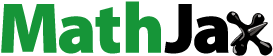
Abstract
A novel approach for the utilisation of fruit waste is attempted in the present investigation. Mangifera indica seed aqueous extract was utilised for green synthesis of silver nanoparticles (AgNPs). The phytoconstituents in the seed acted as reducing and stabilising agent for AgNP formation. UV-Vis, Zeta potential, FT-IR, XRD, TEM, SAED, EDX analysis were used to characterise the green synthesised AgNPs. UV-vis spectra showed characteristic spectra at 450 nm; XRD and SAED confirmed the crystalline nature while TEM revealed the shape to be round and average size was 26.85 nm. FT-IR revealed functional groups like alcohol or phenols, carboxylic acids, ketones, amines, aromatic amines, aliphatic amines, alkyl halides and alkynes which were responsible for AgNP formation. The nanoparticles showed more antibacterial activity than antifungal activity and antibacterial activity towards Gram-negative bacteria was more than Gram-positive bacteria. Dose dependent antioxidant activity (DPPH, SO and ABTS) and dose dependent cytotoxic effect against HeLa, MCF-7 and normal fibroblast cell lines was envisaged. The green synthesised AgNPs exhibited three different bioactivities (3-in-1 system) i.e. dose dependent antimicrobial, antioxidant and cytotoxic activity. Fruit waste can be successfully utilised for silver nanoparticles formation which can be therapeutically useful and effective.

Silver nanoparticles were synthesised from M. indica fruit waste i.e. seed
Characterisation by spectroscopic techniques: UV-Vis, Zeta, FTIR, XRD, SAED, EDX and TEM analysis.
Silver nanoparticles were 26.85 nm in size and round in shape
Antimicrobial activity against 14 microorganisms
Antioxidant activity in terms of DPPH, SO and ABTS
Cytotoxic activity against HeLa, MCF-7 and Fibroblast normal cell lines
Highlights
Introduction
Nanotechnology has applications in many fields like agriculture, dye degradation, wastewater treatment, medical applications, biosensing, drug delivery, fabric and textile industry, cosmetics, food industry, etc. [Citation1]. Nanoparticles like silver, gold, zinc, copper, palladium, platinum can be produced by physical, chemical and biological methods; however biological methods are most preferred and favoured method since physical and chemical methods have many disadvantages [Citation2]. Biological or green method makes use of plants, algae and microorganisms. Green method making use of plant parts is most simple, easy, quick, cost effective and environmental friendly. It has many added advantages. The different plant parts possess many structurally different phytoconstituents like phenols, flavonoids, saponins, glycosides, tannins, alkaloids, anthocyanins which themselves act as reducing and stabilising agent for nano particle synthesis and hence nullifies the use of toxic solvents and chemicals.
Seeds from fruits and vegetables are thrown away into the environment causing pollution; their disposal is also problematic but seeds show many pharmacological activities like antimicrobial, antioxidant, antidiabetic, anti-inflammatory, anti-ulcer, etc. [Citation3] because of various phytoconstituents present in them. Thus, this waste can be utilised therapeutically. Recently the attention has turned towards nanoparticle synthesis. The phytochemicals present in the seeds act as reducing and capping agents and are responsible for silver nanoparticles (AgNPs) formation. There are numerous examples of AgNPs synthesis from seeds of different plant sources like Alpinia katsumadai [Citation4]; Persea americana [Citation5]; Avicennia marina [Citation6].
AgNPs have a wide range of applications and also many pharmacological properties. For e.g. anticoagulant activity of seed hull of Vigna mungo [Citation7], antioxidant activity of Nepeta leucophylla root [Citation8], wound healing activity of Delonix elata leaf [Citation9], cytotoxicity activity of Iboza riparia leaf and Ilex mitis root bark [Citation10], antifungal and antibacterial activity of Amaranthus tricolour leaf [Citation11], etc. They are also used in medical devices (wound dressing, catheters, bone cement), water purification, biosensors, bioimaging, cosmetics, food preservations, cell electrodes, integrated circuits, drinking water filters, etc. [Citation12,Citation13].
Mangifera indica L. is one of the delicious tropical fruit of the world. It belongs to genus Mangifera and family Anacardiaceae. It is considered as king of fruits. It has many bioactive compounds like polyphenols, terpenes, sterols, carotenoids, vitamins and amino acids. Some of the compounds found in various parts of M. indica are Gallic acid, Mangiferin, Ascorbic acid, Quercetin, Atechin, Benzoic acid, Kaempferol, Methyl gallate, Octyl gallate, Propyl gallate, Pentyl gallate, Ocimene, Friedelin, Humulene, Elemene Epigallocatechin gallate, Isomangiferin, Linalool, β-Carotene, β-Sitosterol, Linolenic acid, etc. [Citation14]. It has many ethnomedicinal uses and pharmacological uses such as anti-inflammatory, anticancer, antidiabetic, anti-hemorrhagic, analgesic and antipyretic, anti-tetanus, anti-ulcer, antibacterial, antifungal, antiviral, anti-diarrheal, antibone resorption, antimalarial, hepatoprotective, radio protective, cardio protective, osteoporosis prevention, bronchodilatory and laxative [Citation15]. M. indica mediated synthesised AuNPs is reported by [Citation16,Citation17]; however, the efficacy of M. indica seeds to synthesise AgNPs is yet to be studied and perhaps this is the first report of using seed of M. indica for AgNP synthesis. Hence, the aim of the present work was utilisation of M. indica fruit waste (seed) for the synthesis of AgNPs, characterisation by various spectroscopic techniques and evaluation of their antimicrobial, antioxidant and cytotoxic potential (3-in-1 system).
Material and methods
Plant material
The fruits of M. indica were purchased from fruit market of Rajkot, Gujarat, India. The fruits were washed thoroughly with tap water and then peel and pulp were separated; the seeds were manually separated after breaking seed kernel, shade dried at room temperature and homogenised to fine powder and stored in air tight bottles.
Preparation of seed extract
Mangifera indica seed extract was prepared by decoction extraction method. One gram dry powder was extracted with 100 ml of double distilled water by boiling for 5 min. The extract was filtered through Whatman filter paper No. 1 and centrifuged at 10,000 rpm for 10 min. The filtrate was used for the synthesis of AgNPs.
Biosynthesis of AgNPs
AgNPs were synthesised using 1 mM AgNO3 and aqueous M. indica seed extract. 6 ml of seed extract was added to 40 ml of AgNO3 solution at room temperature (25° C ± 2° C) and incubated in dark for 24 h. This ratio gave best results hence used in the synthesis process. The nanoparticles solution was centrifuged repeatedly for 10 min at 10,000 rpm for purification followed by redispersion of the pellet of nanoparticles in 3–4 ml of acetone. The purified nanoparticles were air dried and stored at 4 °C.
Characterisation of the synthesised AgNPs
The Zeta potential was measured using the instrument Zetasizer Nano ZS (Malvern Instruments Inc., USA). The FTIR spectrum was recorded on Nicolet IS10 (Thermo Scientific, Waltham, MA) at room temperature. The IR spectra was noted in the range of 400–4000 cm−1. Different modes of vibrations were identified and assigned to determine the different functional groups present in the M. indica seed extract. The crystalline nature of synthesizd AgNPs was analysed by XRD. It was characterised by X’Pert Pro X-ray diffractometer (PAN analytical BV) instrument operated at a voltage of 40 kV and a current of 30 mA with Cu Ka radiation in θ–2θ configurations. The crystallite domain size was calculated from the width of the XRD peaks, assuming that they are free from non-uniform strains, using the Scherrer formula D¼0.94 λ/β cos θ, where D is the average crystallite domain size perpendicular to the reflecting planes, λ is the X-ray wavelength, β is the full width at half maximum (FWHM), and θ is the diffraction angle. TEM analysis was done to visualise the shape as well as to measure the diameter of the green synthesised AgNPs. The sample was dispersed in double distilled water. A drop of thin dispersion was placed on a “staining mat.” Carbon coated copper grid was inserted into the drop with the coated side upwards. After about 10 min, the grid was removed and air dried. Then screened in JEOL JEM 2100 Transmission Electron Microscope (JOEL Corp, Tokyo, Japan).
Biological activity of the synthesised AgNPs
Antimicrobial activity
The antimicrobial activity of green synthesised AgNPs was determined by Agar well diffusion method against a panel of microorganisms viz. Staphylococcus aureus (SA) ATCC29737, Corynebacterium rubrum (CR) ATCC14898, Bacillus subtilis (BS) ATCC6633, Bacillus cereus (BC) ATCC11778, Pseudomonas aeruginosa (PA) ATCC9027, Klebsiella pneumoniae (KP) NCIM2719, Escherichia coli (EC) NCIM2931, Salmonella typhimurium (ST) ATCC23564, Candida albicans (CA) ATCC2091, Candida glabrata (CG) NCIM3438, Cryptococcus neoformans (CN) ATCC34664 and 3 fungal clinical isolates (No. 12, 15, 22). The microorganisms were obtained from National Chemical Laboratory, Pune, India and clinical isolates were collected from local hospital. The fungal clinical isolates belonged to genus Candida. The bacteria and fungi were maintained on nutrient agar and MGYP medium (Hi-Media, India) respectively, at 4 °C and subcultured before use. The microorganisms studied are clinically important ones causing several infections, food borne diseases, spoilages, skin infection and it is essential to overcome them through some active therapeutic agents.
Agar well diffusion assay
The antimicrobial activity was determined by Agar well diffusion assay [Citation18]. Five different concentrations (10, 20, 30, 40 and 50 mg/ml) of AgNPs were made in 100% DMSO; 100 μl of different concentration of nanoparticles was added into the well. DMSO was used as a negative control. Antimicrobial activity was assayed by measuring the diameter of the zone of inhibition formed around the well in millimetres. The experiment was done in triplicate, and the average values were calculated for antimicrobial activity.
Antioxidant activity
Determination of 2, 2-diphenyl-1-picrylhydrazyl (DPPH) free radical scavenging assay
The DPPH free radical scavenging activity of AgNPs was measured by the modified method of McCune and Johns [Citation19]. The reaction mixture (3.0 ml) consisted of 1.0 ml DPPH in methanol (0.3 mM), 1.0 ml methanol and 1.0 ml of different concentrations of AgNPs. The reaction mixture was incubated for 10 min in dark, after which the absorbance was measured at 517 nm using UV-Vis Spectrophotometer (Shimadzu, Japan), against a blank sample. Percentage of inhibition was calculated using the following formula:
where B is the Absorbance of the control (DPPH plus methanol) and A is the absorbance of the sample (DPPH, methanol, plus sample).
Determination of superoxide anion radical scavenging assay
The superoxide anion radical scavenging activity of AgNPs was measured by the method described by Robak and Gryglewski [Citation20]. Superoxide radicals were generated by oxidation of NADH and assayed by the reduction of NBT. The reaction mixture (3.0 ml) consisted of 1.0 ml of different concentrations of AgNPs, 0.5 ml Tris-HCl buffer (16 mM, pH 7), 0.5 ml nitroblue tetrazolium chloride (NBT) (0.3 mM), 0.5 ml NADH (0.936 mM) and 0.5 ml phenazine methosulphate (PMS) (0.12 mM). The superoxide anion radical generating reaction was started by the addition of PMS solution to the reaction mixture. The reaction mixture was incubated at 25 °C for 5 min and then the absorbance was measured at 560 nm using UV-Vis Spectrophotometer (Shimadzu, Japan), against a blank sample. Percentage of inhibition was calculated using the formula described above.
Determination of 2, 2’-Azino-bis-(3-ethyl) benzothiazoline-6-sulfonic acid (ABTS) radical cation scavenging assay
The ABTS radical cation scavenging activity of AgNPs was measured by the method described by Re et al. [Citation21]. ABTS radical cations were produced by reaction of ABTS (7 mM) with potassium persulfate (2.45 mM) and incubated the mixture at room temperature in the dark for 16 h. The ABTS working solution obtained was further diluted with methanol to give an absorbance of 0.85 ± 0.20 at 734 nm. 1.0 ml of different concentrations of AgNPs was added to 3.0 ml of ABTS working solution. The reaction mixture was incubated at room temperature for 4 min and then the absorbance was measured at 734 nm using a UV-Vis Spectrophotometer (Shimadzu, Japan) against a blank sample. Percentage of inhibition was calculated using the formula described above.
In vitro cytotoxic activity
Cancer cell viability of the AgNPs was evaluated by the MTT (3-[4, 5-dimethylthiazol-2-yl]-2, 5-diphenyltetrazolium bromide) assay [Citation22]. The cell lines uses were Human cervical cancer cell line (HeLa), Breast Cancer cell line (MCF-7) and Fibroblast normal cell line. The cell lines were obtained from National Repository of Animal Cell Culture, National Centre for Cell Sciences (NCCS), Pune, India.
Results and discussion
The present study describes an innovative idea of making the best use of waste material. Seeds from fruits that are usually thrown away into the environment can be used for synthesis of AgNPs. This is a new idea that can be therapeutically useful and also reduce environmental pollution. In the present work, AgNPs were synthesised from M. indica fruit waste i.e. seed. The green synthesised AgNPs showed good antioxidant, antimicrobial and anticancer activity.
Characterisation of silver nanoparticles
Visual confirmation of synthesis of silver nanoparticles
The first indication of the formation of AgNPs is the visual colour change in the reaction mixture [Citation23]. In addition of seed extract to silver nitrate solution, the synthesis reaction started with in few minutes and colour change occurred in the reaction mixture. The change is due to the surface plasmon resonance (SPR) phenomenon of silver nanoparticles in the reaction mixture. The colourless AgNO3 solution was converted into a dark brown colour which indicated the reduction of Ag+ to Ag0. The rapid colour change of the colourless reaction mixture to brown to black indicates the rapid synthesis of AgNPs and finally it turned to dark brownish-black colour at 24 h (). Similar visual observation was found in AgNPs synthesised from Prosopis juliflora extract [Citation24].
UV-Visible spectrophotometer analysis
The UV–Vis spectroscopy is the most specific approach for identifying the presence of nanoparticles and the same was employed to monitor the synthesis of AgNPs. The UV-Vis spectra of synthesised nanoparticles was recorded on a spectrophotometer (Shimadzu UV-1601) in 300–800 nm range operated at a resolution of 20 nm. AgNPs emit light between 400 and 800 nm depending on the size, shape and morphology. The AgNPs showed their characteristic absorption maxima between 310 and 800 nm; maximum peak was observed 450 nm () which is the characteristic peak of AgNPs due to surface plasmon resonance (SPR). This is consistent with other reports in the literature. AgNPs synthesised using seed extract of Tectona grandis and Lantana camara leaves showed an absorption maxima at 439 and 440 nm respectively [Citation25,Citation26].
Zeta potential analysis
Zeta potential is a characterisation technique that measures and quantifies the electric charge on the nanoparticle surface. The value of zeta potential indicates the stability of nanoparticles. Particles with zeta potentials higher than +30 mV and lower than −30 mV are indicative of stable nanoparticles. The particle size distribution and zeta potential graphs of green synthesised AgNPs are given in . The zeta potential of AgNPs was 0.67 mV indicating moderate stability. Zeta potential of AgNPs synthesised using Lantana camara leaves was found to be −15.2 mV [Citation25] while AuNPs synthesised from M. indica seed was found to be −1.98 mV [Citation17].
X-ray diffraction analysis (XRD)
The X-ray diffraction studies were performed to estimate the crystalline nature of AgNPs. exhibits the XRD configuration of the M. indica seed extract mediated synthesised AgNPs. The diffractogram showed a distinct diffraction peak at 2Ø value 37.95, 43.08, 64.48 and 77.40 which correspond to the crystal planes of (111), (200), (220) and (311) have face centred cubic structure of AgNPs, respectively (). The diffraction data was synchronous with the standard database of Joint Committee on Powder Diffraction Standards JPCDS file No: 04-0783. The XRD pattern clearly showed that the AgNPs were crystalline in nature. Streblus asper leaf synthesised AgNPs showed comparable similar diffraction peaks which indicated face centre cubic crystal (FCC) structure of green synthesised AgNPs [Citation27].
Fourier transform infrared spectroscopy (FTIR) analysis
FTIR analysis is generally done to identify the functional groups involved in metal nanoparticles formation. FTIR spectra were recorded using Nicolet IS10 (Thermo Scientific, USA), in the range of 400–4000 cm−1 to investigate the biomolecules involved in synthesis and capping of AgNPs. FTIR spectra of synthesised AgNPs showed absorption peaks at 3487.42, 2893.32, 2175.78, 1705.13, 1651.12, 1620.26, 1543.10, 1527.67, 1427.37, 1342.50, 1180.47, 1018.45, 933.58, 864.14, 678.97, 632.67, 516.94, 455.22, 424.35 (). FTIR spectra of seed extract showed absorption peaks at 3533.71, 2731.29, 2175.78, 1705.13, 1651.12, 1527.67, 1435.09, 1342.50, 1180.47, 995.30, 933.58, 864.14, 678.97, 632.67, 509.22, 455.22 and 408.92 cm−1 (). These bands are associated with –OH stretching of alcohols, –CH stretching of alkanes, C = C stretching of alkyne, alkene, aromatic, –NO stretching of nitro, C–N stretching of amino, C–F stretching of alkyl halide and C–H stretching of alkenes, C–Br stretching of alkyl halide, C–N stretching of nitrites, respectively [Citation28]. The peak at 3432 cm−1 and 2926 cm−1 was assigned to O–H stretching of polyphenolic compounds or flavonoids and C–H stretching of proteins respectively [Citation29,Citation30]. M. indica seed powder showed the presence of phenols, flavonoids, tannins and coumarins while its polar solvent methanol extract and aqueous extract revealed the presence of phenols, flavonoids, tannins, cardiac glycosides, coumarins and anthocyanins [Citation17]. The presence of these important secondary metabolites may play a significant role in the formation, reduction, capping and stabilisation of AgNPs. Jaffri and Ahmad [Citation31] reported that phenolic compounds and proteins are actively involved in the reduction of silver to silver nanoparticles by Prunus cerasifera fruit extract. They contained functional groups like alcohol or phenol, carboxylic acid, ketones, amines, aromatics, aromatic amines, aliphatic amines, alkyl halides and alkynes. This is in accordance with our results.
Transmission electron microscopy (TEM) analysis
TEM is employed to determine the morphology, shape and size of NPs. TEM images of synthesised AgNPs at different magnifications is given in . The size of the synthesised AgNPs was in the range 9–61 nm and average size was 26.85 nm. The AgNPs were predominantly round in shape. Sphere-shaped AgNPs with average size of 33.93 nm are reported by Varghese et al. [Citation32] from the seed extract of Trigonella foenum-graecum. Singh et al. [Citation33] reported average particle size of 12–30 nm of AgNPs synthesised using the Rhodiola rosea rhizome extracts which were spherical. The selected area electron diffraction (SAED) pattern of AgNPs prepared by M. indica seed extract is given in . The typical characteristic SAED pattern with bright circular rings characteristic to the indexing planes of (111), (200), (220) and (311) was found which indicated the highly crystalline nature of green synthesised AgNPs which is in good agreement with XRD results (). Similar kind of result was reported with Euphrasia officinalis leaf extract [Citation34].
Energy dispersive X-ray (EDX) analysis
The EDX of the AgNPs was performed to investigate the elemental composition of the biosynthesized AgNPs. The EDX reveals a strong signal at 3 keV () which is generally shown by metallic silver nanocrystals due to surface plasmon resonance [Citation35]. The other metal ions like carbon, potassium and copper also appeared in the EDX spectrum which corresponds to the TEM grid utilised for study [Citation36]. There were small signals for Mg, P and AU which were present in the glassware as impurity.
Antimicrobial activity
The antimicrobial activity of AgNPs was evaluated with 5 different concentrations (10, 20, 30, 40 and 50 mg/ml) against a panel of microorganisms (). The antimicrobial activity was found only at 2 higher concentrations; antibacterial activity was more than antifungal activity (). On the whole moderate antibacterial activity was envisaged however, the antibacterial activity towards Gram-negative bacteria was slightly more than Gram-positive bacteria. Antifungal activity against clinical isolates was maximum; all the concentrations showed antifungal activity except lowest concentration could not inhibit clinical isolate No. 22 (). BC was the most susceptible and CR the most resistant Gram-positive bacteria; KP was the most susceptible while EC was the most resistant Gram-negative bacteria. Shivakumar et al. [Citation13] reported more antibacterial activity than antifungal activity of AgNPs synthesised using pre-hydrolysis liquor of Eucalyptus wood. Devaraj et al. [Citation37] reported similar results; bark-mediated synthesised D. monanta AgNPs showed antibacterial activity more towards Gram-negative bacteria than Gram-positive bacteria. The antibacterial activity was more towards K. aerogenes and E. coli than against B. subtilis and S. aureus. The antibacterial effect of AgNPs synthesised using Berberis vulgaris leaf and root aqueous extract was more against Gram-negative bacteria E. coli than against Gram-positive bacteria S. aureus [Citation38]. Generally, nanoparticles showed antibacterial activity more towards Gram-negative bacteria than Gram-positive bacteria. This difference is because of the difference found in the cell wall structure of both Gram-positive and negative bacteria. The cell wall of Gram-negative bacteria is made up of thin peptidoglycan layer while that of Gram-positive bacteria is made up of thick peptidoglycan layer [Citation39]. The antimicrobial activity also depends on nanoparticle concentration used and the type of bacterial species [Citation40].
Antioxidant activity
The antioxidant potential of AgNPs was evaluated using DPPH free radical scavenging activity, Superoxide anion radical scavenging activity and ABTS radical cation scavenging activity. The AgNPs showed dose dependent antioxidant activity i.e. as concentration of nanoparticles increased, antioxidant activity also increased. DPPH free radical scavenging activity of AgNPs at different concentrations is given in , SO in and ABTS in . In the concentration range of 160–960 µg/ml, DPPH activity of AgNPs showed an inhibition of 16% to 90% with IC50 value of 544 µg/ml; while in the same concentration range, SO activity showed inhibition of 16% to 77% with IC50 value of 460 µg/ml. In the concentration range of 100–600 µg/ml, ABTS activity showed an inhibition of 8% to 89% and its IC50 value was 325 µg/ml. All the three antioxidant activities were found to increase in a dose dependent manner. Dose dependent DPPH antioxidant activity by AgNPs synthesised using Alpinia katsumadai seed extract is reported by He et al. [Citation4] while Patra et al. [Citation41] reported by AgNPs synthesised using Citrullus lanatus rind extract. AgNPs synthesised using C. pulcherrima stem extract also showed dose dependent antioxidant activity [Citation42]. Inbathamizh et al. [Citation43] and Mata et al. [Citation44] reported dose dependent superoxide radical scavenging activity by AgNPs synthesised using Morinda pubescens and Abutilon indicum respectively. Similar results are reported from Caesalpinia pulcherrima flower extract mediated synthesised AgNPs [Citation45]. Concentration dependent increase in ABTS radical scavenging activity is reported by Muniyappan and Nagarajan [Citation46] and Moteriya and Chanda [Citation47]. AgNPs synthesised using Teucrium polium extract showed DPPH and FRAP antioxidant activities [Citation48]. Thus, it can be concluded, that green synthesised AgNPs show antioxidant activity in various antioxidant assays. They can be a good source of natural antioxidants and can be utilised to treat diseases and disorders especially those related with stress and caused by excessive production of ROS.
Cytotoxic effect
The cytotoxic effect of green synthesised AgNPs was evaluated by MTT assay against HeLa, MCF-7 and fibroblast normal cell lines exposed to different concentrations (2, 5, 10, 25, 50, 100, 150, 200, 250 and 400 µg/ml) of AgNPs (). AgNPs showed dose dependent cytotoxicity effect on all the three cell lines; all the three cell lines showed strong negative correlation between % cell viability and concentration of AgNPs; as concentration of AgNPs increased, the % cell viability decreased. AgNPs showed best correlation with breast cancer cell line (r = 0.921**, p < .01) while correlation with HeLa was r = 0.912**, p < .01 and with fibroblast normal cell line, it was r = 0.829**, p < .01(). The synthesised AgNPs showed decreased % cell viability against fibroblast normal cells which indicates that the AgNPs show less cytotoxic effect to normal cells. AgNPs synthesised using Aloin extracted from Aloe barbadensis was evaluated for its cytotoxic potential against MCF-7 cell line which showed an IC50 value of 22 μg/ml [Citation49] while Das et al. [Citation50] reported cytotoxic effect of Ananas comosus mediated synthesised AgNPs against HepG2 cell lines. Mahmoudi et al. [Citation51] reported cytotoxic effect of AgNPs synthesised using Lavandula stoechas extract on RAW264 cell line at 62.5 μg/ml as an effective concentration. In fact, green synthesised AgNPs show promising cytotoxic effect against an array of cell lines like Jurkat, MCF, AGS, Hep3B, MG-63, HT29, U87, A549, HeLa, HeK293, SNO, MKN-28, etc. [Citation52,Citation53]. AgNPs cause damage to macromolecules like lipids, proteins and DNA which finally lead to cell death. AgNPs induce cytotoxic effect due to the physicochemical interaction between AgNPs and cancerous cells, which results in the generation of ROS activate Caspase 3, induced DNA fragmentation and membrane leakage which damage DNA leading to cell death. AgNPs may interact with DNA replication or Ag+ may act as oxidative entities by capturing electrons which leads to decrease in cellular ATP content, increase ROS production which ultimately leads to cell death [Citation54,Citation55]. However, cytotoxic effect of AgNPs depend on concentration, dose, time and size of AgNPs.
Conclusion
In the present work, in accordance with the saying best from waste, we have synthesised AgNPs from M. indica fruit waste i.e. seed. The phytochemicals in the seed acted as reducing and stabilising agent for the formation of AgNPs. The green synthesised nanoparticles were round or spherical in shape with an average size of 26.85 nm. They showed dose dependent antioxidant activity and cytotoxic effect and showed more antibacterial activity towards Gram-negative bacteria than Gram-positive bacteria; best activity was towards fungal clinical isolates. Thus, they showed three biological activities (3-in-1 system). This is a good example of best from waste. Seeds which are generally discarded as waste into the environment can be used to synthesise AgNPs which can be therapeutically used to control many diseases especially infectious, stress related and cancer. They can be a good source of natural antimicrobial, antioxidant and anticancer agents. Further work in understanding the molecular mechanism and in vivo studies are needed. Work in this direction is in progress.
Acknowledgements
The authors thank the Department of Biosciences (UGC-CAS) for providing excellent research facilities.
Disclosure statement
No potential conflict of interest was reported by the author(s).
Data availability statement
The authors confirm that the data supporting the findings of this study are available within the article.
References
- Liao C, Li Y, Tjong SC. Bactericidal and cytotoxic properties of silver nanoparticles. IJMS. 2019;20(2):449.
- Moteriya P, Chanda S. Green synthesis of silver nanoparticles from Caesalpinia pulcherrima leaf extract and evaluation of their antimicrobial, cytotoxic and genotoxic potential (3-in-1 system). J Inorg Organomet Polym. 2020;30(10):3920–3932.
- Donga S, Chanda S. Best from waste: therapeutic potential of plant waste (seeds, peels, flowers). Int J Curr Microbiol App Sci. 2020;9(8):2670–2696.
- He Y, Wei F, Ma Z, et al. Green synthesis of silver nanoparticles using seed extract of Alpinia katsumadai and their antioxidant, cytotoxicity and antibacterial activities. RSC Adv. 2017;7(63):39842–39851.
- Giron-Vazquez NG, Gomez-Gutierrez CM, Soto-Robles CA, et al. Study of the effect of Persea americana seed in the green synthesis of silver nanoparticles and their antimicrobial properties. Results Phys. 2019;13:102142.
- Naidu KSB, Murugan N, Adam JK. Biogenic synthesis of silver nanoparticles from Avicennia marina seed extract and its antibacterial potential. Bionanoscience. 2019;9(2):266–273.
- Varadavenkatesan T, Vinayagam R, Selvaraj R. Structural characterization of silver nanoparticles phyto-mediated by a plant waste, seed hull of Vigna mungo and their biological applications. J Mol Struct. 2017;1147:629–635.
- Singh J, Dhaliwal AS. Novel green synthesis and characterization of the antioxidant activity of silver nanoparticles prepared from Nepeta leucophylla root extract. Anal Lett. 2019;52(2):213–230.
- Chai SH, Wang Y, Qiao Y, et al. Bio fabrication of silver nanoparticles as an effective wound healing agent in the wound care after anorectal surgery. J Photochem Photobiol B. 2018;178:457–462.
- Sabela MI, Makhanya T, Kanchi S, et al. One-pot biosynthesis of silver nanoparticles using Iboza riparia and Ilex mitis for cytotoxicity on human embryonic kidney cells. J Photochem Photobiol B. 2018;178:560–567.
- Fatimah I, Aftrid ZHVI. Characteristics and antibacterial activity of green synthesized silver nanoparticles using red spinach (Amaranthus tricolor L.) leaf extract. Green Chem Lett Rev. 2019;12(1):25–30.
- Khatoon N, Mazumder JA, Sardar M. Biotechnological applications of green synthesized silver nanoparticles. J Nanosci Curr Res. 2017;2(1):107.
- Shivakumar M, Nagashree KL, Yallappa S, et al. Biosynthesis of silver nanoparticles using pre-hydrolysis liquor of Eucalyptus wood and its effective antimicrobial activity. Enzyme Microb Technol. 2017;97:55–62.
- Ediriweera MK, Tennekoon KH, Samarakoon SR. A review on ethnopharmacological applications, pharmacological activities, and bioactive compounds of Mangifera indica (Mango). Evid Based Complement Alternat Med. 2017;2017:6949835.
- Parvez GM. Pharmacological activities of mango (Mangifera indica): a review. Res Rev J Pharmacogn Phytochem. 2016;5(3):1–7.
- Vimalraj S, Ashokkumar T, Saravanan S. Biogenic gold nanoparticles synthesis mediated by Mangifera indica seed aqueous extracts exhibits antibacterial, anticancer and anti-angiogenic properties. Biomed Pharmacother. 2018;105:440–448.
- Donga S, Bhadu GR, Chanda S. Antimicrobial, antioxidant and anticancer activities of gold nanoparticles green synthesized using Mangifera indica seed aqueous extract. Artif Cells Nanomed Biotechnol. 2020;48(1):1315–1325.
- Perez C, Paul M, Bazerque P. An antibiotic assay by the agar well diffusion method. Acta Biol Med Exp. 1990;15:113–115.
- McCune LM, Johns T. Antioxidant activity in medicinal plants associated with the symptoms of diabetes mellitus used by the indigenous peoples of the North American boreal forest. J Ethnopharmacol. 2002;82(2-3):197–205.
- Robak J, Gryglewski RJ. Flavonoids are scavengers of superoxide anions. Biochem Pharmacol. 1988;37(5):837–841.
- Re R, Pellegrini N, Proteggente A, et al. Antioxidant activity applying an improved ABTS radical cation decolorization assay. Free Radic Biol Med. 1999;26(9-10):1231–1237.
- Labieniec M, Gabryelak T. Effects of tannins on Chinese hamster cell line B14. Mutat Res Genet Toxicol Environ Mutagen. 2003;539(1-2):127–135.
- Johnson P, Krishnan V, Loganathan C, et al. Rapid biosynthesis of Bauhinia variegata flower extract-mediated silver nanoparticles: an effective antioxidant scavenger and α-amylase inhibitor. Artif Cells Nanomed Biotechnol. 2018;46(7):1488–1494.
- Arya G, Kumari RM, Gupta N, et al. Green synthesis of silver nanoparticles using Prosopis juliflora bark extract: reaction optimization, antimicrobial and catalytic activities. Artif Cells Nanomed Biotechnol. 2018;46(5):985–993.
- Patil SP, Kumbhar ST. Antioxidant, antibacterial and cytotoxic potential of silver nanoparticles synthesized using terpenes rich extract of Lantana camara L. leaves. Biochem Biophys Rep. 2017;10:76–81.
- Rautela A, Rani J, Das MD. Green synthesis of silver nanoparticles from Tectona grandis seeds extract: characterization and mechanism of antimicrobial action on different microorganisms. J Anal Sci Technol. 2019;10(1):5.
- Das S, Das A, Maji A, et al. A compact study on impact of multiplicative Streblus asper inspired biogenic silver nanoparticles as effective photocatalyst, good antibacterial agent and interplay upon interaction with human serum albumin. J Mol Liq. 2018;259:18–29.
- Rashid MI, Mujawar LH, Mujallid MI, et al. Potent bactericidal activity of silver nanoparticles synthesized from Cassia fistula fruit. Microb Pathog. 2017;107:354–360.
- Daisy P. Saipriya K. Biochemical analysis of Cassia fistula aqueous extract and phytochemically synthesized gold nanoparticles as hypoglycemic treatment for diabetes mellitus. Int J Nanomed. 2012;7:1189–1202.
- Netala VR, Kotakadi VS, Domdi L, et al. Biogenic silver nanoparticles: efficient and effective antifungal agents. Appl Nanosci. 2016;6(4):475–484.
- Jaffri SB, Ahmad KS. Augmented photocatalytic, antibacterial and antifungal activity of prunosynthetic silver nanoparticles. Artif Cells Nanomed Biotechnol. 2018;46(sup1):127–137.
- Varghese R, Almalki MA, Ilavenil S, et al. Silver nanopaticles synthesized using the seed extract of Trigonella foenum-graecum L. and their antimicrobial mechanism and anticancer properties. Saudi J Biol Sci. 2019;26(1):148–154.
- Singh P, Pandit S, Beshay M, et al. Anti-biofilm effects of gold and silver nanoparticles synthesized by the Rhodiola rosea rhizome extracts. Artif Cells Nanomed Biotechnol. 2018;46(sup 3):S886–S899.
- Singh H, Du J, Singh P, et al. Ecofriendly synthesis of silver and gold nanoparticles by Euphrasia officinalis leaf extract and its biomedical applications. Artif Cells Nanomed Biotechnol. 2018;46(6):1163–1170.
- Magudapathy P, Gangopadhyay P, Panigrahi BK, et al. Electrical transport studies of Ag nanoparticles embedded in glass matrix. Physica B. 2001;299(1-2):142–146.
- Singh P, Kim YJ, Wang C, et al. Biogenic silver and gold nanoparticles synthesized using red ginseng root extract, and their applications. Artif Cells Nanomed Biotechnol. 2016;44(3):811–816.
- Devaraj B, Josebin MD, Vasantharaj S, et al. Biosynthesis of silver nanoparticles using stem bark extracts of Diospyros montana and their antioxidant and antibacterial activities. J Nanostruct Chem. 2018;8(1):83–92.
- Behravan M, Panahi AH, Naghizadeh A, et al. Facile green synthesis of silver nanoparticles using Berberis vulgaris leaf and root aqueous extract and its antibacterial activity. Int J Biol Macromol. 2019;124:148–154.
- Kokila T, Ramesh PS, Geetha D. Biosynthesis of silver nanoparticles from Cavendish banana peel extract and its antibacterial and free radical scavenging assay: a novel biological approach. Appl Nanosci. 2015;5(8):911–920.
- Patil MP, Rokade AA, Ngabire D, et al. Green synthesis of silver nanoparticles using water extract from galls of Rhus chinensis and its antibacterial activity. J Clust Sci. 2016;27(5):1737–1750.
- Patra JK, Das G, Baek KH. Phyto-mediated biosynthesis of silver nanoparticles using the rind extract of watermelon (Citrullus lanatus) under photo-catalyzed condition and investigation of its antibacterial, anticandidal and antioxidant efficacy. J Photochem Photobiol B. 2016;161:200–210.
- Moteriya P, Chanda S. Biosynthesis of silver nanoparticles formation from Caesalpinia pulcherrima stem metabolites and their broad spectrum biological activities. J Genet Eng Biotechnol. 2018;16(1):105–113.
- Inbathamizh L, Ponnu TM, Mary EJ. In vitro evaluation of antioxidant and anticancer potential of Morinda pubescens synthesized silver nanoparticles. J Pharm Res. 2013;6(1):32–38.
- Mata R, Nakkala JR, Sadras SR. Biogenic silver nanoparticles from Abutilon indicum: their antioxidant, antibacterial and cytotoxic effects in vitro. Colloids Surf B Biointerfaces. 2015;128:276–286.
- Moteriya P, Chanda S. Synthesis and characterization of silver nanoparticles using Caesalpinia pulcherrima flower extract and assessment of their in vitro antimicrobial, antioxidant, cytotoxic, and genotoxic activities. Artif Cells Nanomed Biotechnol. 2017;45(8):1556–1567.
- Muniyappan N, Nagarajan NS. Green synthesis of silver nanoparticles with Dalbergia spinosa leaves and their applications in biological and catalytic activities. Process Biochem. 2014;49(6):1054–1061.
- Moteriya P, Chanda S. Low cost and ecofriendly phytosynthesis of silver nanoparticles using Cassia roxburghii stem extract and its antimicrobial and antioxidant efficacy. Am J Adv Drug Deliv. 2014;2(4):557–575.
- Bardania H, Mahmoudi R, Bagheri H, et al. Facile preparation of a novel biogenic silver-loaded nanofilm with intrinsic anti-bacterial and oxidant scavenging activities for wound healing. Sci Rep. 2020;10(1):1–4.
- Rose LA, Vidhya S, Janeeta PF, et al. Cytotoxic effect of peptide functionalized silver nanoparticles synthesized from Aloin on breast cancer cell line. Asian J Pharm Clin Res. 2019;12(2):170–175.
- Das G, Patra JK, Debnath T, et al. Investigation of antioxidant, antibacterial, antidiabetic, and cytotoxicity potential of silver nanoparticles synthesized using the outer peel extract of Ananas comosus (L.). PLoS One. 2019;14(8):e0220950.
- Mahmoudi R, Aghaei S, Salehpour Z, et al. Antibacterial and antioxidant properties of phyto‐synthesized silver nanoparticles using Lavandula stoechas extract. Appl Organomet Chem. 2020;34(2):e5394.
- Patil MP, Kim GD. Eco-friendly approach for nanoparticles synthesis and mechanism behind antibacterial activity of silver and anticancer activity of gold nanoparticles. Appl Microbiol Biotechnol. 2017;101(1):79–92.
- Pande J, Chanda S. Screening of anticancer properties of some medicinal plants – review. Int J Curr Microbiol App Sci. 2020;9(3):1348–1362.
- Gurunathan S, Raman J, Abd Malek SN, et al. Green synthesis of silver nanoparticles using Ganoderma neo-japonicum Imazeki: a potential cytotoxic agent against breast cancer cells. Int J Nanomed. 2013;8:4399–4413.
- Moteriya P, Chanda S. Green synthesis of silver nanoparticles: future source of new drugs. Saarbrucken (Germany): LAP LAMBERT Academic Publishing GmbH & Co. KG; 2017.