Abstract
In this study, novel carbon dots (CDs) were discovered and separated from Artemisiae Argyi Folium Carbonisata (AAFC) aqueous extract. AAFC-CDs were characterised by a series of methods, mainly including electron microscopy, optical technology and X-ray photoelectron spectroscopy (XPS). Results displayed that AAFC-CDs with a quantum yield (QY) around 0.19% had a size distribution between 6.0 and 10.0 nm and possessed a nearly spherical shape, with a lattice spacing of 0.369 nm. In mice, AAFC-CDs reduced the tissue damage, ear frostbite, and body stiffness caused by cold, and provided energy by increasing the use of blood glucose. The mechanism may be by decreasing concentration of IL-1βk, TNF-α and reducing the rise in blood glucose levels caused by frostbite. This study is the first to indicate that CDs may be the active constituent of AAFC against frostbite, suggesting their potential for clinical applications.
Introduction
Artemisia argyi is an herbaceous plant in the Asteraceae/Compositae family. Artemisiae Argyi Folium (AAF), the leaf of A. argyi, which is named AiYe in Chinese, has been widely used as both food and herbal medicine, with a history of nearly 3,000 years. It is recorded in the book of Zhuangzi that burning AAF is a common folk custom that the Chinese began around 300 BC. After high-temperature combustion, AAF turns into a charcoal herb, named Artemisiae Argyi Folium Carbonisata (AAFC) in traditional Chinese medicine (TCM).
Medicinal use of AAFC was firstly recorded in the Prescriptions for Fifty-Two Diseases (before 186 BC) for treating anal fistulas. The Compendium of Materia Medica (Ming dynasty, 1578 AD) recorded 1,892 Chinese medicines, including more than 700 charcoal medicines. Based on the ancient literature, the Chinese Pharmacopoeia Commission selected 27 charcoal medicines, with a clear clinical efficacy and wide application, for the 2015 edition of Chinese Pharmacopoeia. AAFC is one of the charcoal medicines with a history of having been approved by physicians from ancient to modern times. According to the Chinese Pharmacopoeia Commission, AAFC is used to treat irregular menstruation, infertility, haematemesis, and diseases due to cold.
Frostbite may lead to the formation of intracellular ice crystals, which cause direct damage to cell membranes, while extracellular ice crystals alter the oncotic pressure, leading to electrolyte shifts, electrolyte concentration changes, and cell dehydration. This results in cell structure and function damage [Citation1,Citation2], lowers the enzyme activity, and slows down the energy metabolism [Citation3]. The human body responds to tissue freezing by alternating vasodilation and vasoconstriction cycles, which results in a partially thawed cycle and creates a pre-thrombotic microenvironment [Citation4]. Local vasoconstriction, hypoxia and acidosis can damage the endothelium and promote coagulation and interstitial oedema. Hypoxia, endothelial damage and local thrombosis can cause additional vasoconstriction caused by inflammatory mediators and lead to platelet aggregation and vascular thrombosis. Since inflammatory mediators peak during the rewarming process, the cycles of refreezing and rewarming will exacerbate the degree of tissue loss.
Although AAFC use has been supported by its therapeutic benefits of treating various diseases caused by frostbite, the active ingredients behind its clinical efficacy have remained unclear for thousands of years. There are some explanations in the theory of TCM, but all the views are largely speculative and untenable. Thus, the material basis and mechanism of action of AAFC remain a mystery.
In recent years, nanomaterials have captivated attention of many medical researchers for drug delivery [Citation5–8], fluorescent bioimaging [Citation9,Citation10], and biological applications [Citation11,Citation12]. Their own bioactivities [Citation13,Citation14] and pharmacological effects [Citation15–17] have also attracted attention, but the studies are still in initial stage. Studies have revealed the presence of quantum dots in the smoke from burning turmeric, and it has been proven that those have biological activity [Citation18]. Additional studies have shown that carbon dots (CDs) from Pollen Typhae Carbonisata [Citation19], Schizonepetae Herb Carbonisata [Citation20], and egg yolk oil [Citation21] effectively decreased the activated partial thromboplastin time and increased fibrinogen, indicating that carbon quantum dots have the ability to activate haemostasis.
In our ongoing research, a new type of CDs (hereinafter referred to as AAFC-CDs) has been found in AAFC, making us wonder whether this newly discovered substance is the key to unlocking the mystery of AAFC’s effect on the anti-frostbite ability. Therefore, in this study, we evaluated the role of AAFC-CDs in strengthening the anti-frostbite ability and explored the related mechanisms.
Materials and methods
Plant material and chemicals
Artemisia argyi leaves, harvested in Hubei province, the genuine producing area of AAF, were purchased from Qiancao Chinese Herbal Medicine Company (Beijing, China). Analytical-grade chemical reagents were obtained from Sinopharm Chemical Reagents Beijing (Beijing, China). Dialysis bags of 1,000-Da molecular weight cut-off (MWCO) were purchased from Beijing Ruida Henghui Technology Development Co., Ltd. (Beijing, China). All experiments were performed using deionised water (DW).
Herbal extraction
AAF was prepared according to the literature [Citation19,Citation20]. First, 30 g AAF was placed in a crucible and covered with aluminium foil before replacing the lid to form a seal, then calcined using a muffle furnace(TL0612, Beijing Zhong Ke Anbo Technology Co., Ltd., China)for 1 h at 350 °C to produce AAFC, and then cooled to room temperature. Finally, 50 g of the fine powder obtained was soaked in 1800 ml DW and boiled twice for 1 h each time until the solution turned yellowish brown. After filtering the residue, the brown product solution was concentrated, and dialysed for 72 h (1,000-Da MWCO; Millipore). The solution obtained from the dialysis membrane was concentrated and then stored at 4 °C before use. The preparation process for AAFC-CDs is shown in .
Analysis of AAF and AAFC-CDs by high-performance liquid chromatography
To evaluate changes in components, a comparative analysis of AAF and AAFC-CDs was performed using an Agilent series 1260 high-performance liquid chromatography (HPLC) instrument (Agilent, Waldbronn, Germany) equipped with an autosampler, degasser, quaternary pump, and diode-array detector. The detection conditions used for AAFC-CDs were consistent with those used for AAF [Citation22]. Initially, an aqueous solution of AAFC-CDs and a methanol extract of AAF were prepared. For the HPLC analysis, a 10 μL aliquot was injected into a Reliasil-C18 column (250 mm × 4.6 mm; Orochem Technologies, Naperville, IL, USA) and eluted at 35 °C. The mobile phase consisted of water (solvent A) and methanol (solvent B), which were both filtered through 0.22-μm cellulose acetate membrane filters (Jin Teng, Tianjin, China) prior to use. The gradient program started with 80% solvent A, followed by a linear decrease to 20% solvent A for 10 min, which was maintained for another 30 min. The flow rate was 1.0 ml/min, and the detection wavelength was 254 nm.
Characterization of CDs
The morphology of the resultant AAFC-CDs were characterized using transmission electron microscopy (TEM; Tecnai G2 20; FEI Company; USA), operating at an accelerating voltage of 200 kV. Structural details and atomic lattice fringes of CDs were examined using high-resolution transmission electron microscopy (HRTEM) (JEN-1230; Japan Electron Optics Laboratory, Japan). Spectral properties of CDs were studied using ultraviolet–visible (UV–Vis) (CECIL, Cambridge, UK) and fluorescence (F-4500, Tokyo, Japan) spectroscopies and a standard quartz cuvette. In addition, a Fourier transform infra-red (FTIR) spectrum (Thermo Fisher, California, USA) was recorded to identify organic functional groups in CDs within a spectral window of 400 to 4,000 cm−1. The surface and elemental compositions of CDs were recorded using X-ray photoelectron spectroscopies (XPS) (ESCALAB 250Xi; Thermo Fisher Scientific, USA) with a mono X-ray source Al Kα excitation (1,486.6 eV). X-ray diffraction (XRD) measurement was performed on a D8 DISCOVER Plus X-ray powder diffractometer with Cu Kα radiation.
Animals
This study was performed in accordance with the Guide for the Care and Use of Laboratory Animals and was approved by the Committee of Ethics of Animal Experimentation of the Beijing University of Chinese Medicine. Male Kunming mice (weighing 35.0 ± 2.0 g) were purchased from the Laboratory Animal Centre, Sibeifu, with a Laboratory Animal Certificate of Conformity. The mice were maintained under the following conditions: temperature, 24.0 ± 1.0 °C; relative humidity, 55–65%; and a 12 h light/dark cycle, with ad libitum access to food and water.
Mouse ice water bath experimental model
The effects of AAFC-CDs on the anti-frostbite ability were studied in a mouse ice water bath experimental model, the model imitates cycles of refreezing and rewarming. Mice were randomly divided into five groups, with six animals in each. In the control group and the model group, the mice were administered normal saline at the same volume. In the low-, medium- and high-dose AAFC-CDs groups, the mice were administered AAFC-CDs at concentrations of 3, 6, and 12 mg/kg, respectively. All treatments were performed by intraperitoneal injection. Two hours after administration, the mice were placed in ice-cold water (0 °C) for 5 min, except the control group. Blood samples and frostbitten ears were collected 12 h after administration for 3 days [Citation23].
Effects of AAFC-CDs on frostbite
Measurement of limb stiffness
Before and after the mice were kept in ice-cold water, their grip strength was measured using a rat and mouse grip strength metre (Yiyan, Ji’nan, China.). A relative reduction in grip strength was calculated, which reflected the limb stiffness in each group of mice [Citation24] and was used to evaluate the anti-frostbite ability of mice.
Cytokine measurement
Blood was collected into an Eppendorf tube, and the sample was allowed to clot overnight at 4 °C before centrifugation for 10 min at 800 × g. The serum samples were assessed for the tumour necrosis factor (TNF)-α and interleukin (IL)-1β cytokines. The enzyme-linked immunosorbent assay kits were calibrated with commercial cytokine standards and used as per manufacturer's instructions (Cloud-Clone, USA.) [Citation25].
Histology
The mice were euthanized and the ears were harvested and placed in 10% formaldehyde, followed by changing to water after 24 h. Each sample was then embedded in paraffin, split into 4-μm thick, and stained with haematoxylin and eosin (HE) [Citation26].
Blood glucose measurement as a basic physiological indicator
To explore the physiological state under frostbite and harsh conditions, we measured blood glucose in the mice as the basic physiological indicator. After overnight fasting, the animals were orally administered a glucose solution (1.0 g/kg). Two hours later, their blood glucose levels were measured as the baseline. Then, the mice were frozen at −18 °C, and their blood glucose levels were measured every 15 min. Relative changes in the blood glucose levels were calculated.
Statistical analysis
Statistical analysis was performed using SPSS, version 20.0. Non-normally distributed data were expressed as the median (quartile range). Within-group differences were assessed using a non-parametric test, while the Wilcoxon rank test was used to compare two groups. Normally distributed data and homogeneous variances were expressed as the mean ± standard deviation. Multiple comparisons were performed using a one-way analysis of variance, followed by a least significant difference test. A p-value of < .05 was considered statistically significant.
Results
Characterization of AAFC-CDs
As shown in , the HPLC profile of the AAF extract. A series of small molecular compound peaks and specific peaks of index components were observed, isochlorogenic acid, is indicated. shows an HPLC chromatogram of AAFC-CDs, and it is obvious that the small-molecule constituents that existed in AAF disappeared from the AAFC-CDs solution.
The TEM image of the AAFC-CDs revealed that the CDs were nearly spherical and evenly distributed (). The size distribution of the AAFC-CDs was in the range of 6.0–10.0 nm and conformed to normal distribution characteristics, as determined by the statistical analysis of more than 100 particles by using ImageJ software. HRTEM showed that CDs had a lattice spacing of 0.369 nm (). Moreover, showed the powder XRD pattern exhibited a distinct diffraction peak (2θ = 22.76°), which was attributed to amorphous carbons arranged in a considerably random fashion [Citation27].
Figure 3. Characteristics of AAFC-CDs. (a) TEM image and TEM size distribution of AAFC-CDs (Upper right corner). (b) HRTEM image. (c) XRD pattern. (d) UV–Vis spectrum. (e) Fluorescence spectrum. EM: emission; EX: excitation. (f) FTIR spectrum.
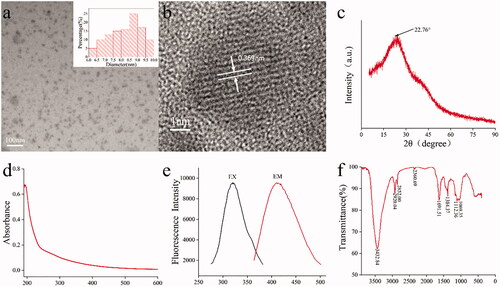
UV–Vis absorption was also analysed to determine the optical properties of AAFC-CDs. The UV–Vis spectrum () of the aqueous AAFC-CDs solution exhibited an absorption pattern with a broad absorption spectrum without any obvious peak. The fluorescence spectrum showed a maximum emission at 410 nm and maximum excitation at 322 nm in , and the QY of PRAC-CDs was calculated to be 0.19% using quinine sulphate as a reference. Fluorescence emission from carbon dots is due to the recombination of radiation between electrons and holes that are generated and trapped on the surface of the carbon dots. The different surface functional groups of carbon dots contribute to different emission states, resulting in excitation-dependent emission [Citation28, Citation29]. In order to better understand the organic functional groups on the surface of AAFC-CDs, we further analysed CDs using FTIR spectroscopies, and the spectrum of purified AAFC-CDs () showed characteristic peaks at 3,432, 2,920, 2,852, 2,360, 1,691, 1,384, 1,112, and 1,069 cm−1. The presence of O–H groups is proved by the distinct peak at 3,432 cm−1. The signal peaks at 2,920 and 2,852 cm−1 were attributed to C–H stretching, which may occur because of the association of methyl or methylene groups with the aliphatic hydrocarbons present in AAFC-CDs. The weak absorption signals at 2,360 cm−1 were attributed to C≡N stretch. Moreover, the AAFC-CDs displayed the characteristic absorption for C=O at 1,691 cm−1. The peak at 1,384 cm−1 in the AAFC-CDs spectrum was related to stretching vibrations of C=N, and a weak C–O stretching band occurs at ∼1,112 cm−1 (alkoxy). The peak at 1,069 cm−1 in the spectrum was attributed to a C–N stretch () [Citation30, Citation31]. These results imply that functional groups, including carboxyl, hydroxyl and amino groups, could exist on the surface of the AAFC-CDs.
XPS was used to determine the surface composition and chemical environments of the AAFC-CDs. XPS spectra of the C 1 s, O 1 s, and N 1 s regions of the AAFC-CDs solution were exhibited in . The purified CDs were mainly composed of the C 1 s and O 1 s elements at relative percentages of 68.67% and 21.26%, respectively. In addition, elemental N, Mg, and Ca were also present in CDs. The components in the C 1 s spectra corresponded to C–C groups (C1), C–C–O groups, C–OH groups, O–C=O, or N–C=O groups. The components in the O 1 s spectra corresponded to O=C and O–C groups. The components in the N 1 s spectra corresponded to C–N and N–H [Citation32, Citation33]. These results were consistent with the surface composition of AAFC-CDs determined by the FTIR analysis.
Effects of AAFC-CDs on frostbite in mice
Histological observations
The histology of HE-stained mouse ear tips was observed under a microscope. The ear tip frostbite necrosis was the most serious in the mice from the NS group. The cartilage between the necrotic part and the ear was broken, and they were connected by connective tissue. The border of the necrotic part is clear, with severe inflammatory cell infiltration and tissue congestion. The low-dose AAFC-CDs group also showed inflammatory cell infiltration and tissue congestion, but the epidermis was not completely necrotic, and necrosis tissue boundaries were not clear. The middle- and high-dose AAFC-CDs groups also showed inflammatory cell infiltration, but did not have obvious necrosis. Neutrophils, lymphocytes, and macrophages were the major infiltrated inflammatory cells ().
Serum cytokine concentrations
To determine the effects of AAFC-CDs treatment on frostbite, IL-1β and TNF-α were measured in mouse sera. As shown in , compared with those in the control group (36.42 pg/mL, 43.97 pg/mL), the IL-1β and TNF-α values of mice in the model group (108.76 pg/mL, 229.32 pg/mL) were significantly elevated (p < .01), indicating that the model was functional. Compared with those in the model group, the IL-1β values in the low-, medium- and high-dose AAFC-CDs groups (75.59 pg/mL, 57.12 pg/mL, 62.12 pg/mL, respectively) were significantly decreased (p < .01). Compared with those in the model group, the TNF-α values in the low-, medium- and high-dose AAFC-CDs groups (171.25 pg/mL, 99.43 pg/mL, 73.93 pg/mL, respectively) were significantly decreased (p < . 01).
Blood glucose changes under frostbite
AAFC-CDs could reduce the rise in blood glucose levels caused by frostbite (). The rise of blood glucose in the AAFC-CDs groups and control groups was not statistically different at 15 min (p > .05). After 30 min, there were statistically significant differences between the AAFC-CDs groups and the control groups (p < .05), but there were no significant differences among the low-, middle-, and high-dose AAFC-CDs groups (p > .05).
Differences in limb stiffness
The effect of AAFC-CDs on frostbite was explored using a relative change of grip strength in mice. A significant decrease in the relative grip strength was observed after AAFC-CDs treatment. The grip strength in the AAFC-CDs-treated groups was 94.46 ± 23.70 g at the high dose, 114.25 ± 13.27 g at the middle dose, and 156.72 ± 13.64 g at the low dose compared with 191.12 ± 34.99 g in the model + NS group. The differences among the groups were all statistically significant ().
Discussion
AAFC, as a traditional charcoal drug, has a long history in clinical treatment of frostbite-induced diseases; however, their active constituents are still obscure. Previous studies have attempted to explain the biological activity of AAFC from small molecules in the original plants such as flavonoids and volatile oils, but a little information was brought back. Some studies indicated that rough crystals after high-temperature combustion are the main active ingredients [Citation34]. Modern medical research [Citation35–37] revealed bioactivities and pharmacological effects of charcoal drugs from the aspect of carbon nano-particles, which inspired us to conduct this study. In the early stages of the experiment, we found that compared with AAF decoction, AAFC water extract has a fluorescence phenomenon, which enlightened us to have produced new substances during the carbonization process.
In this study, novel CDs were discovered and separated from an AAFC aqueous extract. AAFC-CDs were characterised using TEM, HRTEM, as well as FTIR, UV–Vis, and fluorescence spectroscopies. In addition, in order to assess the component changes and eliminate the interference of small organic compounds, the chemical components of AAFC extract and AAFC-CD were compared by HPLC analysis. The precursor herb of AAFC-CDs, AAF, is mainly composed of flavonoids such as isochlorogenic acid and is widely used as an herbal medicine in TCM. However, the HPLC results showed that these small-molecule constituents and other organics disappeared after calcination.
During experiments with multiple ice water bath simulated cycles of refreezing and rewarming, AAFC-CDs could effectively reduce the tissue damage and ear frostbite caused by cold, which we confirmed by HE staining, the mechanism may be by decreasing concentration of IL-1β and TNF-α. In addition, AAFC-CDs showed the ability to improve the body's tolerance to frostbite, reduce the body stiffness caused by frostbite, the mechanism may be by reducing the rise in blood glucose levels caused by frostbite, which provides energy for the body under frostbite conditions.
Conclusions
In this study, a novel material was isolated from AAFC and identified as CDs. Pharmacodynamic experiments in mice revealed that AAFC-CDs could effectively reduce the tissue damage, ear frostbite, and body stiffness caused by cold, the mechanism may be by decreasing concentration of IL-1βk, TNF-α and reducing the rise in blood glucose levels caused by frostbite. This study may provide novel strategies for studying the active components of AAFC and new ideas for the treatment of frostbite and other diseases due to cold.
Disclosure statement
The authors have no other relevant affiliations or financial involvement with any organisation or entity with a financial interest in or financial conflict with the subject matter or materials discussed in the manuscript apart from those disclosed.
Data availability statement
The data that support the findings of this study are available from the corresponding author upon reasonable request.
Additional information
Funding
References
- Murphy JV, Banwell PE, Roberts AH, et al. Frostbite: pathogenesis and treatment. J Trauma. 2000; 48(1):171–178.
- Salimi Z, Wolverson MK, Herbold DR, et al. Frostbite: experimental assessment of tissue damage using Tc-99m pyrophosphate. Work in progress. Radiology. 1986;161(1):227–231.
- Glennie JS, Milner R. Non-freezing cold injury. J R Nav Med Serv. 2014;100(3):268–271.
- George W, Rodway Scott E, McIntosh E, et al. Bradford Washburn's 1962 NEJM article “Frostbite: what it is—how to prevent it—emergency treatment”—historical background and commentary. Wilderness Environ Med. 2011;22(4):366–369.
- Kim J, Park J, Kim H, et al. Transfection and intracellular trafficking properties of carbon dot-gold nanoparticle molecular assembly conjugated with PEI-pDNA. Biomaterials. 2013;34(29):7168–7180.
- Tang J, Kong B, Wu H, et al. Carbon nanodots featuring efficient FRET for real-time monitoring of drug delivery and two-photon imaging. Adv Mater. 2013;25(45):6569–6574.
- Ye Q, Yan F, Kong D, et al. Constructing a fluorescent probe for specific detection of catechol based on 4-carboxyphenylboronic acid-functionalized carbon dots. Sens Actuators B. 2017;250:712–720:
- Hinde E, Thammasiraphop K, Duong HT, et al. Pair correlation microscopy reveals the role of nanoparticle shape in intracellular transport and site of drug release. Nat Nanotechnol. 2017;12(1):81–89.
- Atchudan R, Edison T, Lee YR. Nitrogen-doped carbon dots originating from unripe peach for fluorescent bioimaging and electrocatalytic oxygen reduction reaction. J Colloid Interface Sci. 2016;482:8–18.
- Atchudan R, Edison T, Aseer KR, et al. Highly fluorescent nitrogen-doped carbon dots derived from Phyllanthus acidus utilized as a fluorescent probe for label-free selective detection of Fe3+ ions, live cell imaging and fluorescent ink. Biosens Bioelectron. 2018;99:303–311.
- Atchudan R, Edison TNJI, Perumal S, et al. Hydrophilic nitrogen-doped carbon dots from biowaste using dwarf banana peel for environmental and biological applications. Fuel. 2020;275:117821.
- Atchudan R, Edison TNJI, Perumal S, et al. Betel-derived nitrogen-doped multicolor carbon dots for environmental and biological applications. J Mol Liq. 2019;296:111817.
- Abdelhamid HN, Talib A, Wu HF. One pot synthesis of gold – carbon dots nanocomposite and its application for cytosensing of metals for cancer cells. Talanta. 2017;166:357–363.
- Gowthaman NSK, Sinduja B, Karthikeyan R, et al. Fabrication of nitrogen-doped carbon dots for screening the purine metabolic disorder in human fluids. Biosens Bioelectron. 2017;94:30–38.
- Shan MQ, Chen X, Li J, et al. Comparative study on effects of Rubiae Radix et Rhizoma and carbonized Rubiae Radix et Rhizoma on acute blood stasis rat model. Zhongguo Zhong Yao Za Zhi. 2014;39(3):493–497.
- Shen J, Zhu Y, Yang X, et al. Graphene quantum dots: emergent nanolights for bioimaging, sensors, catalysis and photovoltaic devices. Chem Commun (Camb)). 2012;48(31):3686–3699.
- Zhuo S, Shao M, Lee ST. Upconversion and downconversion fluorescent graphene quantum dots: ultrasonic preparation and photocatalysis. ACS Nano. 2012;6(2):1059–1064.
- Chun S, Muthu M, Gansukh E, et al. The ethanopharmacological aspect of carbon nanodots in turmeric smoke. Sci Rep. 2016;6:35586.
- Yan X, Zhao Y, Luo J, et al. Hemostatic bioactivity of novel Pollen Typhae Carbonisata-derived carbon quantum dots. J Nanobiotechnology. 2017;15(1):60.
- Zhang M, Zhao Y, Cheng J, et al. Novel carbon dots derived from Schizonepetae Herba Carbonisata and investigation of their haemostatic efficacy. Artificial Cells. 2018;46(8):1562–1571.
- Zhao Y, Zhang Y, Liu X, et al. Novel carbon quantum dots from egg yolk oil and their haemostatic effects. Sci Rep. 2017;7(1):4452.
- Jia-Li WU, Wang YL, Liu W, et al. Simultaneous determination of seven constituents in Artemisia argyi leaves by HPLC. Chin Tradit Pat Med. 2017;39(9):1876–1879.
- Liu L, Duan JA, Tang Y, et al. Taoren–Honghua herb pair and its main components promoting blood circulation through influencing on hemorheology, plasma coagulation and platelet aggregation. J Ethnopharmacol. 2012;139(2):381–387.
- Fujiwara M, Iwata M, Inoue T, et al. Decreased grip strength, muscle pain, and atrophy occur in rats following long‐term exposure to excessive repetitive motion. FEBS Open Bio. 2017;7(11):1737–1749.
- Chandramoorthy HC, Bin-Jaliah I, Karari H, et al. MSCs ameliorates DPN induced cellular pathology via [Ca(2+)] i homeostasis and scavenging the pro-inflammatory cytokines. J Cell Physiol. 2018;233(2):1330–1341.
- Schmitz EJ, Herwig-Carl MC, Holz FG, et al. Sebaceous gland carcinoma of the ocular adnexa – variability in clinical and histological appearance with analysis of immunohistochemical staining patterns. Graefes Arch Clin Exp Ophthalmol. 2017;255(11):2277–2279.
- Atchudan R, Edison TNJI, Perumal S, et al. Eco-friendly synthesis of tunable fluorescent carbon nanodots from Malus floribunda for sensors and multicolor bioimaging. J Photochem Photobiol A. 2020;390:112336.
- Sharma V, Saini AK, Mobin SM. Multicolour fluorescent carbon nanoparticle probes for live cell imaging and dual palladium and mercury sensors. J Mater Chem B. 2016;4:2466–2476.
- Atchudan R, Edison TNJI, Chakradhar D, et al. Facile green synthesis of nitrogen-doped carbon dots using Chionanthus retusus fruit extract and investigation of their suitability for metal ion sensing and biological applications. Sensor Actuat B-Chem. 2017;246:497–509.
- Mewada A, Pandey S, Shinde S, et al. Green synthesis of biocompatible carbon dots using aqueous extract of Trapa bispinosa peel. Mater Sci Eng C Mater Biol Appl. 2013;33(5):2914–2917.
- Qu S, Wang X, Lu Q, et al. A biocompatible fluorescent ink based on water-soluble luminescent carbon nanodots. Angew Chem Int Ed Engl. 2012;51(49):12215–12218.
- Li Y, Li S, Wang Y, et al. Electrochemical synthesis of phosphorus-doped graphene quantum dots for free radical scavenging. Phys Chem Chem Phys. 2017;19(18):11631–11638.
- Jie S, Shang S, Chen X, et al. Highly fluorescent N, S-co-doped carbon dots and their potential applications as antioxidants and sensitive probes for Cr (VI) detection, Sens Actuators B. 2017;248:92–100.
- Yanfei HE, Zhou Q, Chen H, et al. Clinical application and research progress of carbonized traditional Chinese medicine. Amino Acids Biotic Res. 2015;37(3):1–6.
- Atchudan R, Jebakumar Immanuel Edison TN, Perumal S, et al. Indian gooseberry-derived tunable fluorescent carbon dots as a promise for in vitro/in vivo multicolor bioimaging and fluorescent ink. ACS Omega. 2018;3(12):17590–17601.
- Sindhwani S, Syed AM, Ngai J, et al. The entry of nanoparticles into solid tumours. Nat Mater. 2020;19(5):566–575.
- Wu J, Zhang M, Cheng J, et al. Effect of Lonicerae japonicae Flos Carbonisata-derived carbon dots on rat models of fever and hypothermia induced by lipopolysaccharide. Int J Nanomedicine. 2020;15:4139–4149.