Abstract
Non-healing wounds have demonstrated aberrant regulation of several growth factors, thus using exogenous growth factors and cytokines in the clinical setting may improve the outcomes of non-healing wounds. Mesenchymal stem cells (MSCs) are the source of growth factors that show beneficial effect in promoting impaired wound healing. Certain culture condition should be developed to stimulate growth factor secretion from stem cell. Resveratrol, a small molecule found to increase MSCs therapeutic effectiveness. This study aims to investigate the effect of RV on secretion of wound healing related growth factors. We isolated and characterised MSCs from wharton’s jelly (WJ), amniotic membrane (AM), and adipose tissue. We treated MSCs with serum deprived medium (SDM) supplemented with RV at 0.1 µM, 0.5 µM, 0.8 µM concentration. Our study revealed that RV at 0.1 µM was more effective to increase cell proliferation rate. Resveratrol at 0.1 µM promoted EGF, HGF, PDGF, and TGF-β1 secretion from MSCs. AD-MSCs showed the greatest response to RV stimulation in the term of cell proliferation and growth factors secretion. As conclusion, RV can facilitate cell proliferation and wound healing related growth factors secretion at dosage dependent manner.
Introduction
Million people are affected by acute and chronic wounds as a result of surgeries, burns, infections, pressure ulcers, as well as diabetic and venous ulcers. However, chronic wounds possess a significant health burden [Citation1–4]. Wound healing is a complex biological process that involves interaction between cellular components, cytokines, growth factors, and extracellular matrix. It is generally divided into three phase: inflammation, proliferation, and remodelling [Citation5]. Numerous growth factors and their receptors are altered during wound healing phase, including platelet derived growth factor (PDGF), fibroblast growth factor (FGF), epidermal growth factor (EGF), transforming growth factor (TGF), vascular endothelial growth factor (VEGF), hepatocyte growth factor (HGF), and keratinocyte growth factor (KGF) [Citation6–8].
In vivo and in vitro studies analysing non-healing acute and chronic wounds have demonstrated aberrant regulation of several growth factors suggesting a potential target for therapy. It has led to a robust interest in using exogenous growth factors and cytokines in the clinical setting to improve the outcomes of non-healing wounds [Citation9]. In recent years, many animal studies have demonstrated a promising use of growth factors to promote impaired wound. This led to the hope that the application of exogenous growth factors may be used clinically to promote wound healing process. PDGF-BB is the only growth factor approved by the US Food and Drug administration (FDA) for clinical use, however, due to its high cost the use of PDGF-BB especially in a developing country is still debatable. Stimulation of wound healing needs crosstalk of many growth factors, hence introduction of single growth factor seems to be inadequate [Citation5,Citation8,Citation10,Citation11]. Regardless of the different growth factors therapeutic approach targeting wound healing enhancement, the search towards source of growth factors is still being explored [Citation6–8].
Mesenchymal stem cells (MSCs) are multipotent cells derived from a variety of tissues including bone marrow, adipose tissues, umbilical cord, and amniotic membrane. Recent studies proved that MSCs stimulated human dermal fibroblast (HDFs) via paracrine effects and enhanced cutaneous wound healing. MSCs secrete many cytokines and growth factors which are important in cell growth and maintaining skin tissues [Citation4,Citation12]. Several attempts have been tried to stimulate specific growth factors released by MSCs, such as manipulating culture condition by hypoxic condition, 3 D culture system, and small molecule supplementation. Resveratrol (RV, 3,5,49 – hydroxystilbene) is a small molecule, naturally occurring product found in a number of plants. Numerous studies have shown that RV possesses a wide range of biological effects that could beneficial for human health. One potential cell target for RV through oral or intravenous injection is the adult stem cells residing in different tissues and organs. Several studies within the last few years have reported the beneficial effects of RV on behaviour of various stem cells. However, limited studies have examined the effect of RV on stem cell behaviour especially in cell secretion products [Citation13,Citation14]. In this study, we hypothesised that RV could induce MSCs to secrete wound healing related growth factors.
Materials and methods
Materials
The main reagents included Type IV collagenase (Roche USA), Type II collagenase (Roche USA), alpha modified eagle medium (α-MEM, Gibco-Life Technologies, USA), dulbecco’s modified eagle’s medium (DMEM, Gibco-Life Technologies, USA), nonessential amino acid (NEAA, Sigma, USA), Trypsin-EDTA (Gibco – Life Technologies, USA), foetal bovine serum (FBS, Biowest, USA), resveratrol (Sigma, USA), bFGF (Gibco- Life Technologies, USA), 3-(4,5-dimethylthiazol-2-yl)-2,5-diphyniltetrazolium bromide (MTT; Gibco- Life Technologies, USA). Antibodies for flowcytometry CD 105, CD 90, CD 73, CD 34, CD 133, and CD 45 were purchased from BD bioscience (US). Human PDGF-B, TGF-β1, VEGF-A, EGF, and HGF ELISA kit from Bioassay Technology Laboratory, China.
Methods
This is an in vitro study to investigate the effect of various doses of RV on wharton’s jelly MSCs (WJ-MSCs), amniotic membrane MSCs (AM-MSCs), and adipose derived MSCs (AD-MSCs) secretion, mainly for PDGF-B, TGF-β1, EGF, HGF, and VEGF-A levels. All experiments were conformed to institutional ethics review board from dr. Soetomo General Academic Hospital (Registered number: 1293/KEPK/VII/2019). This study was conducted at Stem Cell Research and Development Centre, Universitas Airlangga.
Cell isolation
Wharton’s jelly and amniotic membrane MSCs isolation
Wharton’s jelly MSCs and AM-MSCs were isolated from single donor of placenta, obtained from healthy full-term baby through caesarian section under aseptic technique. Umbilical cord in 3 cm length and amnion membrane were collected after the mothers have consented. Wharton’s jelly MSCs and AM-MSCs were isolated using mixed enzymatic-explant method based on protocol developed by Azendeh et al. [Citation15] with our modification. Briefly, blood vessels were removed to allow only the WJ and AM to come into direct contact with the enzymatic solution. Wharton’s jelly and AM were minced into approximately 1 cm3 pieces and washed with PBS. Each tissue was then placed in sterile tube containing α-MEM medium with enzymatic cocktail comprising 0.75 mg/ml Collagenase Type IV and incubated at 37 °C for 30–60 min with gentle agitation. After the incubation period, the tissue pieces were washed with culture media and transferred to a new sterile tube and centrifuged at 300 g for 5 min. Supernatant was discarded and cell pellets resuspended in culture medium composed of α-MEM, 1% penicillin-streptomycin, 1% amphotericin B, 1% NEAA, 5% FBS, and bFGF 5 ng/mL for WJ-MSCs and DMEM-F12, 1% penicillin-streptomycin, 1% amphotericin B, 1% NEAA, 5% FBS, and bFGF 5 ng/mL for AM-MSCs. Cells and remaining small pieces of tissue were cultured with culture media in incubator at 37 °C and 5% CO2. Culture media were changed every 3 days until 90% confluency was reached, then passaged using warm trypsination method.
Adipose derived MSCs isolation
Adipose derived MSCs were isolated from single donor of adipose tissue, obtained from healthy 27 year-old-woman under aseptic technique. Adipose tissue in 2 × 2 cm of size was collected after assignment of informed consent. Adipose derived MSCs were isolated using enzymatic method based on protocol developed by Palumbo et al. [Citation16] with our modification. Briefly, adipose tissue was minced into approximately 1 cm3 pieces and washed with PBS. Adipose tissue was then placed in sterile tube containing α-MEM medium with enzymatic cocktail comprising 0.075% Collagenase Type I and incubated at 37 °C for 30–60 min. After the incubation period, the digested tissues were washed with culture media and transferred to a new sterile tube and centrifuged at 300 g for 5 min. Supernatant was discarded and cell pellets resuspended in culture medium composed of α-MEM, 1% penicillin-streptomycin, 1% amphotericin B, 1% NEAA, 5% FBS, and bFGF 5 ng/mL then stored in incubator at 37 °C and 5% CO2. Culture media were changed every 3 days until 90% confluency was reached, then passaged using warm trypsination method.
Cell characterization
Flow cytometry was used to assess the surface marker of MSCs, using the standard for MSC as described by the International Society for Cellular Therapy (ISCT). Cells (P2-3) were harvested, pelleted and resuspended in 1% bovine serum albumin (BSA in PBS), and counted. Each population cells were used for flow cytometry. Cells were stained with directly PE (phycoerythrin) conjugated antibodies against CD73, CD90, CD105, and CD45 (BD bioscience, US). Cells were analysed on FACS calibur flow cytometry using FACSDiva Software (BD bioscience, US).
Secretome production using resveratrol preconditioning
Amount of 5 × 103 cells/well of WJ-MSCs, AM-MSCs, and AD-MSCs at passage 4 were cultured in each 96 multiwell plate with culture media for 24 h to reach 70% confluency. A day later, the cells were treated using media composed of α-MEM, 1% penicillin-streptomycin, 1% amphotericin B, 1% NEAA, and 2% FBS supplemented with various doses of RV. We divided into three groups of RV supplementation dose that consist of 0.1 µM, 0.5 µM, and 0.8 µM. For control group, we used culture media in serum deprivation condition (SDM) containing 2% FBS. Cell secretome was collected after 48 h of treatment and filtered using 0.45um millipore. The cell secretome then stored at −80 °C until further analysis. All of the experiment groups were conducted in 4 replications.
Cell viability
3-(4,5-Dimethylthiazol-2-yl)-2,5-diphyniltetrazolium bromide assay were conducted to determine the effect of RV at various dose on cell viability. After 48 h of resveratrol preconditioning, 10 µl of 0.45 mg/mL tetrazolium solution was added to each well and incubate at 37 °C for 4 h. After incubation, 100 µl of solubilisation solution were added to dissolve the formazan crystals. The plate then immediately analysed using microplate reader at absorbance of 570 nm.
Growth factor measurement
Growth factor measurement was conducted to determine the effect of RV at various doses which in the concentration of PDGF-B, TGF-β1, EGF, VEGF, and HGF. Enzyme-linked immunosorbent assay was performed according to Bioassay Technology Laboratory protocol. Briefly, cell supernatants were collected and centrifuged at 3000 rpm for 20 min. Amount of 40 µl of supernatants were placed into ELISA well plate. Biotinylated antibody and streptavidin HRP were added, then sample were incubated at 37 °C for 60 min. Samples were washed 3x with washing buffer, then solution A and B were added and incubated at 37 °C for 10 min. Stop solution was added and plate was read immediately using microplate reader at absorbance of 450 nm.
Statistical analysis
The data of cell viability and growth factor level were presented in mean ± standard deviation. Difference of cell viability among groups was analysed with ANOVA and Post-hoc Tukey using SPSS software version 19.0, p < .05 considered to be statistically significant.
Results
Cell isolation and characterisation
We successfully isolated WJ-MSCs, AM-MSCs, and AD-MSCs which fulfilled The International Society for Cellular Therapy criteria for MSCs such as fibroblast-like morphology appearance (), adhere to culture dishes, and positive expression for surface marker of MSCs. Flowcytometry of cell populations at passage 3 indicated positive expression for CD 105, CD 90, and CD 73 FITC antibody but negative for CD 34, and CD 45 antibody as depicted in . Identification of CD73 and CD90 within gate R3 showed subset of positive cells in amniotic membrane (87.42% and 92.36%), wharton’s jelly (87.41% and 89.2%), adipose tissue (89.46% and 91.2%). Identification of CD105 within gate R5 showed subset of positive cells in amniotic membrane 67.42%, wharton’s jelly 59.2%, adipose tissue 61.2%. Identification of CD34 and CD45 within gate R4 showed subset of positive cells in amniotic membrane (15.2% and 8,36%), wharton’s jelly (17.1% and 9.2%), adipose tissue (16.16% and 10.2%).
Figure 1. Fibroblast like morphology of MSCs derived from (a) wharton’s jelly, (b) amniotic membrane, (c) adipose tissue, cells at passage-3 with 90% confluency (Inverted microscope, 100x magnification).
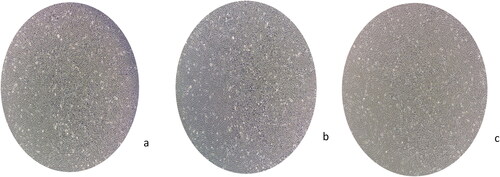
Figure 2. Identification of small CD73, CD90, CD105, CD45, CD34 cell population derived from human amniotic membrane (a), wharton’s jelly (e), and adipose tissue (i) that behave as MSCs in culture using flowcytometry. Using a five-antibody/viability marker panel, we identified a subset of small cells that were positive for CD73, CD90, CD105. (a,e,i) cells were initially displayed on SSC density plot (R2), which was subgated onto antigen plot (b,f,j) for identification of CD73 CD90 (gate R3), (c,g,k) subset of small cells were further subgated and negative for CD34 CD45 (R4) cells were further subgated to display cluster of CD105 (gate R5) (d,h,l).
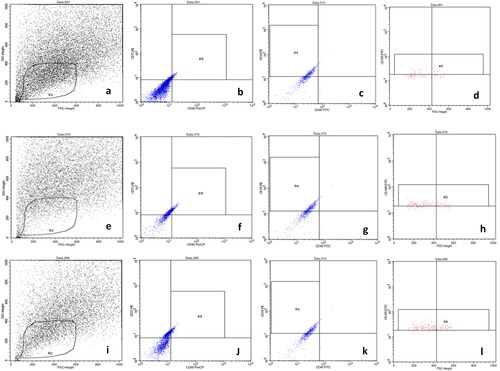
Resveratrol exerts dosage-dependent effect of MSCs proliferation
During 48 h culture of WJ-MSCs, AM-MSCs, and AD-MSCs, it was visually noted that cells treated with 0.1 µM RV expand faster than cells treated with serum deprivation media (SDM) and higher concentrations of RV, respectively. shows that while 0.1 µM RV seemed to provide the best growth advantage over SDM treated cells. AD-MSCs showed the highest proliferation rate in response to 0.1 µM RV treatment compared to AM-MSCs and WJ-MSCs. However, higher RV concentrations showed slight growth advantage over SDM treated cells.
Figure 3. Resveratrol exerts dosage dependent effect on the proliferation rate of MSCs. Cells were plated at equal density and cultured in different concentrations of resveratrol for 48 h and assessed using MTT assay. Blue bar: AD-MSCs, red bar: AM-MSCs, yellow bar: WJ-MSCs, Error bars represent standard deviation (four replications in each treatment condition; SDM: serum deprived media). *ANOVA, post-hoc Tukey, p < .05 compared to SDM treated cells.
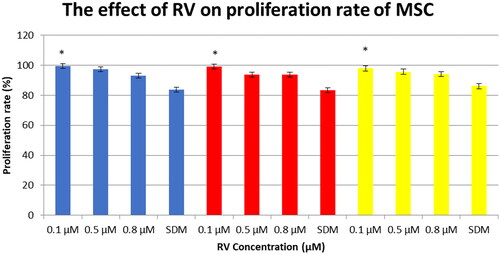
Resveratrol stimulate growth factors secretion from MSCs at dosage dependent manner
To determine the effect of resveratrol on MSCs secretion, we collect the cell supernatant after 48 h of RV treatment and measured the growth factors concentration using direct ELISA. Enzyme linked immunosorbent assay showed that the concentration of PDGF-B, TGF-β1, EGF, HGF, and VEGF in cell supernatant are varied among RV treatment (). reveals stimulation effect of RV on growth factors secretion compared to SDM treated cells.
Figure 4. Resveratrol exerts dosage dependent effect on growth factors secretion of MSCs. Cells were plated at equal density and cultured in different concentrations of resveratrol and cell secretome was collected after 48 h and assessed using ELISA. Error bars represent standard deviation (four replications in each treatment condition; SD: serum deprivation).
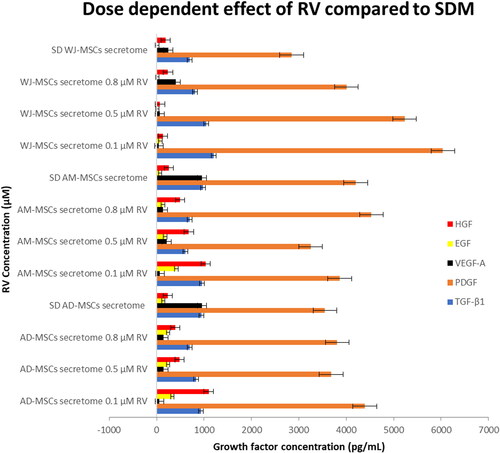
Table 1. Resveratrol induce secretion of wound healing related growth factors at dosage dependent.
0.1 µM RV significantly promoted EGF, HGF, PDGF-B, and TGF-β1 from WJ-MSCs (p < .01). At 0.1 µM, RV promoted EGF and HGF secretion from AM-MSCs (p < .01). The highest level of PDGF and TGF-β1 secretion form AM-MSCs was found at 0.8 µM RV and SDM treatment, respectively, however it was insignificant compared to 0.1 µM RV treated group (PDGF, p = .67 and TGF-β1, p = .984). In AD-MSCs culture, RV at 0.1 µM induced significant increment of EGF, HGF, and PDGF (p < .01) compared to SDM treated cells. We found that treated AD-MSCs with SDM showed highest level of TGF-β1 yet not significant compared to 0.1 µM treatment (p = .94). However, the highest level of VEGF secretion from WJ-MSCs, AD-MSCs, and AM-MSCs was found in SDM treated cell (p < .01).
Discussion
Our study revealed distinct and dynamic actions on self-renewal of MSCs originated from adult and foetal tissues stimulated by different concentrations of RV, as compared to a previous study. We found that RV at lower dose (0.1 µM) is more effective to increase cell proliferation rate, while higher concentration (0.5 µM and 0.8 µM) showed slight effect on cell proliferation. This result is in line with study demonstrating by Peltz et al. [Citation13] which showed that resveratrol is the most beneficial to stem cell development at 0.1 µM. In another study, Komaratih et al. showed that the highest proliferation rate of WJ-MSCs was reached with 0.1 µM RV supplementation [Citation17]. Increased cell proliferation rate would suggest increased representation of S and/or G2/M phase cells in RV treated cells. Peltz et al. demonstrated that at low concentration (0.1 µM), RV promotes self-renewal of AD-MSCs by reducing cell senescence rate and increasing cell proliferation rate as well as reducing cell population doubling time. However, at high concentrations, RV inhibits self-renewal of AD-MSCs by significantly increasing cell senescence rate and cell population doubling time [Citation13]. Yoon et al. showed that RV (0.1 and 1 µM) promoted silent information regulator (SIRT1) activation by suppressing SOX2 acetylation which contributing in cell self-renewal, multipotency, and differentiation [Citation18]. On the other hand, the study recently indicated that RV at higher concentration (50 µM) could stimulate cell proliferation of human embryonic stem cells (ESCs) through cell cycle modulation and enhancement in expression of anti-apoptotic markers [Citation14,Citation19]. Our study revealed that at 0.1 µM concentration, RV exerts the optimal effect in promoting cell proliferation rate in MSCs derived from WJ, AM, and adipose origin. However, the mechanism underlying the biphasic dose response on the regulation of cell proliferation is unclear and would be a great interest for future studies.
Research which was conducted by Kim et al. reported that umbilical cord stem cells conditioned media (UCS-CM) secreted EGF, bFGF, HGF, and PDGF in high amount [Citation12]. In addition, human dermal fibroblasts (HDFs) showed higher proliferation rate and collagen I production when was cultured in USC-CM than ADCS-CM. Other studies have shown that WJ-MSCs can improve the microcirculation and microenvironment significantly through therapeutic paracrine effects in a radiation rat model [Citation12,Citation20]. Sun et al. reported that WJ-MSCs CM promoted wound healing with smaller scar formation in radiation-induced skin wound rat [Citation21]. Natallya et al. demonstrated complete healing in 72.7% subjects with chronic plantar ulcer related to leprosy after topical application of human AM-MSC secretome gel within 2 months period [Citation4]. However, the limited survival of transplanted MSCs reduces the therapeutic effectiveness of MSCs [Citation22]. Hence, strategies must be developed to improve the clinical effectiveness of MSCs. One of the common strategies that improve the therapeutic effects of stem cell transplantation is preconditioned stem cells with small molecules. Small molecule drugs have a protuberant beneficial effects compared to genetic manipulation, as it can be optimised by adjusting their concentration, duration time of exposure, and composition [Citation23]. Resveratrol generally known as a small molecule which promoted MSCs proliferation, maintained differentiation, and delayed MSCs senescence [Citation13,Citation18,Citation19,Citation24]. In this study, we adopted strategy of preconditioning MSCs with RV at various concentrations to promote secretion of wound healing related growth factors as the candidate for cell-free based therapy instead of cell therapy itself. Our study found that RV at 0.1 µM could promote secretion of EGF, HGF, PDGF, and TGF-β1 from WJ-MSCs, however, those levels of growth factors were lower compared to AM-MSCs and AD-MSCs. We found that 0.1 µM is the most effective dose of RV to stimulate EGF, HGF, PDGF, and TGF-β1 from AM-MSCs and AD-MSCs. Our study revealed that AD-MSCs showed the greatest response to RV stimulation in the term of cell proliferation and growth factor secretion. However, AM-MSCs also showed a good response to RV stimulation.
Various types of growth factors play an essential role in the complex process of wound healing and tissue regeneration [Citation2,Citation5,Citation9]. We found that RV, however, increases secretion of growth factors at dosage-dependent manner and tissue-specific origin of MSCs. However, the pathways that may contribute to this effect have yet to be confirmed in this study. Epidermal growth factor, a 6-kDa molecule was obtained in relative low concentration while MSCs cultured in standard culture condition. We found that RV could increase EGF secretion from MSCs. Epidermal growth factor plays important role in completing wound healing process through increasing the rate of epithelialization and reducing the scar formation by preventing excessive wound contraction. Epidermal growth factor coated wound dressing applied to full-thickness skin wound of rabbit resulted in almost normal histological appearance and faster wound healing [Citation9,Citation25,Citation26]. We found the lowest secretion of VEGF while MSCs was treated with lower dose of RV (0.1 µM). Vascular endothelial growth factor a homodimeric 34–46 kDa heparin-binding-glycoprotein, seems to be the strongest stimulation of angiogenesis during wound healing process. However, we found that RV could promote HGF secretion from MSCs in high amount of concentration [Citation27]. Hepatocyte growth factor has a main role in regulating cell growth, motility and morphogenesis in cells such as epithelial and endothelial cells. Thus, it is directly related to epithelial repair, granulation tissue formation and neovascularization. Additionally, a synergistic effect between HGF and VEGF over endothelial cells has been demonstrated providing a more intense angiogenic effect at the wound area [Citation8,Citation9,Citation28,Citation29].
Our study showed that 0.1 µM RV was the most effective concentration to promote the secretion of wound healing related growth factors. SIRT1 is thought to be critical players in prolonging life span that consistently demonstrated altered expression in response to RV at 0.1 µM. Downregulation of SIRT1 was found with the treatment of RV at concentration greater than 0.1 µM. The relative changes correlate to higher senescence rate and percentage of S phase cells in response to high concentration resveratrol treatment. Thus, we assumed that in senescence condition and entering of S phase, cells will decrease the secretion of wound healing related growth factors and other developmental growth factors. Taken together, SIRT1 is involved in wound healing processes and its activation provides an approach for acceleration of wound therapy. Although the studies of SIRT1 regulating wound healing related growth factor have not been reported, studies of SIRT1 regulating VEGF and IGF have been reported [Citation28–30].
Our study revealed that RV could enhance PDGF secretion form MSCs. However, the role in promoting growth factors such as EGF, HGF, PDGF, and TGF-β is still unclear. Study reported that SIRT1 may be involved in RV enhancing MSCs PDGF secretion [Citation31,Citation32]. PDGF plays a role in each stage of wound healing. PDGF works in orchestra with specific cells to stimulate other growth factors secretion. Levels of PDGF are decreased in chronic wounds. Hence, recombinant human variants of PDGF-BB (Becaplermin) have been successfully applied in diabetic patients and it is the only FDA approved drug for chronic wound treatment [Citation4,Citation9,Citation33,Citation34]. The TGF-β1 is the predominates TGF-β in cutaneous wound healing. In this study, we found that RV at 0.1 µM enhanced the highest secretion of TGF-β1 from MSCs. However, serum deprivation condition promoted the highest secretion of TGF-β1 among AD-MSCs and AM-MSCs group. Transforming growth factor-β1 (TGF-β1) is a multifunctional cytokine contributed in growth and differentiation of MSC while inhibiting growth of most other cell types. TGF-β1 protects against serum deprivation-induced apoptosis of MSCs. In wound healing, TGF-β1 is important in inflammation, angiogenesis, re-epithelialization, and connective tissue regeneration. This growth factor coordinates the spatio-temporal control of wound healing. In the case of delayed cutaneous ulcer healing, the dysregulation of TGF-β1 activity leads to wound dehiscence, consequently introducing external TGF-β1 is one of the alternatives to promote wound healing [Citation4,Citation9,Citation35–37].
As limitation, our study is a lack of full understanding of RV dynamic action on growth factors regulation attributing to growth promotion. The mechanism underlying the dose response on growth factors secretion is unclear and would be of great interest for future studies. Future studies would be needed to fully elicit the potential effect of RV preconditioned MSC-CM on wound healing acceleration.
Conclusion
In summary, RV is a potential natural agent for regulating self-renewal and growth factors secretion of various stem cells. Accordingly, RV can facilitate cell proliferation and wound healing related growth factors secretion. This different effect of RV may be explained by various factors like concentration, time and duration of treatment, culture conditions, as well as the stem cell origin. Our results suggested that 0.1 µM RV was the most effective concentration to promote secretion of wound healing related growth factors.
Disclosure statement
We have no conflict of interest in this present study.
References
- Posnett J, Gottrup F, Lundgren H, et al. The resource impact of wounds on health-care providers in Europe. J Wound Care. 2009;18(4):154–161.
- Demidova-Rice TH, Hamblin MR, Herman IM. Acute and impaired wound healing: Pathophysiology and current methods for drug delivery, part 2: role of growth factors in normal and pathological wound healing: therapeutic potential and methods of f delivery. Adv Skin Wound Care. 2012;25(8):349–370.
- Kiritsi D, Nystrom A. The role of TGFβ in wound healing pathologies. Mech Ageing Dev. 2018;172:51–58.
- Natallya FR, Herwanto N, Prakoeswa C, et al. Effective healing of leprosy chronic plantar ulcers by application of human amniotic membrane stem cell secretome gel. Indian J Dermatol. 2019;64(3):250.
- Theoret C. Tissue engineering in wound repair: The three “R”s-repair, replace, regenerate. Vet Surg. 2009;38(8):905–913.
- Maxson S, Lopez EA, Yoo D, et al. Concise review: role of mesenchymal stem cells in wound repair. Stem Cells Transl Med. 2012;1(2):142–149.
- Hassan WU, Greiser U, Wang W. Role of adipose-derived stem cells in wound healing. Wound Repair Regen. 2014;22(3):313–325.
- Barrientos S, Stojadinovic O, Golinko MS, et al. Growth factors and cytokines in wound healing. Wound Repair Regen. 2008;16(5):585–601.
- Yamakawa S, Hayashida K. Advances in surgical applications of growth factors for wound healing. Burns Trauma. 2019;7:10–13.
- Borena BM, Martens A, Broeckx SY, et al. Regenerative skin wound healing in mammals: state-of-the-art on growth factor and stem cell based treatments. Cell Physiol Biochem. 2015;36(1):1–23.
- Pastar I, Stojadinovic O, Yin NC, et al. Epithelialization in wound healing: a comprehensive review. Adv Wound Care. 2014;3(7):445–464.
- Kim YJ, Seo DH, Lee SH, et al. Conditioned media from human umbilical cord blood-derived mesenchymal stem cells stimulate rejuvenation function in human skin. Biochem Biophys Rep. 2018;16:96–102.
- Peltz L, Gomez J, Marquez M, et al. Resveratrol exerts dosage and duration dependent effect on human mesenchymal stem cell development. PLoS One. 2012;7(5):e37162.
- Safaeinejad Z, Nabiuni M, Peymani M, et al. Resveratrol promotes human embryonic stem cells self-renewal by targeting SIRT1-ERK signaling pathway. Eur J Cell Biol. 2017;96(7):665–672.
- Azandeh S, Orazizadeh M, Hashemitabar M, et al. Mixed enzymatic-explant protocol for isolation of mesenchymal stem cells from Wharton’s jelly and encapsulation in 3D culture system. JBiSE. 2012;05(10):580–586.
- Palumbo P, Lombardi F, Siragusa G, et al. Methods of isolation, characterization, and expansion of human adipose-derived stem cells (ASCs): an overview. IJMS. 2018;19(7):1897.
- Komaratih E, Rindiastuti Y, Wirohadidjojo YW, Dinaryati A, et al. The resveratrol increase of hepatocyte growth factor (HGF) and epidermal growth factor (EGF) levels in wharton’s jelly mesenchymal stem cells (WJ-MSCs) secretome: Toward cell free therapy in dry eye disease (DED). Biochem Cell Arch. 2019;19(2):1–9.
- Yoon DS, Choi Y, Jang Y, et al. SIRT1 directly regulates SOX2 to maintain self-renewal and multipotency in bone marrow-derived mesenchymal stem cells. Stem Cells. 2014;32(12):3219–3231.
- Wang X, Ma S, Meng N, et al. Resveratrol exerts dosage dependent effects on the self-renewal and neural differentiation of hUC-MSCs. Mol Cell. 2016;39(5):418–425.
- Isakson M, de Blacam C, Whelan D, et al. Mesenchymal stem cells and cutaneous wound healing: current evidence and future potential. Stem Cells Int. 2015;2015:831095.
- Sun JY, Zhang Y, Song X, et al. The healing effects of conditioned medium derived from mesenchymal stem cells on radiation-induced skin wounds in rats. Cell Transplant. 2019;28(1):105–115.
- Shafiq M, Jung Y, Kim SH. Insight on stem cell preconditioning and instructive biomaterials to enhance cell adhesion, retention, and engraftment for tissue repair. Biomaterials. 2016;90:85–115.
- Li W, Li K, Wei W, et al. Chemical approaches to stem cell biology and therapeutics. Cell Stem Cell. 2013;13(3):270–283.
- Lv YJ, Yang Y, Sui BD, et al. Resveratrol counteracts bone loss via mitofilin-mediated osteogenic improvement of mesenchymal stem cells in senescence-accelerated mice. Theranostics. 2018;8(9):2387–2406.
- Kim YS, Lew DH, Tark KC, et al. Effect of recombinant human epidermal growth factor against cutaneous scar formation in murine full-thickness wound healing. J Korean Med Sci. 2010;25(4):589–596.
- Haase I, Evans R, Pofahl R, et al. Regulation of keratinocyte shape, migration and wound epithelialization by IGF-1- and EGF-dependent signalling pathways. J Cell Sci. 2003;116(Pt 15):3227–3238.
- Zhang R, Yin L, Zhang B, et al. H. Resveratrol improves human umbilical cord-derived mesenchymal stem cells repair for cisplatin-induced acute kidney injury. Cell Death Dis. 2018;9(965):1–13.
- Galiano RD, Tepper OM, Pelo CR, et al. Topical vascular endothelial growth factor accelerates diabetic wound healing through increased angiogenesis and by mobilizing and recruiting bone marrow-derived cells. Am J Pathol. 2004;164(6):1935–1947.
- Tammela T, Enholm B, Alitalo K, et al. The biology of vascular endothelial growth factors. Cardiovasc Res. 2005;65(3):550–563.
- Zielins ER, Atashroo DA, Maan ZN, et al. Wound healing: an update. Regen Med. 2014;9(6):817–830.
- Kunhiraman H, Edatt L, Thekkeveedu S, et al. 2-Deoxy glucose modulates expression and biological activity of VEGF in a SIRT-1 dependent mechanism. J Cell Biochem. 2017;118(2):252–262.
- Sun LJ, Yu JW, Shi YG, et al. Hepatitis C virus core protein induces dysfunction of liver sinusoidal endothelial cell by down-regulation of silent information regulator 1. J Med Virol. 2018;90(5):926–935.
- Lian Z, Yin X, Li H, et al. Synergistic effect of bone marrow-derived mesenchymal stem cells and platelet-rich plasma in streptozotocin-induced diabetic rats. Ann Dermatol. 2014;26(1):1–10.
- Murphy PS, Evans GR. Advances in wound healing: a review of current wound healing products. Plast Surg Int. 2012;2012:190436
- Anitua E, Alkhraisat MH, Orive G. Perspectives and challenges in regenerative medicine using plasma rich in growth factors. J Control Release. 2012;157(1):29–38.
- Penn JW, Grobbelaar AO, Rolfe KJ. The role of the TGF-beta family in wound healing, burns and scarring: a review. Int J Burns Trauma. 2012;2(1):18–28.
- Papanas N, Maltezos E. Growth factors in the treatment of diabetic foot ulcers: new technologies,any promises? Int J Low Extrem Wounds. 2007;6(1):37–53.