expression of cytoplasmic β-catenin was not changed significantly by CGF (). Moreover, the expression of p-YAP (ser127) and p-YAP (ser397) was significantly suppressed by CGF. Furthermore, western blot analysis of the nuclear protein levels demonstrated that CGF could drastically induce the nuclear translocation of YAP in a time-dependent manner (). To further elucidate the cellular localisation of YAP and β-catenin, immunofluorescence staining analysis was performed, which confirmed that β-catenin rarely entered the cell nucleus, and YAP was largely transferred to the cell nucleus, and the nuclear YAP transport in the CGF groups was significantly different from that in the control group (Supplementary Figure 3(A,B)). To further examine the crosstalk of AKT/Wnt/β-catenin and YAP pathways in CGF-mediated gingival regeneration, the inhibitors LY294002 and CA3, a potent inhibitor of YAP, were used, respectively. The results of western blot analyses revealed that AKT phosphorylation for GMSCs treated with CGF could be attenuated by LY294002. Moreover, the expression level of phosphorylated YAP (ser397) was significantly enhanced by CGF treatment with CA3 (). The AKT inhibitor LY294002 also inhibited the phosphorylation of GSK3β and increased the expression level of p-YAP protein (ser397) in the cytoplasm. However, CA3 could not influence the expression of the other two pathways. These results demonstrated that the Wnt/β-catenin, AKT, and YAP pathways effectuate crosstalk in CGF-mediated gingival regeneration, and the AKT pathway may function as upstream of the Wnt/β-catenin and YAP pathways. In addition, the results demonstrated that LY294002 and CA3 could suppress the upregulated expression of FN, Col-1, Ang-1, and VEGF protein (). Moreover, the upregulated migration capability was partially inhibited by these two pathway inhibitors (). Furthermore, immunofluorescence staining could verify that LY294002 and CA3 reduced the levels of FN and Col-1 in GMSCs in comparison with the control group (Supplementary Figure 3(A,B)). The results also clearly demonstrated that the expression of FN and Col-1 was suppressed in GMSCs treated with CGF ().
Figure 4. CGF promotes gingival regeneration via activation of AKT/Wnt and YAP signalling pathways. (A) Western blot assay for expression of AKT and Wnt signalling pathways for GMSCs treated with different concentrations of CGF at 5, 15, 30, 60, and 90 min. (B) Western blot assay for the expression of YAP and p-YAP proteins in cytoplasm and YAP in cell nucleus for GMSCs treated with different concentrations of CGF at 5, 15, 30, 60, and 90 min. (C) Western blot assay for the expression of p-AKT, p-GSK3β, β-catenin, and p-YAP (ser397) for GMSCs cultured in CGF with inhibitors LY294002 and CA3 for 15 min, respectively. (D) Western blot assay for the expression of FN, Col-1, VEGF, and ANG-1 for GMSCs cultured in CGF with inhibitors LY294002 and CA3 for 48 h, respectively. (E) The Transwell assay for GMSCs cultured in CGF with inhibitors LY294002 and CA3. Scale bars: 100 μm. (F) Representative images of GMSCs cultured in CGF with inhibitors LY294002 and CA3 were subjected to immunofluorescence to assess the expression of FN and Col-1. Scale bars: 100 μm.
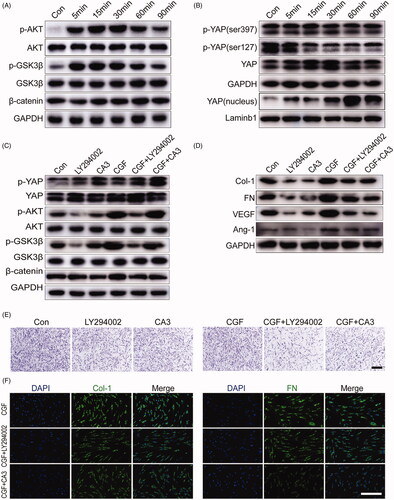
YAP promotes proliferation, migration, and differentiation in GMSCs
In order to further examine the regulatory mechanism of YAP in the process of gingival regeneration, YAP overexpression plasmids were transfected into GMSCs. qPCR and western blot confirmed an increase in the expression level of YAP. Moreover, high mRNA and protein expression levels of FN, Col-1, VEGF, and Ang-1 were detected for YAP-overexpressed GMSCs in comparison with the control group (). Importantly, the cell proliferation and Transwell assays demonstrated that YAP regulates the proliferation and migration abilities of the cells (). Moreover, YAP siRNA and negative control were transfected to verify the effect of YAP on cell proliferation, migration, and differentiation in GMSCs. The results showed that after YAP siRNA transfection, the expression level of YAP was significantly decreased in GMSCs compared to those transfected with the corresponding siRNA control (Supplementary Figure 4(A,B)). Likewise, the mRNA and protein expressions of FN, Col-1, VEGF, and Ang-1 were also obviously downregulated (Supplementary Figure 4(A,B)). The proliferation and Transwell assays revealed that the proliferation and migration abilities of GMSCs treated with YAP siRNA was markedly attenuated in comparison to those transfected with siRNA control (Supplementary Figure 4(C,D)). Taken together, the above data indicated that YAP is one of the major nexuses of upstream and downstream factors that are required for gingival regeneration.
Figure 5. YAP promotes proliferation, migration, and differentiation in GMSCs. (A) qRT-PCR analysis for the expression levels of YAP, Col-1, FN, VEGF, and ANG-1 in GMSCs treated with YAP control and overexpression plasmids. (B) Western blot assay for the expression of Col-1, FN, VEGF, and ANG-1 proteins for GMSCs treated with YAP control and overexpression plasmids. (C) CCK8 assay for GMSCs treated with YAP control and overexpression plasmids at 2, 44, 872 h. (D) Transwell assay for GMSCs treated with YAP control and overexpression plasmids. Scale bars: 100 μm. *p < .05; **p < .01.
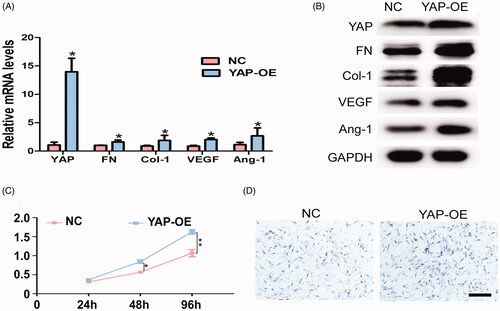
YAP promotes gingival regeneration in CGF microenvironment in vivo
In order to further examine the regenerative capacity of CGF in vivo, gingival buccal injections were administered to rats for four consecutive weeks (Supplementary Figure 5). Subsequently, HE staining showed that the number of layers in the spinous and granule of the SD rats in the CGF group is greater than that in the PBS group. Moreover, the mean thickness of the basal layer to the granule of the gingival mucosa and lamina propria in the CGF group was significantly higher than that in the control group. In addition, the basal layer of the PBS group was relatively flat, while the epithelial spikes in the CGF group were significantly elongated (). The images obtained after immunohistochemical staining also revealed high expression levels of YAP in the nucleus in the CGF group. In addition, the Ki67, a proliferation marker confined to the basal layer, was highly expressed in the CGF microenvironment. The expression level of the endothelial cell marker, CD31, was remarkably upregulated in the tissues in the CGF group as compared to the control group (). These findings suggest that CGF might promote the transport of YAP into the nucleus and improve the microcirculation state, which in turn provides adequate blood supply and sufficient nutrients to achieve the goal of promoting cell proliferation, collagen deposition, and thickening of the gingival tissue.
Figure 6. YAP promotes gingival regeneration in CGF microenvironment in vivo. (A) Representative images of HE staining showed that the thickness in the CGF group was higher than that in the PBS group. Scale bar: 100 μm. (B, C, D): Representative images of immunohistochemistry staining indicated that the expression level of angiogenic marker (CD31), proliferation marker (Ki67), and transcription coactivator (YAP) in the CGF group was much higher as compared to the PBS group. Scale bar: 100 μm. *p < .05; **p < .01.
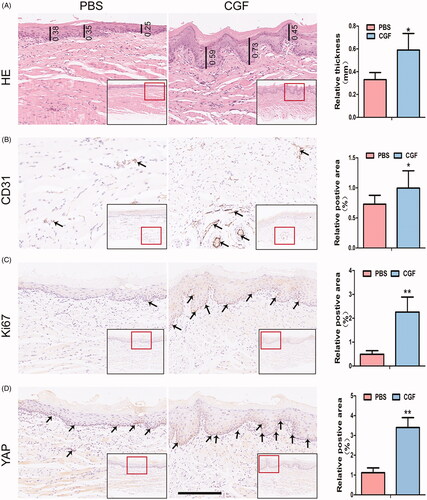
Discussion
Mucogingival tissue deformities are a group of conditions characterised by inadequate thickness of keratinised gingival tissue, which could result in gingival recession, unsatisfactory aesthetics, and poor chewing function [Citation31–35]. Previous studies have demonstrated that an autogenous palatal mucosal graft was the most predictable grafting material to facilitate keratinised gingival repair [Citation36]. However, this approach could lead to secondary damage in the donor site and other drawbacks. Therefore, development of novel strategies for augmentation of gingival width and thickness is an urgent prerequisite. Over the last decade, cell-based therapeutics and tissue engineering applications have gained attention for the treatment of periodontal diseases in both fundamental and clinical studies [Citation37–41]. Intriguingly, GMSCs treated with human platelet lysate showed increased proliferation ability and osteogenic/odontogenic differentiation potential in both in vitro and in vivo studies, which supports the use of platelet lysate as an effective alternative in future studies [Citation42–46]. Furthermore, during follow-up assessments of patients who received PAOO, we observed that the CGF membrane obtained from autologous blood increased the gingival thickness and maintained postoperative stability. Hence, these results prompted us to isolate MSCs from human gingival tissue and culture them in the presence of CGF to examine the potential mechanisms.
Autologous platelet concentrates containing different growth factors play an essential role in tissue regeneration [Citation47]. In this study we selected 10% concentration for experiments because of the existence of optimum doses of CGF based on the results of pre-experiments and references. To the best of our knowledge, this study, for the first time, investigated the safety and efficiency of CGF by evaluating cell proliferation, migration, and differentiation and assessed the potential mechanisms underlying gingival regeneration in vitro and in vivo. The present study demonstrated that CGF enhances the proliferation and migration ability and the expression levels of pro-angiogenic and collagen-related factors in GMSCs. Angiogenesis plays a pivotal role in the process of gingival development and repair, and it occurs before the accumulation of collagen and fibronectin and provides abundant blood supply and nutrients for gingival regeneration [Citation48]. VEGF and Ang-1 are fundamental angiogenic factors that exist predominantly in MSCs, which is a prerequisite for neovascularization and soft tissue formation [Citation49,Citation50]. Moreover, the present study indicated that CGF enhances the expression of VEGF and ANG-1 in GMSCs in a concentration-dependent manner, which might increase the accumulation of Col-1 and FN and facilitate gingival tissue repair in patients with a thin gingival biotype. Based on these results, it is suggested that CGF not only promotes the proliferation and migration of GMSCs but also enhances the expression of pro-angiogenic and collagen-related proteins in vitro, making it a prerequisite for gingival tissue repair and regeneration.
In addition to the AKT and Wnt/β-catenin signalling pathways, YAP nuclear accumulation might play a key role in the physiology and pathophysiology of GMSCs, which could exert a profound effect on diverse processes, including cell proliferation, migration, metabolism, and differentiation [Citation51–53]. Importantly, a previous study demonstrated that YAP overexpression promotes proliferation, inhibits apoptosis, and delays senescence of h-PDLSCs by influencing the ERK and Bcl-2 signalling pathways. However, the detailed mechanisms involved in gingival tissue regeneration in the CGF microenvironment remain to be explored. Therefore, we hypothesised that the AKT, Wnt/β-catenin, and YAP signalling pathways might be involved in gingival tissue regeneration. Hitherto, the correlation between these three signalling pathways in GMSCs was not investigated. Our results confirmed that CGF could activate the AKT and Wnt/β-catenin signalling pathway. Notably, the nuclear translocation of YAP was increased when stimulated by CGF in comparison with the control group. In our previous study, we had demonstrated that the AKT signalling pathway functioned upstream of the Wnt/β-catenin signalling pathway in vascularised bone regeneration [Citation54]. Hence, in the present study, we considered that these two pathways form an organic entirety in CGF-mediated gingival regeneration. Interestingly, the current data also suggested that the AKT signalling pathway may function upstream of the Wnt/β-catenin and YAP signalling pathways. However, the association between β-catenin and YAP in the nucleus and cytoplasm of GMSCs treated with CGF required further clarification. Moreover, based on the literature and existing results, whether upstream and downstream signalling pathways other than the Akt, Wnt/β-catenin, and YAP signalling pathways are involved in cell proliferation, migration, and differentiation, and consequently affect gingival tissue regeneration needs to be further investigated.
Recently, CGF gelatum and liquid CGF were widely used in medical cosmetology due to their safety and convenience [Citation55]. A previous study also confirmed that platelet concentrates could significantly increase the release of chemical products, as well as facilitate blood vessel ingrowth and vascularised regeneration [Citation56]. Interestingly, the current study confirmed that CGF injected into buccal gingival mucosa could increase gingival epithelial thickness as well, which needs to be studied further in vitro. Immunohistochemical results indicated that CGF promotes the transport of YAP into the nucleus and enhances the expression of proliferation marker (Ki67) and endothelial cell marker (CD31), which might contribute to creating a pro-angiogenic biochemical microenvironment for promoting cell proliferation, collagen deposition, and thickening of gingival tissue. Taken together, these results confirm that CGF effectively promotes gingival tissue regeneration in vivo.
Therefore, the present study demonstrated that the CGF membrane increases gingival thickness during PAOO. Subsequently, based on these studies, we plan to conduct a prospective randomised controlled trial to further investigate the effect of CGF on periodontal soft and hard tissue during PAOO. Accordingly, in vitro data indicated that CGF directly enhances the regenerative ability of GMSCs by promoting cell proliferation, migration, and the expression of angiogenic-related and fibroblast-related genes. These stimulatory effects might be attributed to the activation of AKT, Wnt/β-catenin, and YAP signalling pathways, with the AKT signalling pathway functioning upstream to the Wnt/β-catenin and YAP signalling pathways. Importantly, the in vitro and in vivo results confirmed that CGF markedly induces the transport of YAP into the nucleus, which might provide a new perspective for gingival regeneration and further promotion of the clinical application of CGF. shows the schematic of the detailed mechanisms involved in CGF-mediated regeneration of GMSCs.
Conclusion
In summary, the present study demonstrated that CGF has great potential to promote gingival tissue regeneration. CGF directly promotes the proliferation and migration of GMSCs in vitro and enhances microcirculation in vivo. Remarkably, the transport of YAP into the nucleus was proposed as a key mechanism underlying the effects of CGF. However, these experiments need to be repeated to further examine the in-depth mechanisms of CGF in gingival tissue regeneration, and large-sample animal studies are essential to substantiate the complex networks involved in these mechanisms. The current findings may provide novel insights into the mechanisms underlying gingival regeneration in the CGF microenvironment. Thus, CGF may be considered as a bioactive material for soft and hard vascularised regeneration, thereby lending a new perspective for regenerative medicine and further promoting the clinical application of CGF.
Supplemental Material
Download TIFF Image (389.3 KB)Supplemental Material
Download TIFF Image (845.2 KB)Supplemental Material
Download TIFF Image (1.3 MB)Supplemental Material
Download TIFF Image (2.3 MB)supplementary_table_2.docx
Download MS Word (13.4 KB)supplementary_table1.docx
Download MS Word (16 KB)Disclosure statement
The authors declare no competing financial interests exist.
Additional information
Funding
References
- Kloukos D, Koukos G, Doulis I, et al. Gingival thickness assessment at the mandibular incisors with four methods: a cross-sectional study. J Periodontol. 2018;89(11):1300–1309.
- Chiu GS, Chang CH, Roberts WE. Bimaxillary protrusion with an atrophic alveolar defect: orthodontics, autogenous chin-block graft, soft tissue augmentation, and an implant. Am J Orthod Dentofacial Orthop. 2015;147(1):97–113.
- Urban IA, Nagy K, Werner S, et al. Evaluation of the combination of strip gingival grafts and a xenogeneic collagen matrix for the treatment of severe mucogingival defects: a human histologic study. Int J Periodontics Restorative Dent. 2019;39(1):9–14.
- Yaghobee S, Rouzmeh N, Aslroosta H, et al. Effect of Topical Erythropoietin (EPO) on palatal wound healing subsequent to Free Gingival Grafting (FGG). Braz Oral Res. 2018;32:55.
- Grawish ME. Gingival-derived mesenchymal stem cells: an endless resource for regenerative dentistry. World J Stem Cells. 2018;10(9):116–118.
- Yu M, Wang X, Liu Y, et al. Correction to: cytokine release kinetics of concentrated growth factors in different scaffolds. Clin Oral Invest. 2019;23(4):1999–1999.
- Hong S, Li L, Cai W, et al. The potential application of concentrated growth factor in regenerative endodontics. Int Endod J. 2019;52(5):646–655.
- Honda H, Tamai N, Naka N, et al. Bone tissue engineering with bone marrow-derived stromal cells integrated with concentrated growth factor in Rattus norvegicus calvaria defect model. J Artif Organs. 2013;16(3):305–315.
- Chen X, Zhang R, Zhang Q, et al. Microtia patients: auricular chondrocyte ECM is promoted by CGF through IGF-1 activation of the IGF-1R/PI3K/AKT pathway. J Cell Physiol. 2018;10:88–98.
- Hong S, Chen W, Jiang B. A comparative evaluation of concentrated growth factor and platelet-rich fibrin on the proliferation, migration, and differentiation of human stem cells of the apical papilla. J Endod. 2018;44(6):977–983.
- Borsani E, Bonazza V, Buffoli B, et al. Beneficial effects of concentrated growth factors and resveratrol on human osteoblasts in vitro treated with bisphosphonates. Biomed Res Int. 2018;2018:4597321.
- Femminella B, Iaconi MC, Di Tullio M, et al. Clinical comparison of platelet-rich fibrin and a gelatin sponge in the management of palatal wounds after epithelialized free gingival graft harvest: a randomized clinical trial. Periodontol. 2016;87(2):103–113.
- Ardestani A, Lupse B, Maedler K. Hippo signaling: key emerging pathway in cellular and whole-body metabolism. Trends Endocrinol Metab. 2018;29(7):492–509.
- Fu V, Plouffe SW, Guan KL. The Hippo pathway in organ development, homeostasis, and regeneration. Curr Opin Cell Biol. 2017;49:99–107.
- Hong L, Li Y, Liu Q, et al. The Hippo signaling pathway in regenerative medicine. Methods Mol Biol. 2019;1893:353–370.
- Wang J, Liu S, Heallen T, et al. The Hippo pathway in the heart: pivotal roles in development, disease, and regeneration. Nat Rev Cardiol. 2018;15(11):672–684.
- Maugeri-Saccà M, De Maria R. The Hippo pathway in normal development and cancer. Pharmacol Ther. 2018;186:60–72.
- Totaro A, Panciera T, Piccolo S. YAP/TAZ upstream signals and downstream responses. Nat Cell Biol. 2018;20(8):888–899.
- Deng Y, Wu A, Li P, et al. Yap1 regulates multiple steps of chondrocyte differentiation during skeletal development and bone repair. Cell Rep. 2016;14(9):2224–2237.
- Pan JX, Xiong L, Zhao K, et al. YAP promotes osteogenesis and suppresses adipogenic differentiation by regulating β-catenin signaling. Bone Res. 2018;6:18.
- Gouveia RM, Vajda F, Wibowo JA, et al. YAP, ΔNp63, and β-catenin signaling pathways are involved in the modulation of corneal epithelial stem cell phenotype induced by substrate stiffness. Cells. 2019;8(4):347–320.
- Cortes E, Lachowski D, Rice A, et al. Tamoxifen mechanically deactivates hepatic stellate cells via the G protein-coupled estrogen receptor. Oncogene. 2019;38(16):2910–2922.
- Totaro A, Castellan M, Di Biagio D, et al. Crosstalk between YAP/TAZ and notch signaling. Trends Cell Biol. 2018;28(7):560–573.
- Pefani DE, Pankova D, Abraham AG, et al. TGF-β targets the Hippo pathway scaffold RASSF1A to facilitate YAP/SMAD2 nuclear translocation. Mol Cell. 2016;63(1):156–166.
- Jia L, Gu W, Zhang Y, et al. Activated Yes-associated protein accelerates cell cycle, inhibits apoptosis, and delays senescence in human periodontal ligament stem cells. Int J Med Sci. 2018;15(11):1241–1250.
- Xu X, Chen C, Akiyama K, et al. Gingivae contain neural-crest- and mesoderm-derived mesenchymal stem cells. J Dent Res. 2013;92(9):825–832.
- Zhao NB, Wu Z, Qin L, et al. Characteristics and tissue regeneration properties of gingiva-derived mesenchymal stem cells. Crit Rev Eukaryot Gene Expr. 2015;25(2):135–144.
- Han NN, Jia L, Su YY, et al. Lactobacillus reuteri extracts promoted wound healing via PI3K/AKT/β-catenin/TGFβ1 pathway. Stem Cell Res Ther. 2019;10(1):243.
- Kang W, Jia Z, Tang D, et al. Fusobacterium nucleatum facilitates apoptosis, ROS generation, and inflammatory cytokine production by activating AKT/MAPK and NF-B signaling pathways in human gingival fibroblasts. Oxid Med Cell Longev. 2019;2019:1–22.
- Mugahid D, Kalocsay M, Liu XL, et al. YAP regulates cell size and growth dynamics via non-cell autonomous mediators. Elife. 2020;9:e53404.
- Sala L, Alonso-Pérez R, Agustin-Panadero R, et al. Comparative study of two methods for gingival biotype assessment. J Clin Exp Dent. 2018;10:0–863.
- García-Cortés JO, Loyola-Rodríguez JP, Monárrez-Espino J. Gingival biotypes in Mexican students aged 17-19 years old and their associated anatomic structures, socio-demographic and dietary factors. J Oral Sci. 2019;61(1):156–163.
- Cortellini P, Bissada NF. Mucogingival conditions in the natural dentition: narrative review, case definitions, and diagnostic considerations. J Periodontol. 2018;20:204–213.
- Kim SH, Lee JB, Kim MJ, et al. Combining virtual model and cone beam computed tomography to assess periodontal changes after anterior tooth movement. BMC Oral Health. 2018;18(1):180.
- González MM, Araujo-Monsalvo VM, Murayama N, et al. Mandibular anterior intrusion using miniscrews for skeletal anchorage: a 3-dimensional finite element analysis. Am J Orthod Dentofacial Orthop. 2018;154(4):469–476.
- Isler SC, Eraydin N, Akkale H, et al. Oral flurbiprofen spray for mucosal graft harvesting at the palatal area: a randomized placebo-controlled study. J Periodontol. 2018;89(10):1174–1183.
- Sanz AR, Carrión FS, Chaparro AP. Mesenchymal stem cells from the oral cavity and their potential value in tissue engineering. Periodontol 2000. 2015;67(1):251–267.
- Regmi S, Pathak S, Kim JO, et al. Mesenchymal stem cell therapy for the treatment of inflammatory diseases: challenges, opportunities, and future perspectives. Eur J Cell Biol. 2019;33:14–23.
- Li P, Gong Z, Shultz LD, et al. Mesenchymal stem cells: from regeneration to cancer. Pharmacol Ther. 2019;23:32–43.
- Massee M, Chinn K, Lim JJ, et al. Type I and II diabetic adipose-derived stem cells respond in vitro to dehydrated human amnion/chorion membrane allograft treatment by increasing proliferation, migration, and altering cytokine secretion. Adv Wound Care (New Rochelle). 2016;5(2):43–54.
- Zhang LX, Yin YM, Zhang ZQ, et al. Grafted bone marrow stromal cells: a contributor to glial repair after spinal cord injury. Neuroscientist. 2015;21(3):277–289.
- Huang F, Liu ZM, Zheng SG. Updates on GMSCs treatment for autoimmune diseases. Curr Stem Cell Res Ther. 2018;13(5):345–349.
- Moshaverinia A, Xu X, Chen C, et al. Application of stem cells derived from the periodontal ligament or gingival tissue sources for tendon tissue regeneration. Biomaterials. 2014;35(9):2642–2650.
- Yang H, Gao L-N, An Y, et al. Comparison of mesenchymal stem cells derived from gingival tissue and periodontal ligament in different incubation conditions. Biomaterials. 2013;34(29):7033–7047.
- Chen X, Chen YH, Hou Y. Modulation of proliferation and differentiation of gingiva derived mesenchymal stem cells by concentrated growth factors: potential implications in tissue engineering for dental regeneration and repair. Int J Mol Med. 2019;34:45–65.
- Diniz IM, Chen C, Ansari S, et al. Gingival mesenchymal stem cell (GMSC) delivery system based on RGD-coupled alginate hydrogel with antimicrobial properties: a novel treatment modality for peri-implantitis. J Prosthodont. 2016;25(2):105–115.
- Dohan DM, Choukroun J, Diss A, et al. Platelet-rich fibrin (PRF): a second-generation platelet concentrate. Part III: leucocyte activation: a new feature for platelet concentrates? Oral Surg Oral Med Oral Pathol Oral Radiol Endod. 2006;101(3):e51–5.
- Peixe GPG, Lourenço SIM, de Vasconcelos Gurgel BC. Immunohistochemical study of CD34 and podoplanin in periodontal disease. J Periodont. 2019;43:78–89.
- Zhao N, Suzuki A, Zhang X, et al. Dual aptamer-functionalized in situ injectable fibrin hydrogel for promotion of angiogenesis via co-delivery of VEGF and PDGF-BB. ACS Appl Mater Interfaces. 2019;108:44–65.
- Kim JE, Lee J, Jang M, et al. Accelerated healing of cutaneous wounds using phytochemically stabilized gold nanoparticle deposited hydrocolloid membranes. Biomater Sci. 2015;3(3):509–519.
- Kobayashi K, Ichihara Y, Sato N, et al. On-site fabrication of bi-layered adhesive mesenchymal stromal cell-dressings for the treatment of heart failure. Biomaterials. 2019;209:41–53.
- Chen XJ, Shen YS, He MC, et al. Polydatin promotes the osteogenic differentiation of human bone mesenchymal stem cells by activating the BMP2-Wnt/β-catenin signaling pathway. Biomed Pharmacother. 2019;112:108746.
- Lin KC, Park HW, Guan KL. Regulation of the Hippo pathway transcription factor TEAD. Trends Biochem Sci. 2017;42(11):862–872.
- Liu L, Liu YQ, Feng C, et al. Lithium-containing biomaterials stimulate bone marrow stromal cell-derived exosomal miR-130a secretion to promote angiogenesis. Biomaterials. 2019;192:523–536.
- Yılmaz O, Özmeriç A, Alemdaroğlu KB, et al. Effects of concentrated growth factors (CGF) on the quality of the induced membrane in Masquelet’s technique – sn experimental study in rabbits. Injury. 2018;49(8):1497–1503.
- Wang X, Yang Y, Zhang Y, et al. Fluid platelet-rich fibrin stimulates greater dermal skin fibroblast cell migration, proliferation, and collagen synthesis when compared to platelet-rich plasma. J Cosmet Dermatol. 2019;18(6):2004–2365.