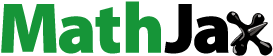
Abstract
Chrysanthemum morifolium (Chr) is a traditional Chinese medicine (TCM) that has been used in the treatment of inflammation-linked diseases for hundreds of years. Naringenin (Nar) and apigenin (Api) are the major active components in aqueous extracts of C. morifolium. The aim of our study was to clarify the roles of Chr, Nar and Api in ameliorating depression-like behaviour induced by corticosterone. First, the behavioural and biochemical indicators closely related to depression were examined to evaluate the therapeutic effects of Chr/Nar/Api on a depression model. Then, a metabolomics approach was utilized to screen for biomarkers and related pathways between a control group and Chr/Nar/Api groups. The comprehensive results revealed that Chr/Nar/Api exerted anti-depressant effects through interfering with tryptophan metabolism, arginine and prolinemetabolism, citrate cycle, niacin and niacinamide metabolism, phenylalanine metabolism, and alanine, aspartate and glutamate metabolism. The mechanism of Chr/Api/Nar in the treatment of depression was elucidated based on material and energy metabolism. Moreover, Nar could be used as a substitute for Chr for reversing depression-like behaviour, and Api was similar to a positive drug in terms of function on depression. The integrated metabolomics approach demonstrated here should be an effective method for interpreting the function of herbs from TCM and clarifying the mechanism of their components in future studies.
Introduction
Depression is a common mental disorder that is characterized by anhedonia, insomnia, loss of appetite, loss of concentration and even suicidal tendencies [Citation1]. Many synthetic anti-depressants, such as tricyclic anti-depressants (TcAs), tetracyclic anti-depressants (TeCAs), and selective serotonin reuptake inhibitors (SSRIs), have been introduced to control the development of depression in the clinic [Citation2,Citation3]. Herbal alternatives, such as St John’s Wort, and nutritional supplements, including probiotics, have also become popular remedies used by the public [Citation4,Citation5].
Chrysanthemum morifolium (Chr), the dried capitulum of C. morifolium Ramat., is used as both a beverage and a traditional Chinese medicine (TCM) in China. The C. morifolium extract has anti-inflammatory effects [Citation6,Citation7]. However, proinflammatory cytokines, acting as neuromodulators, mediate the pathogenesis of the behavioural, neuroendocrine, and neurochemical features of depressive disorders [Citation8]. Additionally, inflammatory cytokines are potential treatment targets for depression [Citation9]. However, there has been a lack of suitable strategy for clarifying the efficacy and mode of action of Chr on depression.
Naringenin (Nar; ) and Apigenin (Api; ) are the major active components in an aqueous extract of Chr. Nar (4′,5,7-trihydroxydihydroflavone) is a dihydrogen flavonoid, which exists in many plants, including, rutaceae, citrus and rosaceae. It possesses a variety of pharmacological activities, such as anti-inflammation [Citation10], anti-oxidation [Citation11,Citation12], anti-cancer [Citation13] and anti-viral activities [Citation14]. Moreover, Nar has been shown to significantly attenuate doxorubicin-induced anxiety and depressive-like behaviour [Citation15,Citation16]. Api (4′,5,7- trihydroxy flavone) is a flavonoid that has various pharmacological effects, including anti-oxidation, anti-tumor and anti-inflammation activities. Furthermore, Api can significantly ameliorate chronic, mild stress-induced depressive behaviour [Citation17]. However, the anti-depression mechanism of Nar and Api is still unclear.
Metabolomics is a powerful approach for studying the complex metabolic changes caused by genetic or environmental stimuli [Citation18]. This approach can broaden the research horizons of genomics and proteomics through presenting additional information, such as information on drug toxicity, gene function and the pathological mechanism of diseases [Citation19]. As a part of systems biology, metabolomics provides an ideal approach for understanding the mechanism of complex component medicines by revealing systemic metabolite changes. In terms of metabolomic tools, proton nuclear magnetic resonance (1H NMR), gas chromatography-mass spectrometry (GC-MS) and liquid chromatography-mass spectrometry (LC-MS) are the main analytical platforms adopted in metabolomics studies, but 1H NMR suffers from low sensitivity and may not detect low-abundance metabolites [Citation20]. In recent years, the combinations of gas chromatography-quadrupole time-of-flight mass spectrometry (GC-Q-TOF/MS) and liquid chromatography-quadrupole time of flight mass spectrometry (LC-Q-TOF/MS) has been applied successfully in numerous metabolomics studies to achieve more sensitive and accurate metabolic profiling and screening of biomarkers [Citation21].
To clarify the therapeutic mechanism of Chr, Nar and Api in ameliorating depression, a depression model was established via corticosterone in this study. Then, an integrated metabolomic analysis was performed by UPLC-Q/TOF-MS.
Materials and methods
Animals
Male C57BL/6 mice (weighing 18–22 g) were obtained from Beijing Vital River Laboratory Animal Technology Co., Ltd. (animal licence number: SCXK (Jing) 2016-0006; Beijing, China), housed individually in cages, and provided with standard rat chow and tap water ad libitum. The room temperature and relative humidity were maintained at 22 ± 2 °C and 45 ± 15%, respectively, under a 12-h light/dark cycle starting at 8 a.m. After a habituation period of one week, 48 mice were randomly divided into three groups: a control (C, 8) group, a corticosterone (Cor, 8) group and a therapy group (C, 32) for examination. The therapy group included a fluoxetine (Flu, 8), chrysanthemum (Chr, 8), naringenin (Nar, 8) and apigenin (Api, 8) group. The Cor group received corticosterone (20 mg·kg−1) through irregular subcutaneous injection, and then the Flu, Chr, Nar, and Api group mice received Flu (5 mg·kg−1), Chr (3.33 g·kg −1), Nar (100 mg·kg−1) and Api (20 mg·kg−1), respectively. The drugs were orally administered once a day for 21 days, while the model group and control group mice received the same volume of a sterile saline solution. The study was performed according to the Experimental Animal Administration regulations issued by the State Committee of Science and Technology of the People’s Republic of China. Mice were handled according to the guidelines provided by Henan University of Chinese Medicine Committee on Experimental Animal Welfare.
Chemical and reagents
HPLC-grade acetonitrile was purchased from Fisher Chemical (USA). Purified water was prepared with Molecular Water Purification system. Formic acid (LC-MS grade, FA) was purchased from Anaqua Chemicals Supply Inc. (USA). Corticosterone (Cayman, USA) and Fluoxetine (Patheon, France) Chr was purchased from Wanxi Pharmaceutical Company, Henan Province. The raw herbal medicines were identified as the dried capitulum of C. morifolium Ramat. by Professor Suiqing Chen and Chengming Dong. Specimen vouchers for these herbs are kept in our laboratory at the Henan University of Chinese Medicine. Nar and Api were obtained from the flowers of C. morifolium Ramat.
Extraction
Dried Chr (1 kg) was decocted with water three times (10 L × 3, 1 h each time) at 100 °C, and then the combined supernatants were evaporated and dried in vacuum to obtain an extract of Chr.
Sucrose preference test
Before the sucrose preference, test mice were trained on a sucrose solution. Two bottles of a 1% (w/v) sucrose solution were placed in each cage for 24 h at the same time, and then one bottle of sucrose solution was replaced with water. After 12 h, the position of the two bottles was changed for feeding for 12 h to avoid possible effects of side preference in this test. After adaptation, mice were deprived of water and food for 24 h, and then housed in individual cages and given free access to the two bottles containing the sucrose solution (1%, w/v) and water, respectively. After 12 h, the volume (ml) of both the consumed sucrose solution and water were recorded, and the sucrose preference was calculated using the following formula:
Sample collection and preparation
At the termination of the experiment (day 21), all of the mice in each group were housed in metabolic cages to collect 24-h urine samples after fasting for 12 h, with water being allowed. The urine samples were stored at −80 °C until analysis.
Urine samples were thawed in ice water before analysis and centrifuged at 12,000 rpm for 10 min, and then the supernatant was mixed at a ratio of 1:3 with cold acetonitrile (4 °C), and 2 µl of the supernatant was injected into a UPLC-Q/TOF-MS system after centrifugation at 12,000 rpm for 10 min.
The same and minute volume of (30 μl) each urine sample was extracted to mix into Quality Control (QC) samples that contained most of the information in whole urine samples. QC samples were prepared by the same method as the urine samples and injected into the UPLC-Q/TOF-MS system after running every 10 urine samples to surveil the stability of the whole analysis system.
Measurement of serum corticosterone and 5-hydroxytryptamine levels
After the urine samples were collected, blood was collected from the eyeball, and then centrifuged at 3000 rpm for 10 min. Finally the supernatant was taken and stored at −80 °C until analysis. Serum corticosterone and 5-hydroxytryptamine levels were measured by using a commercially available enzyme-linked immunosorbent assay kit (ELISA; Suzhou Calvin Biotechnology Co., Ltd., China) according to the manufacturer’s instructions. Absorbance was measured at 450 nm using a microplate reader (Bio-Rad, USA).
Uplc-Q/TOF-MS analysis
The urine samples were separated at 40 °C on an Ultra-Performance Liquid Chromatography (UPLC) system (Dionex; UltiMate 3000 System, Thermo Scientific, USA) equipped with an Acclaim6 RSLC 120 C 18 column (2.2 µm, 2.1 × 100 mm; Thermo Scientific). The mobile phases consisted of acetonitrile (A) and water (B) containing 0.1% formic acid. The flow rate of the mobile phase was 0.3 ml/min. The gradient elution programme was as follows: (0–2 min, 10–15% A; 2–7 min, 15–30% A; 7–15 min, 30–70% A; 15–20 min and 70–90% A).
MS analysis was performed by a Q/TOF mass spectrometer system equipped with an ESI ion source. The full-scan mass data were from 50 to 1500 m/z with a scan time of 0.2 s and spectra rate of 1.00 HZ, desolvation temperature of 230 °C, desolvation gas flow of 50 l/h, a power source of 3.0 eV, with capillary voltage, 3.2 kV in the positive mode and 3.5 kV in the negative mode, was used. Na-Formate was used as the lock-mass throughout all of the analyses with a flow rate of 50 µl/min.
Statistical analysis
Prior to using principal components analysis (PCA) and orthogonal partial least squares discriminant (OPLS-DA) analysis, the original data collected by UHPLC-Q/TOF-MS were imported into Profile Analysis (version 2.1, Bruker Germany) to pretreat the data, including peak alignment, background noise subtraction and normalization. Then, the achieved statistical table was imported into SIMCA-P software (version 13.0 Umetrics AB, Sweden) for multivariate analysis (PCA and OPLS-DA), and the obtained score scatter plot was used to observe the global clustering trends of various groups and visualize their distributions. The R2 and Q2 values were calculated, respectively, to evaluate the fitness and predictive capability of two constructed pattern recognition models. Finally, potential biomarkers were filtered by the results of variable importance for the projection (VIP) values (VIP > 1) and t-test (p<.05) values.
The identification of potential biomarkers was performed by comparison of mass-to-charge ratio (m/z) with relevant published literature, the Human Metabolome Database (http://www.hmdb.ca/) and METLIN (https://metlin.scripps.edu/). Semi-quantitative analysis (thermogram) and cluster analysis of potential biomarkers were performed using Mev (version 4.8.0). Finally, correlation networks for disturbed pathways in depression mice were constructed based on KEGG (http://www.kegg.jp/) and Metabo Analyst (http://www.metaboanalyst.ca/).
Results
Biochemical assessment
First, we estimated the anti-depressive effect of Chr. As shown in , chronic exposure to corticosterone induced a significant decrease in both sucrose preference and blood serum 5-HT levels, while the corticosterone levels increased significantly. Compared with the model group, Chr and Flu treatment significantly increased the sucrose preference and the serum 5-HT levels, and decreased the corticosterone level.
Figure 2. Influence of Flu and Chr on sucrose consumption (A), serum levels of 5-hydroxytryptamine (B) and corticosterone levels (C). All of the values are given as mean ± SD (n = 8), **p<.001 vs. C group; #p<.05 vs. Cor group; ##p<.01 vs. Cor group. Control (C)/Corticosterone (Cor)/Fluoxetine (Flu)/Chrysanthemum morifolium (Chr) group.
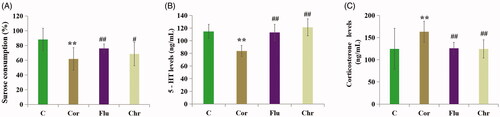
Consequently, the anti-depressive effects of Nar and Api were assessed. As shown in , Nar and Api could also improve all of the assessed biochemical indices to some extent.
Figure 3. Influence of Flu, Nar and Api on Sucrose consumption (A), serum level of 5-hydroxytryptamine (B) and corticosterone (C). All of the values are given as mean ± SD (n = 8), **p<.001 vs. C group; #p<.05 vs. Cor group; ##p<.01 vs. Cor group. Control (C)/Corticosterone (Cor)/Fluoxetine (Flu)/Naringenin (Nar)/Apigenin (Api) group.
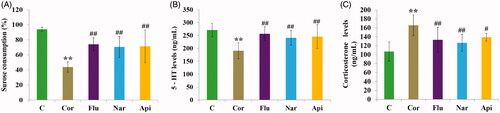
Multivariate analysis of urine metabolomics
Principal components analysis was performed to explore the effects of Flu, Chr, Nar, and Api on depressed mice. A clear separation between the C and Cor groups was observed in (ESI+ R2X = 0.709, Q2 = 0.493; ESI− R2X = 0.762, Q2=0.584), indicating that the metabolic profiles differed significantly between them. As shown in ), the Flu, Chr, Nar and Api groups all trended towards the C group in the direction of the first principal component, indicating that they all contributed to the recovery of depressed mice towards the normal condition in some ways.
Identification of biomarkers
To investigate the metabolic fluctuations induced by corticosterone, supervised orthogonal partial least squares discriminant analysis was applied (OPLS-DA) to construct classification models and distinguish the differential variables between the Cor and C/Flu/Chr/Nar/Api group, respectively (ESI+: C vs. Cor: R2X = 0.729, R2Y = 0.993, Q2 = 0.925; Cor vs. Chr: R2X = 0.744, R2Y = 0.969, Q2 = 0.911; Cor vs. Nar: R2X = 0.741, R2Y = 0.997, Q2 = 0.969; Cor vs. Api: R2X = 0.754, R2Y = 0.994, Q2 = 0.947; ESI−: C vs. Cor: R2X = 0.829, R2Y = 0.991, Q2 = 0.958; Cor vs. Chr: R2X = 0.707, R2Y = 0.998, Q2 = 0.994; Cor vs. Nar: R2X = 0.812, R2Y = 0.994, Q2 = 0.752; and Cor vs. Api: R2X = 0.862, R2Y = 0.999, Q2 = 0.985). In both the positive and negative ion mode, OPLS-DA score plots showed obvious separation between the Cor group and the C/Chr/Nar/Api group (Supplementary Figure 1*). The variable importance in the projection (VIP) value of each variable in the OPLS-DA model was calculated to indicate its contribution to the classifications. The potential biomarkers with VIP values (>1 and t-test (p<.05)) were further carefully filtered in METLIN (http://metlin.scripps.edu/), HMDB (http://www.hmdb.ca/) and KEGG (http://www.genome.jp/kegg/) using exact molecular weights or MS/MS fragmentation pattern data. Consequently, a total of 80 potential biomarkers are listed in Supplementary Table 1*, revealing their metabolic fluctuation in depressed mice. The changing trends of the potential biomarkers are shown in Supplementary Table 2*. Furthermore, Venn diagrams () showed that the 57, 58, and 47 biomarkers were significantly regulated by Nar, Api and Chr, respectively, while 30 biomarkers were regulated by all 3. To visualize the variation tendency of the metabolite levels between different groups, we produced a heat map of the 30 shared metabolites ().
Figure 5. Venn diagram of metabolites showing the commonality and peculiarity between the Chr/Nar/Api groups (A); heatmap of the top 30-shared metabolites (B). The colours from green to red indicate the increasing level of metabolites. (Control (C)/Corticosterone (Cor)/Fluoxetine (Flu)/Chrysanthemum morifolium (Chr)/Naringenin (Nar)/Apigenin (Api) groups).
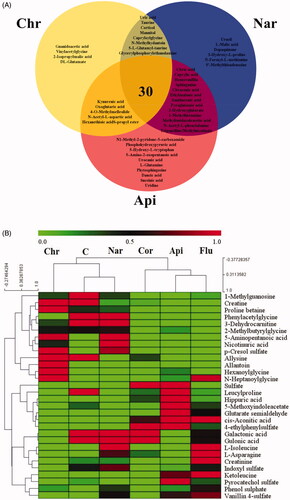
Correlation network of disturbed pathways
To investigate the potential associations between metabolites, pathway enrichment analysis was first performed on the basis of MetaboAnalyst 4.0 (www.metaboanalyst.ca) in this study. Then, the related metabolic pathway profiles were obtained based the on above consequences and the Kyoto Encyclopaedia of Genes and Genomes (KEGG) pathway database (http://www.kegg.jp/kegg/pathway. html). As shown in , the six disturbed metabolic pathways, namely, tryptophan metabolism; glycine, serine and threonine metabolism; alanine, aspartate, and glutamate metabolism; arginine and proline metabolism; citrate cycle and nicotinate and nicotinamide metabolism, were closely interconnected with each other.
Discussion
The corticosterone-induced model successfully simulated the symptoms of depression, including the increase in serum corticosterone levels, the decline of sucrose consumption, as well as decreases in the serum 5-hydroxytryptamine level. The results indicated that Chr/Nar/Api markedly ameliorated the corticosterone-induced changes, whereas the detection of several indexes cannot fully respond to the mechanism of drug action. Therefore, we analysed the urine samples of all of the experimental groups using metabolomics to compare the systemic therapeutic effects of Chr, Nar and Api.
Tryptophan metabolism
5-Hydroxytryptamine (5-HT) is an important product of tryptophan metabolism. It is an important brain neurotransmitter that is implicated in mental and neurodegenerative diseases and is modulated by ovarian hormones [Citation22]. Related studies have shown that depressed patients have a significantly lower level of 5-HT [Citation23]. As shown in , tryptophan is metabolized to 5-hydroxy-L-trptophan (precursor of 5-hydroxy tryptamine) in the body. In this study, the level of 5-hydroxy-L-trptophan in the Cor group was lower than that in the C group. As the precursor of neurotransmitter 5-HT, the lower level of 5-hydroxy-L-trptophan could lead to the reduction of 5-HT. After the administration of Api, the level of 5-hydroxy-L-trptophan was increased. Furthermore, 5-methoxyindoleacetate is the metabolite of 5-HT. The decrease in its level also meant that the secretion of 5-HT was insufficient. After the administration of Chr/Nar/Api, the level of 5-methoxyindoleacetate increased. This suggested that Chr/Nar/Api could regulate tryptophan metabolism, and thus, improve the secretion of 5-HT to exert anti-depressive effects. Tryptophan is both a precursor of 5-HT and a kynurenine. Tryptophan is catalyzed by tryptophan 2,3-dioxygenase (TDO) and indoleamine 2,3-dioxygenase (IDO) to generate kynurenine, and then kynurenine is metabolized by kynurenine aminotransferase (KAT) to form kynurenic acid [Citation24]. As shown in Supplementary Table 2*, the level of kynurenic acid in the Cor group was lower than that in the C group, suggesting that the TDO, IDO and KAT activity reduced in the Cor group. After the administration of Chr/Api, the level of kynurenic acid also dramatically increased. Therefore, Chr and Api could exert anti-depressive effects by elevating the level of TDO, IDO and KAT activity.
Arginine and proline metabolism
Arginine and proline metabolism was reported previously to significantly disturbed in major depressive disorders [Citation25]. Creatine is an important product of arginine and proline metabolism. Creatine is further metabolized to form 1-methyl-hydantoin. At present, it is agreed that there is abnormal growth hormone secretion in patients with depression [Citation26]. Experiments have shown that 1-methylhydantoin can reduce the level of growth hormone in rats [Citation27]. The level of 1-methylhydantoin was increased after the administration of Chr/Nar, indicating that Chr and Nar can regulate the secretion of growth hormones to play a role as anti-depressants, but Api did not have this effect. In addition, ATP is a key factor involved in astrocytic modulation of depressive-like behaviour in mice. Low ATP abundance was detected in the brains of mice that were susceptible to chronic social defeat. Furthermore, others have observed that ATP induced a rapid anti-depressant-like effect in mice [Citation28]. Creatine exists in the form of creatine and creatine phosphate in muscle tissue. The cytosolic creatine kinase isoenzyme can catalyze the reversible transfer of N-phosphoryl acid from creatine phosphate to adenosine diphosphate (ADP), thus producing ATP and creatine [Citation29]. The concentration of creatine in the Cor model group decreased significantly compared with the C group. Upregulation of creatine by Chr/Nar/Api indicated that Chr and its two active components could promote the body to produce more ATP and reverse the disorder of arginine metabolism in depressed mice. However, creatine can exert anti-depressant effects by affecting the K-3β/Nrf2 pathway [Citation30]. Thus, additional creatine production can better ameliorate depressive-like behaviour in Cor model groups.
Citrate cycle
Energy deficiency or fatigue is one of the most frequently reported symptoms in major depressive disorders [Citation31]. Sugar, fat, and protein are the main substances of the human energy supply. Although the characteristics are different in terms of the supply of energy, the citrate cycle is the final metabolic pathway for utilizing sugar in mammals [Citation32]. Compared with the C group, the oxoglutaric acid, cis-aconitic acid, succinic acid, L-malic acid and citric acid level in the urine of depressed mice decreased significantly. The decrease of these biomarkers suggested that there was an obstacle to energy metabolism in mice with depression, which may be the cause of fatigue in mice [Citation33]. The production of succinic acid is the result of succinyl-coenzyme A being catalyzed by succinyl CoA synthetase. This process is the only substance-level phosphorylation in the Krebs cycle. The level of succinic acid in the Cor group was significantly lower than that in the C group, indicating that indeed there was a barrier to the energy supply system of depressed mice. After the administration of Api, the level of succinic acid significantly increased, indicating that Api can improve the production of succinic acid, ameliorates the barrier of energy metabolism, and then exerts anti-depressant effects.
A systematic review and meta-analysis found that oxidative stress is elevated in major depressive disorders [Citation34]. Meanwhile, evidence that successful anti-depressant treatment may improve oxidative stress status [Citation35]. As shown in , citric acid is catalyzed by aconitase to form cis-aconitic acid [Citation36]. cis-Aconitic acid is related to oxidative stress. During stages of electrophilic stress, cis-aconitic acid can react with superoxide to form ˙OH, rendering them inactive [Citation37]. After the administration of Chr/Nar, the level of citric acid did not increase, while the level of cis-aconitic acid increased significantly and indicated that Chr and Nar may convert more citric acid into cis-aconitic acid by promoting the expression of aconitase to ameliorates the deficiency of cis-aconitic acid secretion in depressed mice. Therefore, Chr/Nar could promote the expression of aconitase to improve oxidative stress of depressed mice.
Niacin and niacinamide metabolism
Niacin is one of the 13 essential vitamins, and it can be converted to nicotinamide in the human body [Citation38]. Niacin deficit symptoms include several nervous system pathologies, such as dementia and depression, as well as other symptoms resembling those observed in other neurodegenerative diseases [Citation39]. In our study, a low level of niacin in depressed mice was detected. After the administration of Chr and its two active components, the level of nicotinuric acid increased significantly. In terms of energy metabolism, nicotinamide and phosphoribosyl- pyrophosphate combine to generate nicotinamide mononucleotide, which can continue to react with adenosine triphosphate (ATP) to generate coenzyme I. The generation of coenzyme II (NADP) is the result of the combination of coenzyme I and ATP. Coenzyme I and coenzyme II are coenzymes of dehydrogenase and indispensable substance transfer carriers in the human body that participate in the process of lipid metabolism and saccharide anaerobic decomposition. The symptoms of energy deficiency or fatigue in patients with major depression have been described above [Citation36]. Chr and its two active components could increase the level of nicotinuric acid. Furthermore, as a direct metabolite of niacin, the level of nicotinuric acid increased after Chr/Nar/Api treatment, indicating that more niacin exerts its biological activity in the body. Thus, Chr/Nar/Api improved the energy supply by accelerating the niacin and niacinamide metabolism in depressed mice.
Phenylalanine metabolism
From the theories of TCM, depression is closely related to liver injury, and elevated blood ammonia levels may be one of the important causes of abnormalities in the liver [Citation40]. The conjugation of glycine with phenylacetate to form phenylacetylglycine through acyl-CoA has been shown to be a new ammonia-lowering route [Citation41]. After the administration of Chr/Nar/Api, the level of phenylacetylglycine increased. This indicated that Chr/Nar/Api could ameliorate liver injury in depressed mice by reducing the level of blood ammonia. Hippuric acid is also a metabolite of glycine [Citation42]. Glycine is deceased in the liver periphery, and may serve as a clinical trait-marker for depression [Citation43]. Glycine is currently a favoured therapeutic target for rapid anti-depressant action [Citation44]. The levels of phenylacetylglycine and hippuric acid were significantly increased after the administration of Chr and its two active components, indicating that the level of total glycine had increased.
Alanine, aspartate, and glutamate metabolism
Depression is closely related to alanine, aspartate and glutamate metabolism [Citation45]. Under long-term stress, the continuous activation of the hypothalamic–pituitary–adrenal axis (HPA) increases the corticosterone content in the body, prompting glial cells to release glutamate and cause nerve cell damage or apoptosis [Citation46]. Meanwhile, the glutamate content in the urine of mice with depression showed a decreasing trend compared with group C in our study. After the administration of Chr and Api, the glutamate content increased significantly, indicating that Chr and Api could improve glutamate metabolism disorder in depressed mice, thus exerting anti-depressant effects.
Conclusion
A UPLC-Q/TOF-MS-based urinary metabolomics method was established to evaluate the anti-depressive efficacy and mechanism of Chr and its two active components. The integrating results of biochemistry and metabolomics study indicated that Chr/Nar/Api exerted their anti-depressive effects mainly through interfering with six metabolic pathways, namely, tryptophan metabolism, arginine and proline metabolism, citrate cycle, niacin and niacinamide metabolism, phenylalanine metabolism, and alanine, aspartate and glutamate metabolism. Nar could be used as a substitute for Chr in reversing depression-like behaviour, and Api was similar to fluoxetine as an anti-depressant. Furthermore, this integrated metabolomic approach is a reliable strategy for researching the mechanism of Chinese medicinal herbs and their active components.
1_LABB_2019_1461.R1_supplementary_material.docx
Download MS Word (534.5 KB)Additional information
Funding
References
- Malhi GS, Mann JJ. Depression. Lancet. 2018;392(10161):2299–2312.
- Jakobsen JC, Katakam KK, Schou A, et al. Selective serotonin reuptake inhibitors versus placebo in patients with major depressive disorder. A systematic review with meta-analysis and trial sequential analysis. BMC Psychiatry. 2017;17(1):58.
- Cipriani A, Furukawa TA, Salanti G, et al. Comparative efficacy and acceptability of 21 antidepressant drugs for the acute treatment of adults with major depressive disorder: a systematic review and network meta-analysis. Lancet. 2018;391(10128):1357–1366.
- Ng QX, Venkatanarayanan N, Ho CY. Clinical use of Hypericum perforatum (St John’s wort) in depression: a meta-analysis. J Affect Disord. 2017;210:211–221.
- Ng QX, Peters C, Ho CYX, et al. A meta-analysis of the use of probiotics to alleviate depressive symptoms. J Affect Disord. 2018;228:13–19.
- Ukiya M, Akihisa T, Yasukawa K, et al. Constituents of compositae plants. 2. Triterpene diols, triols, and their 3-o-fatty acid esters from edible chrysanthemum flower extract and their anti-inflammatory effects. J Agric Food Chem. 2001;49(7):3187–3197.
- Yoo KY, Kim IH, Cho JH, et al. Neuroprotection of Chrysanthemum indicum Linne against cerebral ischemia/reperfusion injury by anti-inflammatory effect in gerbils. Neural Regen Res. 2016;11(2):270–277.
- Ng QX, Ramamoorthy K, Loke W, et al. Clinical role of aspirin in mood disorders: a systematic review. Brain Sci. 2019;9(11):296.
- Achtyes E, Keaton SA, Smart L, et al. Inflammation and kynurenine pathway dysregulation in post-partum women with severe and suicidal depression. Brain Behav Immun. 2020;83:239–247.
- Han NR, Moon PD, Ryu KJ, et al. Inhibitory effect of naringenin via IL-13 level regulation on thymic stromal lymphopoietin-induced inflammatory reactions. Clin Exp Pharmacol Physiol. 2018;45(4):362–369.
- Wojnar W, Zych M, Kaczmarczyk-Sedlak I. Antioxidative effect of flavonoid naringenin in the lenses of type 1 diabetic rats. Biomed Pharmacother. 2014;307:1024–1031.
- Cavia-Saiz M, Busto MD, Pilar-Izquierdo MC, et al. Antioxidant properties, radical scavenging activity and biomolecule protection capacity of flavonoid naringenin and its glycoside naringin: a comparative study. J Sci Food Agric. 2010;90(7):1238–1244.
- Chandrika BB, Steephan M, Kumar TRS, et al. Hesperetin and Naringenin sensitize HER2 positive cancer cells to death by serving as HER2 Tyrosine Kinase inhibitors. Life Sci. 2016;160:47–56.
- Jia B, Yu D, Yu G, et al. Naringenin improve hepatitis C virus infection induced insulin resistance by increase PTEN expression via p53-dependent manner. Biomed Pharmacother. 2018;103:746–754.
- Bansal Y, Singh R, Saroj P, et al. Naringenin protects against oxido-inflammatory aberrations and altered tryptophan metabolism in olfactory bulbectomized-mice model of depression. Toxicol Appl Pharmacol. 2018;355:257–268.
- Kwatra M, Jangra A, Mishra M, et al. Naringin and sertraline ameliorate doxorubicin-induced behavioral deficits through modulation of serotonin level and mitochondrial complexes protection pathway in rat hippocampus. Neurochem Res. 2016;41(9):2352–2366.
- Li R, Wang X, Qin T, et al. Apigenin ameliorates chronic mild stress-induced depressive behavior by inhibiting interleukin-1β production and NLRP3 inflammasome activation in the rat brain. Behav Brain Res. 2016;296:318–325.
- Nicholson JK, Lindon JC. Systems biology: metabonomics. Nature. 2008;455(7216):1054–1056.
- Nicholson JK, Connelly J, Lindon JC, et al. Metabonomics: a platform for studying drug toxicity and gene function. Nat Rev Drug Discov. 2002;1(2):153–161.
- Ong ES, Chor CF, Zou L, et al. A multi-analytical approach for metabolomic profiling of zebrafish (Danio rerio) livers. Mol Biosyst. 2009;5(3):288–298.
- Wang P, Ng QX, Zhang H, et al. Metabolite changes behind faster growth and less reproduction of Daphnia similis exposed to low-dose silver nanoparticles. Ecotoxicol Environ Saf. 2018;163:266–273.
- Sánchez MG, Morissette M, Di Paolo T. Oestradiol modulation of serotonin reuptake transporter and serotonin metabolism in the brain of monkeys. J Neuroendocrinol. 2013;25(6):560–569.
- Albert PR, Lemonde S. 5-HT1A receptors, gene repression, and depression: guilt by association. Neuroscientist. 2004;10(6):575–593.
- Myint AM. Kynurenines: from the perspective of major psychiatric disorders. Febs J. 2012;279(8):1375–1385.
- Ozden A, Angelos H, Feyza A, et al. Altered plasma levels of arginine metabolites in depression. J Psychiatr Res. 2020;120:21–28.
- Kristiansen RG, Rose CF, Fuskevåg OM, et al. GHS-R1a deficiency alleviates depression-related behaviors after chronic social defeat stress. Front Neurosci. 2019;13:364.
- Fei L, Xiao-Chun Z, Qing-Mo L. 1-methylhydantoin inhibits secretion of growth hormone in rabbits. Chin Pharmacol Bull. 2017;33:401–406.
- Cao X, Li LP, Wang Q, et al. Astrocyte-derived ATP modulates depressive-like behaviors. Nat Med. 2013;19(6):773–777.
- Whittington HJ, Ostrowski PJ, McAndrew DJ, et al. Over-expression of mitochondrial creatine kinase in the murine heart improves functional recovery and protects against injury following ischaemia-reperfusion. Cardiovasc Res. 2018;114(6):858–869.
- Rosa JM, Pazini FL, Cunha MP, et al. Antidepressant effects of creatine on amyloid β1-40-treated mice: the role of GSK-3β/Nrf2 pathway. Prog Neuropsychopharmacol Biol Psychiatry. 2018;86:270–278.
- Serretti A, Mandelli L, Lattuada E, et al. Depressive syndrome in major psychoses: a study on 1351 subjects. Psychiatry Res. 2004;127(1–2):85–99.
- Bowtell JL, Marwood S, Bruce M, et al. Tricarboxylic acid cycle intermediate pool size: functional importance for oxidative metabolism in exercising human skeletal muscle. Sports Med. 2007;37(12):1071–1088.
- Sahlin K, Tonkonogi M, Söderlund K. Energy supply and muscle fatigue in humans. Acta Physiol Scand. 1998;162(3):261–266.
- Iqbal M, Ullah S, Zafar S, et al. Effect of exercise interventions on kainate induced status epilepticus and associated co-morbidities; a systematic review and meta-analysis. Neurochem Res. 2019;44(5):1005–1019.
- Jiménez-Fernández S, Gurpegui M, Díaz-Atienza F, et al. Oxidative stress and antioxidant parameters in patients with major depressive disorder compared to healthy controls before and after antidepressant treatment: results from a meta-analysis. J Clin Psychiatry. 2015;76(12):1658–1667.
- Quirós PM. Determination of aconitase activity: a substrate of the mitochondrial lon protease. Methods Mol Biol. 2018;1731:49–56.
- Vasquez-Vivar J, Kalyanaraman B, Kennedy MC. Mitochondrial aconitase is a source of hydroxyl radical. An electron spin resonance investigation. J Biol Chem. 2000;275(19):14064–14069.
- Fricker RA, Green EL, Jenkins SI, et al. The influence of nicotinamide on health and disease in the central nervous system. Int J Tryptophan Res. 2018;11:32–24.
- Gasperi V, Sibilano M, Savini I, et al. Niacin in the central nervous system: an update of biological aspects and clinical applications. IJMS. 2019;20(4):974.
- Weiss N, Mochel F, Rudler M, et al. Peak hyperammonemia and atypical acute liver failure: the eruption of an urea cycle disorder during hyperemesis gravidarum. J Hepatol. 2017; 68: 185–192.
- Kristiansen RG, Rose CF, Fuskevåg OM, et al. L-Ornithine phenylacetate reduces ammonia in pigs with acute liver failure through phenylacetylglycine formation: a novel ammonia-lowering pathway. Am J Physiol Gastrointest Liver Physiol. 2014;307:1024–1031.
- Kasumov T, Brunengraber LL, Comte B, et al. New secondary metabolites of phenylbutyrate in humans and rats. Drug Metab Dispos. 2004;32(1):10–19.
- Zheng P, Gao HC, Li Q, et al. Plasma metabonomics as a novel diagnostic approach for major depressive disorder. J Proteome Res. 2012;11(3):1741–1748.
- Machado-Vieira R, Henter ID, Zarate CA. Jr. New targets for rapid antidepressantaction. Prog Neurobiol. 2017;152:21–37.
- Duman RS, Sanacora G, Krystal JH. Altered connectivity in depression: GABA and glutamate neurotransmitter deficits and reversal by novel treatments. Neuron. 2019;102(1):75–90.
- Workman JL, Gobinath AR, Kitay NF, et al. Parity modifies the effects of fluoxetine and corticosterone on behavior, stress reactivity, and hippocampal neurogenesis. Neuropharmacology. 2016;105:443–453.