Abstract
5-Aminolevulinic acid hydrochloride (ALA)-mediated sonodynamic therapy (SDT) had anti-tumour effect on pancreatic cancer cells. Hence, ALA loaded lipid/poly(lactic-co-glycolic acid) (PLGA) microbubbles (MBs)-mediated SDT for pancreatic cancer has great potential. The average size of ALA-lipid MBs and ALA-PLGA MBs was about 3.0 µm. The two kinds of MBs had good biocompatibility to normal HPDE6-C7 cells and were not toxic to pancreatic cancer cells. Compared with ALA-induced SDT, a statistically significant decrease in cell viability was observed in ALA lipid/PLGA MBs combined with ultrasound groups in AsPC-1 and BxPC-3 cells (p < .05). Obvious effect on the apoptotic rate, apoptosis and pyroptosis morphology, enhanced reactive oxygen species was found in ALA-lipid/PLGA MBs mediated SDT in vitro. Through in vivo study, we found ALA-lipid/PLGA MBs-mediated SDT was a promise treatment for pancreatic cancer.
Introduction
Conventional therapy of pancreatic cancer such as surgery, chemotherapy, radiotherapy, targeted therapy or combined treatment has shortcomings such as low selectivity, systemic toxicity and drug resistance. Therefore, the development of novel, minimally invasive and highly selective cancer therapeutic methods is urgently needed.
Sonodynamic therapy (SDT) is composed of sonosensitizer, low intensity ultrasound and molecular oxygen. 5-aminolevulinic acid hydrochloride (ALA) is an effective sonosensitizer for cancer treatment using SDT, ALA-mediated SDT created in vitro anti-tumour effect on pancreatic cancer cells [Citation1]. However, the ALA has the non-specific nature, so such therapies are often related to significant off-target effects.
Microbubbles (MBs) are small gas bubbles with the diameter from 1 to 10 μm, they are stabilized by the lipid, polymer or protein shell [Citation2]. The MBs are safe and used as the ultrasound contrast agents, the size guarantees their passage through the lung capillaries [Citation3,Citation4]. The MBs undergo both inertial and stable acoustic cavitations in response to ultrasound pulses [Citation5]. They can cause the cell membrane disruption and facilitate intracellular drug uptake at the target site [Citation6]. The SDT and MBs combination could significantly increase the cytotoxic effect on human breast cancer cells [Citation7]. We aimed to make lipid and poly(lactic-co-glycolic acid) (PLGA) MBs as the carrier of sonosensitizer ALA and evaluate the effect of ALA-loaded MBs on SDT in pancreatic cancer cells in vitro and in vivo.
Materials and methods
Reagents
The ALA, 3-(4,5-dimethyl-2-thiazolyl)-2,5-diphenyl-2-H-tetrazolium bromide (MTT) and FITC-annexin V apoptosis detection kit were purchased from Sigma-Aldrich (St. Louis, MO). Trypsin–EDTA solution and reactive oxygen species (ROS) kit were obtained from Beyotime Biotechnology (Haimen, Jiangsu, China). Foetal bovine serum (FBS) and RPMI 1640 medium were from Hyclone Laboratories (Logan, UT, USA). PLGA (lactide:glycolide = 50:50, Mw = 5–15 kDa) and poly(vinyl alcohol) (PVA, 98–99% hydrolysed, Mw = 31,000–50,000) were from Jinan Daigang Biomaterial Co., Ltd. (Jinan, China). Sulphur hexafluoride MBs were named SonoVue (Bracco Suisse SA, Geneva, Switzerland). Moreover, 1,2-distearoyl-sn-glycero-3-phosphoethanolamine-N-[methoxy(polyethylene glycol)-2000] (DSPE) and 1,2-distearoyl-sn-glycero-3-phosphocholine (DSPC) were obtained from Avanti Polar Lipids (Alabaster, Alabama, USA).
Cell culture
HPDE6-C7, AsPC-1 and BxPC-3 cells from American Type Culture Collection (ATCC, Manassas, VA, USA) were cultured in RPMI 1640 medium supplemented with 100 U/mL penicillin, 0.1 mg/mL streptomycin and 10% FBS. Cultures were maintained in a humidified incubator including 5% CO2 at 37 °C. The HPDE6-C7 and pancreatic cancer cells in the exponential growth phase were used for the experiment.
Preparation of ALA-lipid MBs
DSPE (3 mg), DSPC (7 mg) and ALA (3 mg) were dissolved in methyl alcohol (2 mL) and methylene chloride (4 mL). The solvent was shifted to a beaker and evaporated in thermal water at 60 °C. The lipids thin films was mixed well at 2 mL of phosphate-buffered saline (PBS), the liposome was aliquoted at 1 mL in 2 mL cylindroid tubes with rubber hats. Afterwards, the air in the tubes was substituted by perfluoropropane gas with a injector across the rubber hats. The tubes were automatically oscillated for 45 s in a dental amalgamator, we got ALA-lipid MBs. The free drug (not contained into the MBs) was separated from the resultant white mixture by centrifugal flotation. The lipid MBs without the addition of ALA (lipid MBs) was equally prepared.
ALA-PLGA MBs preparation
PLGA (100 mg) was dissolved in 4 mL methylene chloride and mixed with 0.4 mL ALA solution (75 mg/mL, 30 mg ALA dissolved in 0.4 mL deionized water). To form the first emulsion, C3F8 gas was added to the polymer solution and sonicated in an ice water bath, the applied power using a sonicator probe (25 kHz, LC-1000, Ningbo, China) was with 150 W, 10 pulses of 3 s separated by 1 s pauses. Then, the emulsion mixture was dispersed within 40 mL of a 2% (g/100 mL) PVA solution, the solution was homogenized for 20 min at 9000 rpm to shape into the second emulsion. After homogenizing, 2% isopropyl alcohol (10 mL) was added to the emulsion and stirred for 2 h to let the methylene chloride disappear. The ALA-PLGA solution was centrifuged (3000×g, 5 min) and the epipelagic solution was dumped. The deionized water was added, mixed and then centrifuged again. This process was repeated twice. Ultimately, the microspheres were freeze dried for 48 h and the resulting samples were stored in a icebox at 4 °C for further use, we obtained the ALA-PLGA MBs. Compared with the ALA-PLGA MBs, 0.4 mL ALA solution was replaced by 0.4 mL deionized water, PLGA MBs without ALA (PLGA MBs) were prepared.
MBs characterization
The morphology characterization of ALA-lipid MBs and ALA-PLGA MBs was observed by IX71 inverted microscope (Olympus, Tokyo, Japan). The ALA-PLGA MBs with a shell were sputter-coated with gold using a vacuum evaporator, examined at 5 kV accelerating voltage and observed by scanning electron microscopy (KYKY-2000; Beijing, China). The size distribution and average size were tested by dynamic light scattering (DLS, NICOP 380ZLS, Santa Barbara, CA, USA).
The amount of ALA incorporated in the MBs was determined by a TU-1901 dual beam UV-vis spectrometer (Beijing, China). A series of ALA standard solutions with the concentration of ALA at 0.125, 0.25, 0.5, 1, 1.5 2, 3 and 6 mg/mL were prepared to construct standard curve. ALA encapsulation efficiency and loading efficiency was determined in triplicate by determining the amount of free ALA during preparation of ALA-lipid MBs and ALA-PLGA MBs at a 264 nm excitation wavelength. Drug effective load was calculated as the difference between the total ALA and free ALA. ALA loading efficiency and encapsulation efficiency were then calculated.
Biocompatibility of lipid MBs and PLGA MBs
The normal pancreatic ductal epithelial HPDE6-C7 cells (5 × 103 per well) were seeded in the 96-well culture plate. After incubation for 12 h, the lipid/PLGA MBs without ALA or lipid/PLGA MBs with ALA were incubated with the cells for 24 h, the lipid/PLGA MBs to cell ratio was 5:1, 10:1 and 20:1. The treated cells were incubated for 20 h before cell viability test using MTT assay. After addition of 12.5 μL MTT to each well for 4 h, dimethyl sulphoxide was added. Optical density was tested by an ELx800 microplate reader (BioTek, Winooski, VT, USA) at 450 nm. The blank wells without cells were compared to the other hole. The experiments were repeated at least three times under the same conditions.
Cytotoxicity of lipid MBs and PLGA MBs on pancreatic cancer cells
Cells were seeded in a 96-well plate at a concentration of 5 × 103 cells per well for 12 h. The lipid/PLGA MBs without ALA or lipid/PLGA MBs with ALA were added to the cells for 24 h, the lipid/PLGA MBs to cell ratio was 5:1, 10:1 and 20:1. The cells viability was measured by MTT method 24 h after various experimental factors exposure.
SDT device
We utilized the Dundex intelligent dual-frequency ultrasound therapy device (Shenzhen, China) to modify the SDT system. The ultrasonic transducer (35 mm diameter, 60% duty cycle, 100 Hz pulse repetition frequency, 3 MHz resonance frequency, ultrasound of intensity 2 W/cm2) was under a degassed water bath (8 cm). The 35 mm culture dishes with the cultured cells was placed in a degassing water bath for 10 min. The ultrasound device was calibrated utilizing an UPM-DT-10 AV ultrasonic power meter (Ohmic Instruments, Easton, MD).
Effect of ALA-SDT on pancreatic cancer cells
The AsPC-1 and BxPC-3 cells (3 × 105 cells) were cultured in the 35 mm tissue culture dishes for 12 h. The cells were incubated with 2.2 µM ALA for 3 h and observed by confocal microscope.
The cells at a concentration of 5 × 103 cells per well were seeded in the 96-well plate for 12 h. The cells were incubated with complete RMPI 1640 medium (control) or 2.2 µM ALA for 3 h. The internal diameter of each 96-well cell culture plate was about 6.4 mm, at least four wells of the 96-well plates was placed and completely exposed to the 35 mm ultrasonic transducer. The cells were sonicated (60% duty cycle, 3 MHz resonance frequency, 100 Hz pulse repetition frequency, ultrasound of intensity 2 W/cm2) for 1 min, 3 min, 5 min, 10 min or 15 min in the dark. The viability was measured 24 h after various experimental factors exposure.
Effect of ALA-lipid/PLGA MBs mediated SDT on pancreatic cancer cells
The cells at a concentration of 5 × 103 cells per well were seeded in the 96-well plate for 12 h. They were incubated with complete RPMI 1640, lipid/PLGA MBs, ALA or ALA-lipid/PLGA MBs for 3 h, the ratio of lipid/PLGA MBs or ALA-lipid/PLGA MBs to cells was 20:1. The amount of ALA in single ALA group was equal to the ALA contained in the group of ALA-lipid/PLGA MBs. Ultrasound treatment (60% duty cycle, 100 Hz pulse repetition frequency, 3 MHz resonance frequency, ultrasound of intensity 2 W/cm2) for 10 min in the dark was applied. The cells viability was measured 24 h after different experimental factors exposure.
Cell death analysis by flow cytometry
The AsPC-1 and BxPC-3 cells at a concentration of 3 × 105 cells per well were plated into the 35 mm culture dishes for 12 h. The cells were exposed to RPMI1640 complete growth medium (control), lipid/PLGA MBs, ALA or ALA-lipid/PLGA MBs. The ratio of lipid/PLGA MBs or ALA-lipid/PLGA MBs to cells was 20:1, the amount of ALA in single ALA group was equal to the ALA contained in the group of ALA-lipid/PLGA MBs. The cells were treated by ultrasound (60% duty cycle, 100-Hz pulse repetition frequency, 3 MHz resonance frequency, ultrasound of intensity 2 W/cm2) for 10 min in the dark. After incubation for 20 h, the cells were suspended in the binding buffer, mixed with FITC-annexin V and propidium iodide (PI). AsPC-1 or BxPC-3 cells were incubated at room temperature for 20 min, the cell death rate was studied using flow cytometry.
Transmission electron microscopy
The AsPC-1 and BxPC-3 cells (3 × 105 cells) were plated into the 35 mm tissue culture dishes and cultured for 12 h. The cells were exposed to RPMI 1640 complete growth medium, lipid/PLGA MBs, ALA and ALA-lipid/PLGA MBs for 3 h, the ratio of lipid/PLGA MBs or ALA-lipid/PLGA MBs to cells was 20:1. The amount of ALA in single ALA group was equal to the ALA contained in the group of ALA-lipid/PLGA MBs. Ultrasound treatment (60% duty cycle, 100 Hz pulse repetition frequency, 3 MHz resonance frequency, ultrasound of intensity 2 W/cm2) was applied in the dark for 10 min. After incubated for 6 h, the AsPC-1 and BxPC-3 cells were fixed with glutaraldehyde, dehydrated and embedded. The ultrathin sections were prepared using Recher-Rongchao ultramicrotome. The sections were placed on formvar-coated slot copper grids and counter stained using uranyl acetate. The ultrastructure morphology of the pancreatic cancer cells was investigated by H7650 transmission electron microscope (Hitachi Ltd., Tokyo, Japan).
ROS production
The cells cultured on the glass bottom cell culture dishes (NEST Scientific, Wuxi, China) at 3 × 105 cells per dish were incubated with 10 µM DCFH-DA (diluted with serum-free RPMI1640) for 20 min or 50 µg/mL Rosup for 20 min at 37 °C, followed by washing with the serum-free RPMI1640 for three times. The intracellular ROS levels were detected by an LSM 800 laser scanning confocal microscope (Carl Zeiss Microscopy, White Plains, NY, USA) at 488 nm excitation and 525 nm emission wavelengths. ROS was quantified using the ZEN 2.3 software (blue edition, Zeiss, Oberkochen, Germany).
In vivo test and histology examination
The in vivo experiments were performed in compliance with the criteria of the National Regulation of China for Care and Use of Laboratory Animals and were approved by the Ethics Committee of Harbin Medical University Cancer Hospital. The tumours were built up by subcutaneous injection of 5 × 106 BxPC-3 cells into the right axilla of each 5-weeks-old nude male mouse (Beijing Weitong Lihua Experimental Animal Technical Co., Ltd., Beijing, China). When the long diameter of the tumour was about 5 mm after seven days, the tumour-bearing mice were divided into eight groups randomly (n = 6, each group) and treated by normal saline (control group), U group (the ultrasonic transducer: 35 mm diameter, 60% duty cycle, 100-Hz pulse repetition frequency, 3 MHz resonance frequency, ultrasound of intensity 2.5 W/cm2,10 min), lipid MBs (1.75 × 108/kg)/PLGA MBs (6.8 × 107/kg) group, MBs + U group, ALA (50 mg/kg) group, ALA + U group, ALA-lipid MBs (1.75 × 108/kg)/ALA-PLGA MBs (6.8 × 107/kg) group or ALA-lipid/PLGA MBs + U group. The ALA content was equal in ALA group and ALA-lipid MBs/ALA-PLGA MBs group. The mice were treated, the tumour was tested, every other day, on the 0, 2nd, 4th, 6th, 8th, 10th and 12th day, a total of seven times, the mice were sacrificed on the 14th day.
Histology examination
Tumours of all groups were separated and sliced. After dehydration using buffered formalin, ethanol of different concentrations and xylene. The dehydrated tissues were embedded, sliced for haematoxylin eosin staining and analysed for pathology.
Statistical analysis
Data were expressed as mean ± SD. The comparison between groups was analysed with Student’s t-test and one-way ANOVA.
Results
Characterizations of MBs
The appearance of all MBs was milky white. shows a confocal microscope micrograph of lipid MBs, ALA-lipid MBs, PLGA MBs and ALA-PLGA MBs. We found these MBs had round spheres and smooth surfaces, the spheres centres were bright and empty. No significant difference in morphology was viewed between them. Scanning electron microscopy () showed the ALA-PLGA MBs exhibited a smooth and uniform spherical morphology.
Figure 1. Confocal microscope image of (a) lipid MBs, (b) ALA-lipid MBs, (c) PLGA MBs and (d) ALA-PLGA MBs. (e) SEM images of ALA-PLGA MBs. Particle size distribution of (f) lipid MBs, (g) ALA-lipid MBs, (h) PLGA MBs and (i) ALA-PLGA MBs.
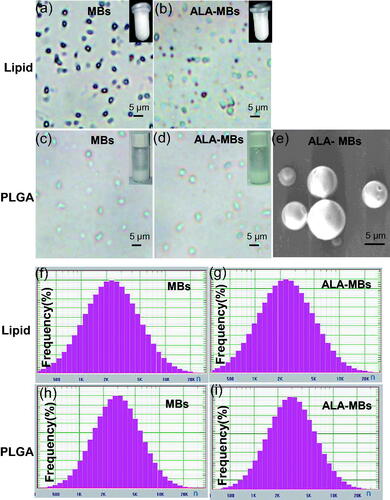
shows the average sizes of lipid MBs, ALA-lipid MBs, PLGA MBs and ALA-PLGA MBs were 3051.2 nm, 3091 nm, 3748.1 nm and 3772.9 nm, respectively. The MBs containing the inner space and the shell are suitable for taking drugs [Citation8]. We successfully prepared the ALA-lipid/PLGA MBs, the activity of photosensitizer would reserve during ultrasound-induced MBs destruction. In ALA-lipid MBs, the drug entrapment efficiency was 74.29 ± 2.60% and the drug-loading capacity was 17.14 ± 0.60%. In comparison, in ALA-PLGA MBs, the entrapment efficiency and loading capacity of the drug were 76.77 ± 1.64% and 18.75 ± 0.43%, respectively.
Biocompatibility and cytotoxicity analysis
The ratio of lipid/PLGA MBs or ALA-lipid/PLGA MBs to HPDE6-C7 cells was 5:1, 10:1, 20:1, the cell viability of all groups was almost 100% (), it indicated the lipid/PLGA MBs or ALA-lipid/PLGA MBs were not toxic to HPDE6-C7 cells when the lipid/PLGA MBs or ALA-lipid/PLGA MBs to cell ratio was 5:1 to 20:1.
Figure 2. The ratio of lipid MBs or ALA-lipid MBs (a), PLGA MBs or ALA-PLGA MBs (b) to HPDE6-C7 cells was 5:1, 10:1, and 20:1. The ratio of (c) lipid MBs or ALA-lipid MBs to AsPC-1 cells, (d) PLGA MBs or ALA-PLGA MBs to AsPC-1 cells, (e) lipid MBs or ALA-lipid MBs to BxPC-3 cells and (f) PLGA MBs or ALA-PLGA MBs to BxPC-3 cells, was 5:1, 10:1, and 20:1.
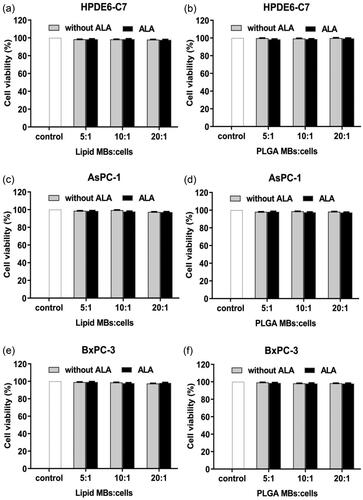
Lipid/PLGA MBs and ALA-lipid/PLGA MBs were added to the cells (the ratio of MBs to cells was 5:1, 10:1, 20:1, ). In comparison with the control groups, as the ratio of lipid/PLGA MBs and ALA-lipid/PLGA MBs to cells increased, the cell viability of the AsPC-1 and BxPC-3 cells was almost 100%. We believed the lipid/PLGA MBs or ALA-lipid/PLGA MBs were not toxic to AsPC-1 or BxPC-3 cells when the lipid/PLGA MBs or ALA-lipid/PLGA MBs to cell ratio was 5:1 to 20:1. Thus, we chose 20:1 ratio of the lipid/PLGA MBs or ALA-lipid/PLGA MBs to cells to perform the following combination experiments.
Selection of ultrasound action time
ALA enters the AsPC-1 cells () and BxPC-3 cells (). The AsPC-1 cells () and BxPC-3 cells () in single ALA group, single ultrasound (1 min, 3 min, 5 min, 10 min and 15 min) groups, ultrasound (1 min, 3 min and 5 min) with ALA (SDT) groups did not have obvious reduction in cell viability compared to the control group (p < .05 compared to control). In ultrasound with ALA groups (U with ALA, SDT), when the ultrasound time was 10 min, the cell viability was 66.3 ± 5.4% in AsPC-1 cells, 75.6 ± 16.0% in BxPC-3 cells. In 15 min SDT groups, the cell viability was 46.3 ± 24.8% in AsPC-1 cells, 62.9 ± 19.6% in BxPC-3 cells. Hence, we chose 10 min SDT groups in the following research.
Figure 3. The (a) AsPC-1 and (b) BxPC-3 cells were incubated with 2.2 µM ALA for 3 h and observed by confocal microscope with or without the 3 h incubation with ALA. The amount of ALA is the quantity of ALA contained when the ratio of ALA loaded MBs number and cell is 20:1. (c) AsPC-1 and (d) BxPC-3 cells viability were tested 24 h after exposure to ultrasound for 1, 3, 5, 10 or 15 min. *p < .05, **p < .01 compared with the control group.
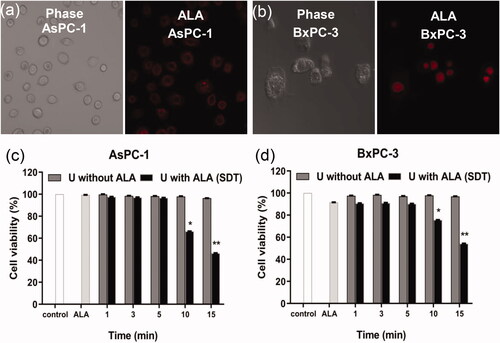
Effect of ALA loaded MBs on SDT
Compared with the control group, single ultrasound groups (U), lipid/PLGA MBs groups (without U), lipid/PLGA MBs plus ultrasound groups (with U), ALA groups (without U) and ALA-lipid/PLGA MBs groups (without U) had no significant effect on AsPC-1 or BxPC-3 cell viability (). However, compared to the control groups, the cell viability in ALA with ultrasound groups (with U) had statistically significant decrease in both AsPC-1 and BxPC-3 cells (*p < .05).
Figure 4. The survival rates of (a) ALA-lipid MBs treated AsPC-1 cells, (b) ALA-PLGA MBs treated AsPC-1 cells, (c) ALA-lipid MBs treated BxPC-3 cells and (d) ALA-PLGA MBs treated BxPC-3 cells determined by MTT. *p < .05, **p < .01, compared with the control group; #p < .05 compared with the ALA-SDT group.
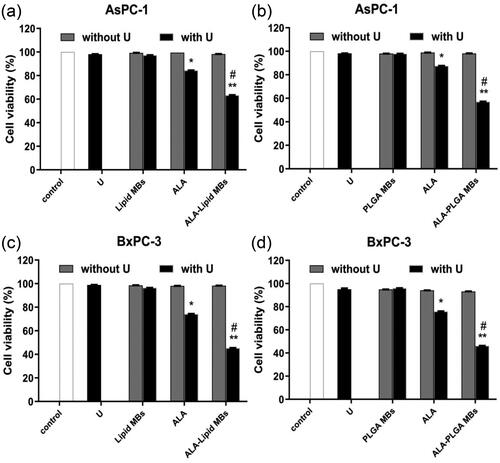
For ALA-lipid MBs with ultrasound (with U), the cell viability decreased to 63.3 ± 17.1% and 45.2 ± 25.8% (vs. control group, **p < .01) in AsPC-1 cells () and BxPC-3 cells (). In ALA-PLGA MBs with ultrasound groups (with U), the cell viability in AsPC-1 cells () and BxPC-3 cells () was 57.1 ± 20.7% and 46.2 ± 11.2% (vs. control group, **p < .01). Compared with ALA with ultrasound groups (with U), a statistically significant decrease in cell viability was observed in ALA + lipid/PLGA MBs with U in AsPC-1 and BxPC-3 cells (#p < .05).
Apoptosis assay using flow cytometry
When cells become apoptosis, FITC-labelled annexin V binds to phosphatidylserine and shows green fluorescence. PI (red fluorescence) can stain cells such as necrotic cells or cells in late stage of apoptosis that lose cell membrane integrity. The AsPC-1 and BxPC-3 cells were co-stained by FITC-annexin V and PI, apoptotic rate was tested using flow cytometry. Annexin V negative and PI positive cells were necrotic cells, annexin V positive and PI positive cells were late apoptotic cells, annexin V negative and PI negative cells represented viable cells, annexin V positive and PI negative cells were early apoptotic cells. Apoptotic rate (%)=(early apoptotic cells + late apoptotic cells)/the total cells × 100%.
The apoptotic rates of single ultrasound (U) groups, lipid/PLGA MBs groups, ALA groups, ALA-lipid/PLGA MBs groups were less than 10% in AsPC-1 and BxPC-3 cells (). Compared to single ultrasound (U) or lipid/PLGA MBs, the apoptotic rates in combination of lipid/PLGA MBs and ultrasound groups (lipid/PLGA MBs + U) had statistical difference (#p < .05). Compared with single ultrasound (U) or ALA group, the apoptotic rates in ALA and ultrasound groups (ALA + U) had statistical difference (*p < .05). The ALA-lipid/PLGA MBs + U groups had the most amount of apoptosis (&p < .05), suggesting ALA-lipid/PLGA MBs mediated SDT had obvious effect on the apoptosis of AsPC-1 and BxPC-3 cells. We found the apoptosis rates of ALA-lipid MBs + U group (49.65%) and ALA-PLGA MBs + U group (49.76%) were almost the same in BxPC-3 cells. But in AsPC-1 cells, the apoptosis rate of ALA-lipid MBs + U group and ALA-PLGA MBs + U group was 68.06% and 20.68%, respectively, it indicated that the effect of ALA-lipid MBs mediated SDT was better than ALA-PLGA MBs mediated SDT in AsPC-1 cells. The differences of apoptotic results in this study may be due to the difference between the two pancreatic cancer cell lines.
Figure 5. The apoptotic percentages of (a) ALA-lipid MBs + U treated AsPC-1 cells, (b) ALA-PLGA MBs + U treated AsPC-1 cells, (c) ALA-lipid MBs + U treated BxPC-3 cells and (d) ALA-PLGA MBs + U treated BxPC-3 cells determined by flow cytometry. #p < .05 compared with the lipid/PLGA MBs or ultrasound group. *p < .05 compared with the ALA or U group. &p < .05 compared with the ALA-lipid/PLGA MBs or U group.
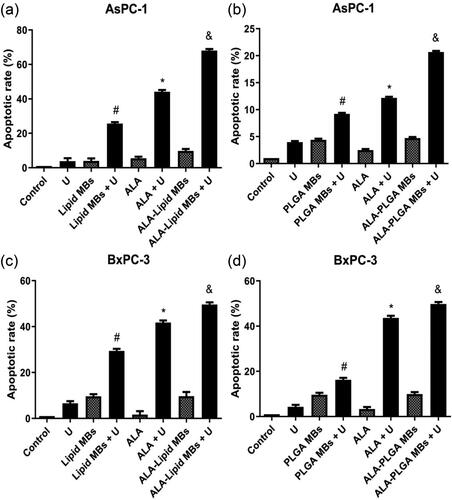
Ultrastructural morphologic changes in pancreatic cancer cells
In the control groups (), the cells possessed the irregular and distinct nucleus, simple cytoplasmic organelles, the cells had abundant microvilli covering their surface. In the ultrasound (U) groups (), lipid/PLGA MBs (MBs) groups (), lipid/PLGA MBs + ultrasound (MBs + U) groups (), ALA groups () and ALA-lipid/PLGA MBs (ALA-MBs) groups (), there were less microvilli in the AsPC-1 and BxPC-3 cells.
Figure 6. Transmission electron microscopy images of AsPC-1 and BxPC-3 cells. Cells were divided into (a) control group, (b) single ultrasound group (U), (c) lipid/PLGA MBs group, (d) lipid/PLGA MBs + U, (e) single ALA group, (f) ALA + U group, (g) ALA-lipid/PLGA MBs group and (h, i) ALA-lipid/PLGA MBs + U group. Scale bar: 2 mm. White star represented apoptosis, white triangle represented pyroptosis.
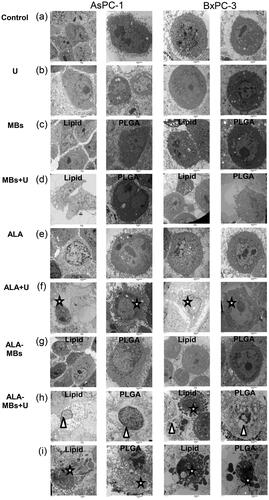
In ALA + ultrasound (ALA + U) groups (), the AsPC-1 and BxPC-3 cells showed typical characteristics of apoptosis (, white star), including disappearance of microvilli, and a small amount of apoptotic bodies. Pyroptosis, one of the most vital immune responses to danger signal and infection, is a caspase-1-dependent of programmed cell death. Pyroptosis is faster than apoptosis [Citation9,Citation10], it is characterized by cytoplasmic content released to the extracellular space and inducing strong inflammation. In the ALA-lipid/PLGA MBs + ultrasound (ALA-MBs + U) groups, we observed some of the AsPC-1 and BxPC-3 cells with typical pyroptosis (, white triangle) characteristics including microvilli disappearance, cytoplasm swelling, shrunken nucleus and without apoptotic bodies. But some of the AsPC-1 and BxPC-3 cells with typical apoptosis (, white star) characteristics including microvilli disappearance, nuclear lysis, cell membrane encapsulation into apoptotic bodies.
We found the apoptosis of cells in ALA-lipid/PLGA MBs + U groups (ALA-MBs + U, ALA-lipid/PLGA MBs-mediated SDT, in ), the apoptotic bodies of cells and the nuclear pyknosis were more obvious. Some cells showed pyroptosis in ALA-lipid/PLGA MBs and ultrasound groups (ALA-MBs + U, ALA-lipid/PLGA MBs-mediated SDT, ). MBs plus ultrasound can increase cell membrane permeability and cause cell swelling, we speculated that ALA-lipid/PLGA MBs-mediated SDT could promote pyroptosis in AsPC-1 and BxPC-3 cells.
ROS production
DCFH-DA is the cell-permeable fluorogenic stain, it can diffuse into cells and is deacetylated by cellular esterases into non-fluorescent DCFH. ROS rapidly oxidizes DCFH and the highly fluorescent DCF is generated. We detected the DCF intensity proportionally to the ROS level in AsPC-1 cells () and BxPC-3 cells (). Compared with U or ALA group, the ROS generation (% of control) in ALA plus ultrasound groups (ALA + U) were increased (*p < .05). The ALA-lipid/PLGA MBs + U groups had the most amount of ROS generation (#p < .05 compared with ALA-lipid/PLGA MBs or U group) in AsPC-1 and BxPC-3 cells, suggesting the higher intracellular ROS production in ALA-lipid/PLGA MBs mediated SDT.
Figure 7. ROS generation of (a) ALA-lipid MBs + U treated AsPC-1 cells, (b) ALA-PLGA MBs + U treated AsPC-1 cells, (c) ALA-lipid MBs + U treated BxPC-3 cells and (d) ALA-PLGA MBs + U treated BxPC-3 cells determined by MTT. *p < .05, compared with the ALA or U group; #p < .05, compared with the ALA-lipid/PLGA MBs or U group.
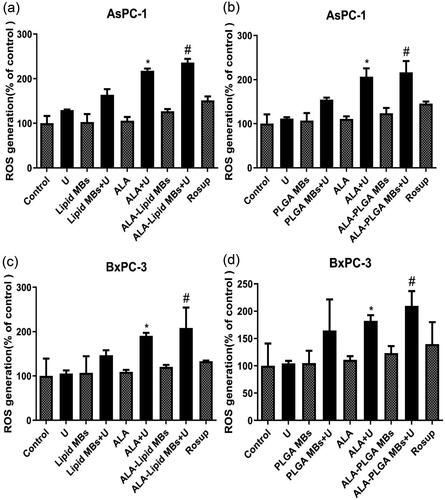
In vivo anti-tumour effects and histology examination
We found that the apoptosis rates of ALA-lipid MBs + U group and ALA-PLGA MBs + U group were almost the same in BxPC-3 cells, so we chose BxPC-3 subcutaneous xenograft model in nude mice to evaluate the anticancer efficacy of ALA-lipid/PLGA MBs mediated SDT in vivo. The mice were treated every other day, a total of seven times. With the prolonged time, the body weight of nude mice in all groups did not decrease (data not shown), indicating the little side effect of every treatment. In MBs + U group, ALA + U group and ALA-lipid/PLGA MBs + U group, the tumour growth inhibition efficacy () was better than other groups. The mice in ALA-lipid/PLGA MBs + U group had the lowest tumour volume ().
Figure 8. Tumour size and H&E stained images for BxPC-3 tumour of nude mice in different groups, (a) ALA-lipid MBs + U treatment and (b) ALA-PLGA MBs + U treatment. The BxPC-3 tumour volume of nude mice in different groups versus the treatment time, (c) ALA-lipid MBs + U treatment and (d) ALA-PLGA MBs + U treatment.
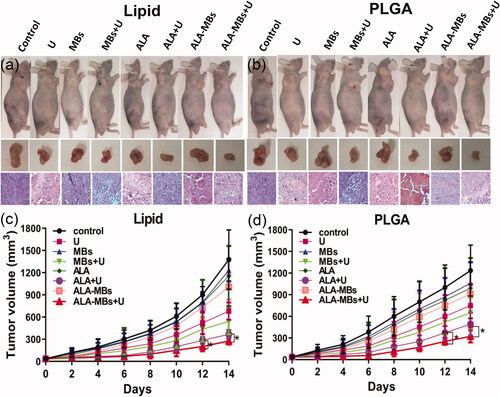
Discussion
SDT is a novel methodology that activates sonosensitizers in tumours by ultrasonic irradiation. We previously reported that SDT was a promising new way for pancreatic cancer therapy using Capan-1 cells [Citation11]. Our results were consistent with his results, but we used pancreatic cancer AsPC-1 and BxPC-3 cells.
The MBs utilized in contrast-enhanced ultrasonography were reported the promising carriers for drugs [Citation12], they play an essential role in ultrasound-mediated imaging and therapy [Citation13]. The commercial MBs named SonoVue consisting of a sulphur hexafluoride core and a phospholipidic monolayer shell are approved for routine clinical ultrasonography [Citation14]. PLGA is copolymer approved by the FDA for clinical use. We produced ALA-lipid MBs and ALA-PLGA MBs. There was no significant morphological difference between the SonoVue or ALA-lipid/PLGA MBs (data not shown). The diameter of MBs has to be less than 7 μm to pass through the pulmonary capillary wall [Citation15]. The average diameter of SonoVue is 3.0 μm [Citation13], the average size of lipid MBs, ALA-lipid MBs, PLGA MBs and ALA-PLGA MBs was about 3.0 μm. ALA-lipid MBs and ALA-PLGA MBs had good biocompatibility to normal HPDE6-C7 cells and were not toxic to pancreatic cancer cells. In the in vivo studies, it was reported that the PLGA-MBs (3.5 ± 0.6 μm) were applied for ultrasound imaging of the atherosclerotic plaques in the rabbit abdominal aorta [Citation4], the docetaxel-loaded MBs (3.387 ± 0.005 μm) were used for ultrasound imaging of the dogs’ liver [Citation16], these MBs were safe and did not cause obstruction or embolism.
Our previous research found that SonoVue MBs increase the cell viability inhibition of ALA-mediated SDT in liver cancer cells [Citation17]. Investigation showed that MBs enhanced the SDT performance in tumour [Citation18]. MBs may enhance the acoustic cavitation level and plasma membrane permeability [Citation19]. Our results showed that compared with ALA-induced SDT, the cell viability decreased in ALA lipid/PLGA MBs-induced SDT in pancreatic cancer cells. SDT kills cancer cells through generation of ROS and cavitation effect [Citation20]. We speculated that the combination of ultrasound-induced MBs destruction and MBs cavitation improved uptaking of ALA by pancreatic cancer cells, then enhanced the SDT effect via increased ROS production.
We previously found that ALA-SDT induces apoptosis of pancreatic cancer cells [Citation11]. Compared to the sonosensitizer alone, sonosensitizer and MBs conjugates improve cancer cell cytotoxicity and suppress tumour growth [Citation18]. In our study, the cell apoptosis rate enhanced in ALA loaded lipid/PLGA MBs-induced SDT in two pancreatic cancer cells. Targeting apoptosis is considered as a better modality over induction of necrosis in tumour therapy [Citation21]. After ALA-SDT action and in ALA loaded lipid/PLGA MBs mediated SDT, we observed apoptosis in pancreatic cancer cells.
Photodynamic therapy is famous for its inducing anti-tumour immune responses [Citation22]. SDT is based on photodynamic therapy. The principles, processes and photo/sono-sensitizers in the two action are similar [Citation23]. SDT induces immune responses, but the exact mechanism remains unclear. Pyroptosis is a new cell death mediated by proteases activated within inflammasomes. It is also a prominent target to mediate anti-tumour treatments [Citation24]. In ALA loaded lipid/PLGA MBs mediated SDT, we also observed pyroptosis in AsPC-1 and BxPC-3 cells. Pore formation in cell membrane is the most important event in pyroptosis [Citation24]. In the ALA loaded lipid/PLGA MBs mediated SDT group, the MBs destruction and cavitation may take part in the membrane pore formation in pyroptosis.
Excessive cellular ROS formation can lead to oxidative damage to the cell components [Citation25]. Increased ROS played an important role for SDT-related apoptosis in cancer cells [Citation26]. MBs had stimulatory function on ROS production for SDT in liver cancer cells [Citation17]. Hyperglycaemia-induced NLRP3 inflammasome activation may be an ROS-dependent process in pyroptotic cell death [Citation27]. Our results showed ALA mediated SDT (ALA + U) caused a rise in ROS generation in pancreatic cancer cells, ALA-lipid/PLGA MBs mediated SDT (ALA-lipid/PLGA MBs + U) produced much more ROS, accompanied by the features of apoptosis and pyroptosis.
Intravenous injections provide a more uniform and extensive distribution of the therapeutic effects of MBs compared with the intratumoural or intraperitoneal injections. All the mice survived during the treatment, there were no signs of pulmonary embolism in mice during the injection process. The tumour growth curve and the tumour size showed the tumour inhibitory effect of ALA-lipid/PLGA MBs mediated SDT was superior to other groups in vivo.
Conclusions
In summary, we successfully developed lipid/PLGA MBs loaded the sonosensitizer ALA to treat pancreatic cancer through the SDT approach. Ultrasound-induced ALA-loaded MBs destruction realized improved SDT efficacy. Our results showed a promise treatment in pancreatic cancer using the combination of ALA-loaded MBs and the locally focussed ultrasound in vitro and in vivo.
Disclosure statement
No potential conflict of interest was reported by the author(s).
Additional information
Funding
References
- Huang H, Wang W, Zou J, et al. Over expression of 5-aminolevulinic acid synthase 2 increased protoporphyrin IX in nonerythroid cells. Photodiagn Photodyn Ther. 2017;17:22–28.
- Geers B, De Wever O, Demeester J, et al. Targeted liposome-loaded microbubbles for cell-specific ultrasound-triggered drug delivery. Small. 2013;9(23):4027–4035.
- Ao M, Wang Z, Ran H, et al. Gd-DTPA-loaded PLGA microbubbles as both ultrasound contrast agent and MRI contrast agent—a feasibility research. J Biomed Mater Res B Appl Biomater. 2010;93(2):551–556.
- Zhao X, Zhang XY, Xue L, et al. Preparation and in vivo evaluation of ligand-conjugated polymeric microbubbles as targeted ultrasound contrast agents. Colloids Surf A Physicochem Eng Aspects. 2014;452:59–64.
- Wu J, Nyborg WL. Ultrasound, cavitation bubbles and their interaction with cells. Adv Drug Deliv Rev. 2008;60(10):1103–1116.
- Tsutsui JM, Xie F, Porter RT. The use of microbubbles to target drug delivery. Cardiovasc Ultrasound. 2004;2:23.
- Wang H, Wang P, Li L, et al. Microbubbles enhance the antitumor effects of sinoporphyrin sodium mediated sonodynamic therapy both in vitro and in vivo. Int J Biol Sci. 2015;11(12):1401–1409.
- Unger EC, Matsunaga TO, McCreery T, et al. Therapeutic applications of microbubbles. Eur J Radiol. 2002;42(2):160–168.
- Shi J, Zhao Y, Wang K, et al. Cleavage of GSDMD by inflammatory caspases determines pyroptotic cell death. Nature. 2015;526(7575):660–665.
- Wang Y, Gao W, Shi X, et al. Chemotherapy drugs induce pyroptosis through caspase-3 cleavage of a gasdermin. Nature. 2017;547(7661):99–103.
- Li YJ, Huang P, Jiang CL, et al. Sonodynamically induced anti-tumor effect of 5-aminolevulinic acid on pancreatic cancer cells. Ultrasound Med Biol. 2014;40(11):2671–2679.
- Geis NA, Katus HA, Bekeredjian R. Microbubbles as a vehicle for gene and drug delivery: current clinical implications and future perspectives. Curr Pharm Des. 2012;18:2166–2183.
- Lee H, Kim H, Han H, et al. Microbubbles used for contrast enhanced ultrasound and theragnosis: a review of principles to applications. Biomed Eng Lett. 2017;7(2):59–69.
- Bokor D, Chambers JB, Rees PJ, et al. Clinical safety of SonoVue, a new contrast agent for ultrasound imaging, in healthy volunteers and in patients with chronic obstructive pulmonary disease. Invest Radiol. 2001;36(2):104–109.
- Cosgrove D. Ultrasound contrast agents: an overview. Eur J Radiol. 2006;60(3):324–330.
- Ren ST, Liao YR, Kang XN, et al. The antitumor effect of a new docetaxel-loaded microbubble combined with low-frequency ultrasound in vitro: preparation and parameter analysis. Pharm Res. 2013;30(6):1574–1585.
- Xie R, Xu T, Zhu J, et al. The combination of glycolytic inhibitor 2-deoxyglucose and microbubbles increases the effect of 5-aminolevulinic acid-sonodynamic therapy in liver cancer cells. Ultrasound Med Biol. 2017;43(11):2640–2650.
- Nomikou N, Fowley C, Byrne NM, et al. Microbubble-sonosensitiser conjugates as therapeutics in sonodynamic therapy. Chem Commun (Camb). 2012;48(67):8332–8334.
- Skachkov I, Luan Y, van der Steen AF, et al. Targeted microbubble mediated sonoporation of endothelial cells in vivo. IEEE Trans Ultrason Ferroelectr Freq Control. 2014;61(10):1661–1667.
- Hiraoka W, Honda H, Feril LB, et al. Comparison between sonodynamic effect and photodynamic effect with photosensitizers on free radical formation and cell killing. Ultrason Sonochem. 2006;13(6):535–542.
- Rock KL, Kono H. The inflammatory response to cell death. Annu Rev Pathol. 2008;3:99–126.
- Yang Y, Hu Y, Wang H. Targeting antitumor immune response for enhancing the efficacy of photodynamic therapy of cancer: recent advances and future perspectives. Oxid Med Cell Longev. 2016;2016:5274084.
- Zhang Q, Bao C, Cai X, et al. Sonodynamic therapy-assisted immunotherapy: a novel modality for cancer treatment. Cancer Sci. 2018;109(5):1330–1345.
- Pizato N, Luzete BC, Kiffer L, et al. Author correction: omega-3 docosahexaenoic acid induces pyroptosis cell death in triple-negative breast cancer cells. Sci Rep. 2018;8(1):9775.
- Perry G, Raina AK, Nunomura A, et al. How important is oxidative damage? Lessons from Alzheimer's disease. Free Radic Biol Med. 2000;28(5):831–834.
- Song W, Cui H, Zhang R, et al. Apoptosis of SAS cells induced by sonodynamic therapy using 5-aminolevulinic acid sonosensitizer. Anticancer Res. 2011;31(1):39–45.
- Qiu Z, Lei S, Zhao B, et al. NLRP3 inflammasome activation-mediated pyroptosis aggravates myocardial ischemia/reperfusion injury in diabetic rats. Oxid Med Cell Longev. 2017;2017:9743280.