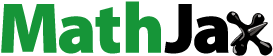
Abstract
This study reports the synthesis of silver nanoparticles (AgNPs) from silver nitrate by leaf extract of a medicinal plant Ziziphus nummularia. The leaf extract acts as a reducing and stabilizing agent for the formation of nanoparticles. The green synthesized AgNPs were characterized by ultraviolet–visible (UV–vis) spectroscopy, Fourier transform infrared (FITR) spectroscopy, Thermogravimetric analysis (TGA), X-ray diffraction (XRD), transmission electron microscopy (TEM) analysis and evaluated their antimicrobial, antioxidant, cytotoxic and genotoxic potential. The UV–Vis spectroscopy showed a characteristic absorption peak at 430 nm due to surface plasma resonance. TEM analysis showed that synthesized AgNPs were spherical and oval with an average size of 25.96 nm. AgNPs showed effective antimicrobial activity (lowest MIC-0.625 µg/mL against Escherichia coli), synergistic antimicrobial activity (lowest ΣFIC 0.09 with chlormaphenicol against Corynebacterium rubrum) and antibiofilm activity. AgNPs showed strong DPPH activity with IC50 − 520 µg/mL and ABTS activity IC50 − 55 µg/mL and reducing capacity assessment. In vitro cytotoxic effect was evaluated by MTT assay against HeLa cells, breast cells and fibroblast cells. Genotoxic effect was evaluated by comet assay. AgNPs displayed dose-dependent cytotoxic and genotoxic effect. Our findings indicated that synthesized AgNPs could be considered as multifunctional and have great potential for use in biomedical applications.
Silver nanoparticles were synthesized using leaf extract of Ziziphus nummularia
Characterization was done by various spectral techniques
Antimicrobial efficacy was demonstrated against an array of bacteria
AgNPs exhibited significant cytotoxic effect against HeLa cell line
AgNPs showed cytotoxicity and genotoxicity in a dose-dependent manner
Highlights
Graphical Abstract
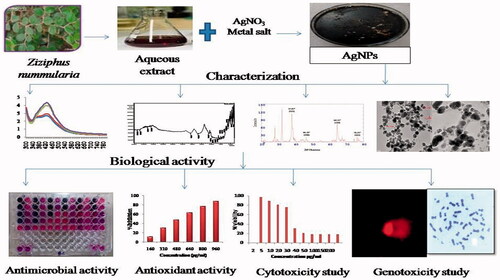
Introduction
Nanoparticles have gained immense importance due to their broad range of applications in different areas such as pharmacy, biology, biotechnology, medical, physic, chemistry, etc. Metal nanoparticles like silver, gold, copper, zinc, platinum show various properties like antifungal and antibacterial, biosensing, drug delivery, cell labelling and imaging [Citation1], etc. There are various physical, chemical and biological methods for synthesis of metal nanoparticles. However, the physical and chemical methods are laborious, time-consuming, not environmental friendly producing toxic chemicals that contaminate the environment and also not convenient for large-scale production. On the other hand, green synthesis i.e. using plant extracts is the most desirous and preferred method. It has many advantages such as cost-effective, simple, eco-friendly and can be easily scaled up for large production. Plants have various phytochemicals like polyphenols alkaloids, flavonoids which are responsible for the reduction of metal ions into metal nanoparticles and act as capping and stabilization agents for synthesized metal nanoparticles. Hence does not require any additional chemicals or compounds. Green synthesized metal nanoparticles have enormous applications in biomedical field such as antibacterial, antifungal, antioxidant and anticancer agents [Citation1,Citation39].
The development of multidrug resistance in pathogens is key hazard to public health because of exploitation of drugs, diagnosis and treatment and overconsumption of antibiotics. Silver nanoparticles have been considered as a possible substitute to antibiotics and act as effective antimicrobial agents against a panel of the pathogenic microorganisms that do not produce microbial resistance and present a good bacteriostatic and bactericidal activity. The biosynthesized AgNPs from A. marina seed extract display broad-spectrum antibacterial activity. The AgNPs showed inhibitory activity against a range of pathogenic bacterial species such as Pseudomonas aeruginosa, Escherichia coli, Bacillus subtilis and Staphylococcus aureus [Citation11,Citation33] synthesized AgNPs using marine red algae Gelidium amansii and evaluated their antibacterial potential against B. pumilus, S. aureus, Aeromonas hydrophila, P. aeruginosa and Vibrio parahaemolyticus.
Cancer is a deadly disease whose mortality level has been rapidly increasing every year. The main modalities used for the treatment of cancer are surgery, chemotherapy and radiotherapy [Citation15]. But considering the various restrictions as well as severe side effects of currently available anticancer drugs and therapy, there is an emerging need to develop new classes of therapeutic agents and drug delivery methodologies for effective release of drug at targeted site. Nanoparticles have been shown to stimulate the apoptotic pathway in vitro which showed antitumor and antiproliferative effects [Citation8]. Nanoparticles have a good free radical scavenging ability by which they act as good antioxidant agents and can enhance the immune defense and lower the risk of various diseases [Citation23].
Ziziphus nummularia (Burm.f.) Wight & Arn. belongs to family Rhamnaceae. It grows abundantly in arid areas of India (). It possesses various pharmacological activities like antioxidant, analgesic and anti-inflammatory, antinociceptive, antipyretic, activity. The leaves are used for the treatment of cough, cold, typhoid and for healing of cuts and cutaneous disease [Citation30]. So it has many medicinal properties hence we selected Ziziphus for synthesis of AgNPs. The aim of the present study was to synthesize multifunctional AgNPs using a natural environmental system. To achieve this goal, this work was designed to synthesize AgNPs using aqueous leaf extract of Z. nummularia and investigation of antimicrobial, antioxidant, cytotoxic and genotoxic potential.
Materials and methods
Plant material and chemicals
Fresh leaves of Z. nummularia were collected from botanical garden of Saurashtra University, Rajkot, Gujarat, India. All the chemicals were obtained from Hi Media Laboratories Pvt. Limited and SRL, Mumbai, India. Mili Q water was used for all the experiments.
Preparation of plant extract
Fresh leaves of Z. nummularia were thoroughly washed with tap water, followed by double distilled water and cut into small pieces. 5 g of cut leaves were mixed with 100 mL Mili Q water and boiled for 10 min. The extract was cooled to room temperature and filtered through Whatman filter paper No.1. The filtrate was used for the synthesis of AgNPs.
Synthesis of silver nanoparticles
Silver nanoparticles were synthesized using, 6 mL of leaves extract was added to 40 mL of 1 mM AgNO3 solution at room temperature (25° C) and incubated in dark for 24 h. The nanoparticle solution was purified by repeated centrifugation at 10,000 rpm for 10 min followed by redispersion of the pellet of nanoparticles in acetone. After air drying of the purified nanoparticles they were stored at 4 °C for further analysis.
Characterization of the silver nanoparticles
Characterization of the synthesized AgNPs was carried out by various spectroscopy techniques. Synthesis of nanoparticles is initially observed by ultraviolet–visible (UV–Vis) spectroscopy. The reduction of the metal ions in solution was monitored by UV–Vis spectra of the solution. UV–Vis spectra was monitored on a spectrophotometer (Analytik Jena, Specord 200 Plus) in 300-700 nm range operated at an interval of 10 nm. Zeta potential is an essential parameter for the determination of stability of nanoparticles. The zeta potential measurement was performed using a Microtra (Zetatra Instruments). Thermal stability and surface weight loss were determined by Thermogravimetric analysis (TGA) using DTG-60H (Shimadzu) instrument. TGA spectra were recorded at a heating rate of 10 °C/min under nitrogen gas flow of 100 mL/min. Possible functional groups involved in the synthesis and stabilization of nanoparticles were studied by FITR spectroscopy. Nanoparticles were mixed with potassium bromide (KBr) and pressed into a pellet. The pellet was placed into the sample holder and spectra were recorded in the range of 400–4000 cm−1 Nicolet IS10 (Thermo Scientific, USA). The various modes of vibrations were identified and assigned to determine the different functional groups present in the Z. nummularia leaf extract. Transmission electron microscopy (TEM) analysis was done to determine the size and shape of nanoparticles. The sample was dispersed in double-distilled water. A drop of thin dispersion was placed on a “staining mat.” Carbon coated copper grid was inserted into the drop with the coated side upwards. After about ten minutes, the grid was removed and air dried. Then screened in JEOL JEM 2100 TEM.
Antimicrobial activity
The antimicrobial activity was determined by measuring MIC by broth dilution method by Resazurin microtiter (REMA) plate method as described by [Citation31] against eleven microorganisms. The synergistic antimicrobial activity of the AgNPs with antibiotics (Chloramphenicol and Ceftazidime for bacteria, Amphotericin B and Nystatin for fungi) was also assessed. Biofilm inhibition activity was determined by 96-well microtiter plate method with some modification [Citation40]. (Details of methods are given in supplementary file).
Antioxidants activity
The antioxidant activity of AgNPs was evaluated by DPPH free radical scavenging activity, ABTS cation radical scavenging activity and Reducing capacity assessment by the methods described in our earlier work [Citation6]. (Details of methods are given in supplementary file).
In vitro cytotoxic activity
Human cervical cancer cell line (HeLa), Breast Cancer cell line (T-47D) and Fibroblast normal cell line were used in this assay, it was obtained from the National Repository of Animal Cell Culture, National Centre for Cell Sciences (NCCS), Pune, India. The cytotoxic effect of AgNPs, was evaluated by the MTT (3-[4,5-dimethylthiazol-2-yl]-2,5-diphenyltetrazolium bromide) assay [Citation20]. 150 µl of cells was seeded in 96-well plates (Becton Dickinson (BD), USA) at the density of 1.1 × 104 viable cells/well and incubated for 24 h to allow cell attachment. Following attachment, the medium was replaced with complete medium (150 µl/well) containing AgNPs, (concentrations ranging from 2–200 µg/mL) for 72 h. Following treatment, the cells were washed with PBS and incubated with 100 µl/well fresh medium containing 0.5 mg/mL MTT. The MTT- containing medium was removed after 3 h incubation in dark conditions. The MTT formazan was dissolved in 100 µl/well DMSO and optical density was determined at 570 nm using an ELISA plate reader (Bio-Tek, USA). Standard anticancer drug Mitomycin C was used as positive control. Cell viability was calculated by the following equation:
where AS is the absorbance of the cells incubated with the nanoparticles and Acontrol is the absorbance of the cells incubated with the culture medium only.
In vitro genotoxicity study
The normal human peripheral blood lymphocyte culture was used for single-cell gel electrophoresis (comet assay) and chromosomal aberration assay. Blood sample was taken from healthy human volunteers.
Comet assay (single-cell gel electrophoresis)
The genotoxicity of AgNPs was determined using Comet assay, based on the measurement of DNA migration under electrophoresis as per slightly modified technique given by [Citation38]. The cells were exposed to AgNPs, (2, 50, 200 µg/mL) for 24 h. The treated cells were then trypsinized to produce a single-cell suspension and 180 µl of 1% normal melting point agarose (NMP) was gelled on fully frosted slide (75 × 25 mm) and 100 µl 0.5% low melting point agarose (LMP) -containing cell suspension (20 µl) was layered on the top of the NMP agarose. After the formation of cell suspension containing layer, additional 100 µl of LMP agarose was added to fill the residual hole and to form an additional layer to increase the distance between the cells and the gel surface. After agarose gel solidification, the slides were placed in a lysis solution [2.5 M NaCl, 100 mM EDTA and 10 mM Tris HCl (pH 10), plus 1% Triton X-100 and 10% DMSO were added just before use] for 24 h at 4 °C, incubated in an alkaline electrophoresis buffer (300 mM NaOH/1 mM EDTA, pH >13) for 40 min and electrophoresed (Midi-Submarine electrophoresis Unit-7050, Tarsons, Kolkata, India) for 35 min. After the comets were formed, the alkaline gel was neutralized by rinsing the slides with a suitable buffer (0.4 M Tris HCl, pH 7.5) three times. The slides were then stained with the fluorescent dye SYBR Green I (SG) and the comet images were captured under fluorescence microscope. The proportion of DNA damaged was scrutinized by scoring 100 comets for each group (Tri-Tek Comet-Score™ V1.5 software, Germany).
Chromosomal aberration assay
Lymphocyte cultures were prepared by as method described by [Citation14] with slight modifications. Heparinized whole blood (0.5 mL) was added to mixture containing 5 mL of culture medium RPMI-1640, 0.1 mL phytohemagglutinin (Lectin) and 0.05 mL heparin and culture vials were put in an incubator for 72 h, at 37 °C. Lymphocyte cultures were exposed to AgNPs, (2, 50, 200 µg/mL), positive control, and negative control and incubated for 96 h of incubation. Then 0.1 mL demecolcine solution was added at 94 h of the incubation period to arrest cells at metaphase. The cells were collected by centrifugation, resuspended in a pre-warmed hypotonic solution (KCl, 0.075 M) for 20–25 min and fixed in chilled methanol/acetic acid (3:1, v/v) solution (Carnoy’s fixative). Suspensions of cells were prepared after several changes of Carnoy’s fixative washes. Using the cell suspension, slides were prepared and air dried on a hot plate at 50–60 °C. The slides were then stained with 2% Giemsa’s stain for 10 min. From each slide, 100 well spread metaphase chromosomes were scored for chromosome and chromatid type of aberrations.
Results and discussion
Characterization of silver nanoparticles
UV–Vis spectroscopy
The AgNPs showed their characteristic absorption maxima between 400 and 460 nm. The maximum and narrow absorption peak was observed at 430 nm (). AgNPs synthesized using O. sanctum leaf extract showed the maximum absorption peak at 430 nm which is due to surface plasma resonances [Citation19].
Zeta potential analysis
Zeta potential value indicates surface charge and stability of nanoparticles. The zeta potential value ±30 is considered as stable nanoparticles. The zeta potential value of synthesized AgNPs was −4.31 mv (). The negative charge on the surface of nanoparticles can cause strong repulsion among the particles and thereby increase stability of nanoparticles.
Table 1. Zeta potential of AgNPs.
Thermal gravimetric analysis (TGA)
TGA analysis of AgNPs revealed initial weight loss of 1.3% at 100 °C which was ascribed to removal of water molecules absorbed on the surface of nanoparticles. The subsequent weight loss of AgNPs was 21.52% at 100 °C to 800 °C was due to thermal decomposition of plant bioorganic compounds absorbed on the surface of nanoparticles () [Citation18] observed steady weight loss of 42.3% of AgNPs synthesized using olive leaf extract in the temperature range of 160–600 °C due to desorption of bioorganic compounds from surface of AgNPs .
Fourier transform infrared spectroscopy (FTIR)
FTIR spectra of synthesized AgNPs showed intense peak at 2358.94, 1323.17, 1029.99, 613.16, and 532.35 cm−1. The absorption band at 2358.94 cm−1 is characteristic of the C–H strong stretching of methylene group. The absorption band at 1323.17 cm−1 and 1029.99 cm−1 corresponds to C-N stretching of aliphatic amine and –C–O– or –C–O–C– stretching of carboxyl group respectively. The absorption band at 613.16 cm−1 and 532.35 cm−1 is characteristic of C–X stretching of halide alkanes () [Citation19] reported that AgNPs synthesized using Tulsi leaf extract showed absorption band at 2349 cm−1 corresponds to C–H which may be due to stretching of methylene group and 1635 cm−1 may be due to stretching of amide group. FTIR spectrum identified different functional groups suggested that AgNPs synthesized using Z. nummularia leaf extract are capped by polyphenols, proteins and flavonoides having functional groups of methylene, carboxyl, alcohol and aliphatic amine which act as reducing and stabilizing agents for nanoparticles. Similar results are also reported by [Citation25], they reported a significant role of C. microphylla fruit extract in the reduction of Ag ions and formation of silver nanoparticles
X-Ray diffraction analysis (XRD)
X-ray diffraction (XRD) analysis of synthesized AgNPs showed distinct diffraction peak at 2Ø value 27.12, 37.87, 46.33, 64.55 and 76.25 which correspond to the crystal planes of (100), (111), (200), (220) and (311) have face centred cubic structure of AgNPs respectively (). The observed data are in good agreement with the standard database of Joint Committee on Powder Diffraction Standards JPCDS file No: 04-0783. The face centred cubic crystal structure of AgNPs synthesized by Abutilon indicum leaf and Seriphidium quettense plant extract was reported by Ashokkumar et al. [Citation3] and Nasar et al. [Citation28], respectively.
Transmission electron microscopy analysis (TEM)
The size of AgNPs was in the range of 10.63 nm − 47.37 nm and average size was 25.96 nm. The shape of AgNPs were highly diversified which included spherical and some were oval () [Citation7] reported average particle size of 25–60 nm of gold nanoaprticles synthesized using the Convolvulus fruticosus extract. The SAED image showed bright circular spots corresponding to various orientations appearing inside the rings indicated that AgNPs were crystalline in nature (). Similar kind of result was reported with Berberis aristata [Citation35].
Antimicrobial activity
The antimicrobial activity of synthesized AgNPs was evaluated using the minimum inhibitory concentration is given . It ranged from 0.625 to >10 mg/mL. AgNPs strongly inhibited Gram-negative bacteria, E. coli was the most susceptible bacterial pathogen (MIC- 0.625 mg/mL). The MIC value of S. typhimurium and K. pneumoniae was 1.25 mg/mL. AgNPs inhibited the Gram-positive bacteria by higher MIC value. AgNPs effectively inhibited fungal pathogens; Candida glabrata was the most susceptible fungal pathogen (MIC—1.25 mg/mL). AgNPs strongly inhibited the growth of Gram-negative bacteria as compared to Gram-positive bacteria and fungi. Rajabi et al., [Citation34] reported that green synthesized nanoparticles using Suaeda aegyptiaca plant extract exhibited good antimicrobial activity against various pathogenic microorganisms.
Various mechanisms have been proposed to explain the antimicrobial activity of nanoparticles. One possible mechanism was interference in ATP production and DNA replication by uptake of free metal ions Lok et al., [Citation22] elucidated that AgNPs exhibited destabilization of outer membrane and rupture of the plasma membrane, thereby causing depletion of intracellular ATP. Li et al., [Citation21] reported that AgNPs inhibit DNA replication and condensed DNA as resulted in cell death. Nanoparticles directly interact with the microbial cells and cause damage to the cell membranes. Balakumaran et al., [Citation4] observed the accumulation of AgNPs over the cell wall of microorganisms and release of intracellular protein and nucleic acid as compared to control group which reflects a loss in membrane integrity and cell death.
Synergistic antimicrobial activity
Synergistic effect was determined by calculating FIC index of nanoparticles and antibiotics, and results of the synergistic effect in the form of ƩFIC are presented in . The combination of AgNPs with chlormaphenicol exhibited synergistic effect on growth of four Gram-positive bacteria and four Gram-negative bacteria with ΣFIC indices ranged from 0.09 to 0.31. Maximum synergistic activity of AgNPs with chlormaphenicol found against E. coli with ΣFIC indices 0.31. The combination of AgNPs with ceftazidime exhibited synergistic effect on growth of Corynebacterium rubrum and E. coli with ΣFIC indices 0.3 and 0.27 respectively, whereas combination showed indifferent and antagonistic effect against remaining bacterial strains.
Table 2. ΣFIC index of Ag-NPs with antibiotics against bacteria and fungi.
The combination of AgNPs with amphotericine B exhibited synergistic effect on growth of fungi C. glabrata and C. neoformans with ΣFIC indices 0.16 and 0.09 respectively and the combination showed partial synergistic effect on growth of C. albicans with ΣFIC indices 0.75. The combination of AgNPs with nystatin exhibited synergistic effect on growth of fungi C. albicans, C. glabrata and C. neoformans with ΣFIC indices 0.16, 0.15 and 0.16 respectively. Ghosh et al., [Citation12] investigated synergistic antibacterial activity of AgNPs synthesized using Dioscorea bulbifera tuber extract with 22 different antibiotics against 14 bacterial strains and results suggested that AgNPs combination with antibiotic streptomycin showed maximum 11.8 fold increase in zone diameter against E. coli. Synergistic antimicrobial activity of nanoparticles may be due to the bonding reaction between nanoparticles and antibiotic. Nanoparticles-antibiotics conjugates are formed in which nanoparticles core is surrounded by antibiotic molecules thereby enable the penetration of nanoparticles into the microbes, which results in damage of biomolecules like DNA, protein, lipid and death of microbial cells [Citation10,Citation27].
Antibiofilm activity
The synthesized AgNPs showed dose-dependent antibiofilm activity. Maximum % biofilm inhibition by AgNPs at concentration 10 mg/mL was against B. subtilis (82% inhibition) () amongst Gram-positive bacteria while amongst Gram-negative bacteria, it was against E. coil (88% inhibition) () and amongst fungi was against C. glabrata (80% inhibition) (). The synthesized AgNPs exhibited significant antibiofilm activity against all tested microorganisms. Goswami et al., [Citation13] reported antibiofilm activity of AgNPs and found biofilm inhibition of 89% for S. aureus and 75% for E. coli at 15 mg/mL. Kalishwaralal et al. [Citation16] reported 95% inhibition of biofilm formation of P. aeruginosa and S. epidermidis treated with silver nanoparticles. AgNPs synthesized using aqueous leaf extracts of E. officinalis were able to inhibit the biofilm formation in concentration-dependent manner. It was found that at a concentration of 10 mg/mL AgNPs completely inhibited the biofilm formation of S. aureus and P. aeruginosa [Citation37].
Antioxidant activity
DPPH activity was found to increase in a dose-dependent manner. The IC50 value of AgNPs was 520 µg/mL (). Kanipandian et al.[Citation17] reported DPPH free radical scavenging activity of AgNPs synthesized from Cleistanthus collinus, 69% inhibition at a concentration of 1000 µg/mL however the activity was quite less as compared to the present results; the synthesized AgNPs showed 88% inhibition at concentration of 960 µg/mL. ABTS radical cation scavenging activity was also dose dependent, IC50 value of synthesized AgNPs was 55 µg/mL ().Nagajyothi et al., [Citation26] also reported dose-dependent ABTS radical cation scavenging activity for AgNPs synthesized using Inonotus obliquus extract. The reducing capacity of AgNPs showed a steady increase in the absorbance of the reaction mixture with increase in concentration of AgNPs (). Similar results are reported by [Citation5] for AgNPs synthesized using Syzygium cumini seed extract.
In vitro cytotoxic effect
The synthesized AgNPs showed dose-dependent cytotoxic effect, when the concentration of nanoparticles increased, the percent cell viability decreased. The standard anticancer drug Mitomycin C was used as positive control which showed 23%, 38% and 38% cell viability of HeLa cells, Breast cells and Normal cells at 50 µg/mL concentrations respectively while negative control without nanoparticles showed 100% cell viability (). A clear cytotoxic effect was observed at higher concentrations of AgNPs. AgNPs exhibited higher cytotoxic effect against HeLa cells as compared to Breast cells and Normal cells. The synthesized AgNPs showed decreased % cell viability against fibroblast normal cells as compared to HeLa cancer cells and breast cancer cells. The results suggested that synthesized AgNPs have less cytotoxic effect to normal cells as compared to cancer cells. The anticancer activity of AgNPs is reported against RAW 246.7 cells (murine macrophage, non-cancerous), A549 (human lung cancer) and HeLa (human cervical cancer) cell lines [Citation37]. Cytotoxic effect of nanoparticles may be due to generation of reactive oxygen species (ROS) which damage the intracellular components of cells via oxidative stress [Citation23,Citation29] Mata et al., reported that AgNPs synthesized using A. indicum leaf extract induced apoptotic cell death at a very low concentration in human colon cancer (COLO 205) cell; DNA fragmentation and cell cycle arrest occurred because of increase in ROS generation. Samadian et al. [Citation26] and Moloudi et al. [Citation24] developed gold nanoparticles base nanomedicine which exhibited an excellent cancer chemotherapy against human breast cancer cells.
In vitro genotoxicity study
Comet assay
The cells treated with lower concentration of AgNPs (2 and 50 μg/mL) showed intact and round nuclei without any DNA fragmentation () while cells treated with higher concentrations (200 µg/mL) showed DNA fragmentation (). The cells treated with standard anticancer drug Mitomycin C used as positive control showed fragmentation of DNA and increasing comet length at 50 µg/mL concentration () while negative control without nanoparticles showed intact and round nuclei, without any DNA fragmentation (). The synthesized AgNPs showed dose-dependent genotoxicity. The % tail DNA increased with increasing concentrations of AgNPs, and maximum % tail DNA was found at 200 µg/mL concentrations, but it was less than that of positive control. The standard anticancer drug Mitomycin C was used as positive control which showed 75% tail DNA at 50 µg/mL concentration while negative control without nanoparticles showed 13% tail DNA. When concentration of AgNPs was 2 µg/mL, 50 µg/mL and 200 µg/mL, % Tail DNA was 13%, 22% and 31% respectively (). AgNPs showed 13% tail DNA at 2 µg/mL concentration which almost similar to negative control.
Chromosomal aberration assay
Lower concentration of AgNPs (2 and 50 μg/mL) treated cells did not show appearance of aberrations () while higher concentration of AgNPs (200 µg/mL) showed chromatid break in chromosome (). The cells treated with mitomycin C drug showed aberrations like interchange and break in metaphase () while negative control without nanoparticles showed clearer metaphase (). Dose-dependant genotoxicity effects of AgNPs are reported by other researchers [Citation9,Citation32]. The results suggested that green synthesized AgNPs did not induce genotoxic effect in normal human cells at low concentration.
Conclusion
In the present study, we report a simple, nontoxic, cost-effective, eco-friendly and green method for synthesis of AgNPs using Z. nummularia leaf extract. The UV–Vis spectroscopy showed maximum absorption peak at 430 nm. The average size of AgNPs was 25.96 nm as revealed by TEM analysis and the shape of nanoparticles was spherical and oval. AgNPs showed promising antimicrobial activity and synergistic antimicrobial activity against pathogenic microorganisms. AgNPs exhibited significant antioxidant activity. AgNPs showed potential cytotoxic effect against HeLa and breast cancer cells as compared to normal fibroblast cells. The activity of AgNPs may be enhanced because of medicinal properties of Ziziphus plant extract. The green synthesized AgNPs showed four significant biological activities (4-in-1 system), i.e. antimicrobial activity against Gram-positive, Gram-negative and fungal pathogens, antioxidant activity against different free radicals like DPPH, ABTS and reducing power and cytotoxic activity against Human cervical cancer cell line (HeLa) and Human breast cancer cell line (T-47D) and biocompatible with Human fibroblast normal cell line, and AgNPs did not induce genotoxic effect in normal human cells at low concentration, proving their biomedical importance; thus showing various biological activities (4-in-1 system). Based on the results, Z. nummularia synthesized AgNPs could be employed as a source for the exploration of novel therapeutic agents in biomedical field to control various human diseases.
Acknowledgements
The authors thank the Department of Biosciences (UGC-CAS) for providing excellent research facilitie
Disclosure statement
No potential conflict of interest was reported by the author(s).
Data availability statement
The authors confirm that the data supporting the findings of this study are available within the article.
References
- Salem SS, Fouda A. Green synthesis of metallic nanoparticles and their prosective biotechnological applications: an overview. Biol Trace Elem Res. 2021;199(1):344–370.
- Alvand ZM, Rajabi HR, Mirzaei A, et al. Ultrasonic and microwave assisted extraction as rapid and efficient techniques for plant mediated synthesis of quantum dots: green synthesis, characterization of zinc telluride and comparison study of some biological activities. New J Chem. 2019;43(38):15126–15138.
- Ashokkumar S, Ravi S, Kathiravan V, et al. Synthesis of silver nanoparticles using A. indicum leaf extract and their antibacterial activity. Spectrochim Acta A Mol Biomol Spectrosc. 2015;134:34–39.
- Balakumaran MD, Ramachandran R, Balashanmugam P, et al. Mycosynthesis of silver and gold nanoparticles: optimization, characterization and antimicrobial activity against human pathogens. Microbiol Res. 2016;182:8–20.
- Banerjee J, Narendhirakannan RT. Biosynthesis of silver nanoparticles from Syzygium cumini (L.) seed extract and evaluation of their in vitro antioxidant activities. Digest J Nanomater Biostruct. 2011;6(3):961–968.
- Chanda S, Rakholiya K, Dholakia K, et al. Antimicrobial antioxidant and synergistic properties of two nutracutical plants Terminalia catappa L. and Colocasia esculenta L. Turk J Biol. 2013;37:81–91.
- Ebrahimzadeh MA, Naghizadeh A, Mohammadi-Aghdam S, et al. Enhanced catalytic and antibacterial efficiency of biosynthesized Convolvulus fruticosus extract capped gold nanoparticles (CFE@AuNPs). J Photochem Photobiol B. 2020;209:111949.
- Eid AM, Fouda A, Niedbała G, et al. Endophytic Streptomyces laurentii mediated green synthesis of Ag-NPs with antibacterial and anticancer properties for developing functional textile fabric properties. Antibiotics. 2020;9(10):641.
- Ernest V, Doss GP, Muthiah A, et al. Genotoxicity assessment of low concentration AgNPs to human peripheral blood lymphocytes. Int J Pharm Pharm Sci. 2013;5(2):377–381.
- Fayaz AM, Balaji K, Girilal M, et al. Biogenic synthesis of silver nanoparticles and their synergistic effect with antibiotics: a study against Gram-positive and Gram-negative bacteria. Nanomedicine. 2010;6(1):103–109.
- Fouda A, Saad EL, Salem SS, Shaheen TI. In-Vitro cytotoxicity, antibacterial, and UV protection properties of the biosynthesized Zinc oxide nanoparticles for medical textile applications. Microb Pathog. 2018;125:252–261.
- Ghosh S, Patil S, Ahire M, et al. Synthesis of silver nanoparticles using Dioscorea bulbifera tuber extract and evaluation of its synergistic potential in combination with antimicrobial agents. Int J Nanomedicine. 2012;7:483–496.
- Goswami SR, Sahareen T, Singh M, et al. Role of biogenic silver nanoparticles in disruption of cell–cell adhesion in Staphylococcus aureus and Escherichia coli biofilm. J Ind Eng Chem. 2015;26:73–80.
- Hungerford DA. Leukocytes cultured from small inocula of whole blood and the preparation of metaphase chromosomes by treatment with hypotonic KCl. Stain Technol. 1965;40(6):333–338.
- Jemal D, Thomas A, Murray T, et al. Cancer statistics, 2002. CA Cancer J Clin. 2002;52(1):23–47.
- Kalishwaralal K, Barath Mani Kanth B, Pandian SR, et al. Silver nanoparticles impede the biofilm formation by Pseudomonas aeruginosa and Staphylococcus epidermidis. Colloids Surf B Biointerfaces. 2010;79(2):340–344.
- . Kanipandian N, Kannan S, Ramesh R, et al. Characterization, antioxidant and cytotoxicity evaluation of green synthesized silver nanoparticles using Cleistanthus collinus extract as surface modifier. Mater Res Bull. 2014;49:494–502.
- Khalil MMH, Ismail EH, El-Baghdady KZ, et al. Green synthesis of silver nanoparticles using olive leaf extract and its antibacterial activity. Arabian J Chem. 2014;7(6):1131–1139.
- Khatoon N, Mishra A, Alam H, et al. Biosynthesis, characterization, and antifungal activity of the silver nanoparticles against pathogenic Candida species. BioNanoSci. 2015;5(2):65–74.
- Labieniec M, Gabryelak T. Effects of tannins on Chinese hamster cell line B14. Mutat Res Genet Toxicol Environ Mutagen. 2003;539(1-2):127–135.
- Li WR, Xie XB, Shi QS, et al. Antibacterial effect of silver nanoparticles on Staphylococcus aureus. Biometals. 2011;24(1):135–141.
- Lok CN, Ho CM, Chen R, et al. Proteomic analysis of the mode of antibacterial action of silver nanoparticles. J Proteome Res. 2006;5(4):916–924.
- Mata R, Nakkala JR, Sadras SR. Biogenic silver nanoparticles from Abutilon indicum: Their antioxidant, antibacterial and cytotoxic effects in vitro. Colloids Surf B Biointerfaces. 2015;128:276–286.
- Moloudi K, Samadian H, Jaymand M, et al. Iron oxide/Gold nanoparticles-decorated reduced graphene oxide nanohybrid as the thermo-radiotherapy agent. IET Nanobiotechnol. 2020;14(5):428–432.
- Mortazavi-Derazkola S, Ebrahimzadeh MA, Amiri O, et al. Facile green synthesis and characterization of Crataegus microphylla extract-capped silver nanoparticles (CME@ Ag-NPs) and its potential antibacterial and anticancer activities against AGS and MCF-7 human cancer cells. J Alloys Compd. 2020;820:153186.
- Nagajyothi PC, Sreekanth TVM, Lee J, et al. Mycosynthesis: antibacterial, antioxidant and antiproliferative activities of silver nanoparticles synthesized from Inonotus obliquus (Chaga mushroom) extract. J Photochem Photobiol B. 2014;130:299–304.
- Naqvi SZH, Kiran U, Ali MI, et al. Combined efficacy of biologically synthesized silver nanoparticles and different antibiotics against multidrug-resistant bacteria. Int J Nanomed. 2013;8:3187–3195.
- Nasar MQ, Zohra T, Khalil AT, et al. Seripheidium quettense mediated green synthesis of biogenic silver nanoparticles and their theranostic applications. Green Chem Lett Rev. 2019;12(3):310–322.
- Nayak D, Minz AP, Ashe S, et al. Synergistic combination of antioxidants, silver nanoparticles and chitosan in a nanoparticle based formulation: characterization and cytotoxic effect on MCF-7 breast cancer cell lines. J Colloid Interface Sci. 2016;470:142–152.
- Padalia H, Chanda S. Characterization, antifungal and cytotoxic evaluation of green synthesized zinc oxide nanoparticles using Ziziphus nummularia leaf extract. Artif Cells Nanomed Biotechnol. 2017;45(8):1751–1761.
- Palomino JC, Martin A, Camacho M, et al. Resazurin microtiter assay plate: simple and inexpensive method for detection of drug resistance in Mycobacterium tuberculosis. Antimicrob Agents Chemother. 2002;46(8):2720–2722.
- Patlolla AK, Berry A, May LB, et al. Genotoxicity of silver nanoparticles in Vicia faba: a pilot study on the environmental monitoring of nanoparticles. IJERPH. 2012;9(5):1649–1662.
- Pugazhendhi A, Desika Prabakar D, Jacob JM, et al. Synthesis and characterization of silver nanoparticles using Gelidium amansii and its antimicrobial property against various pathogenic bacteria. Microb Pathog. 2018;114:41–45.
- Rajabi HR, Naghiha R, Kheirizadeh M, et al. Microwave assisted extraction as an efficient approach for biosynthesis of zinc oxide nanoparticles: synthesis, characterization, and biological properties. Mater Sci Eng C Mater Biol Appl. 2017;78:1109–1118.
- Saddal SK, Telang T, Bhange VP, et al. Green synthesis of silver nanoparticles using stem extract of Berberis aristata and to study its characterization and antimicrobial activity. J Pharm Res. 2018;12(6):840–844.
- Samadian H, Mohammad-Rezaei R, Jahanban-Esfahlan R, et al. A de novo theranostic nanomedicine composed of PEGylated graphene oxide and gold nanoparticles for cancer therapy. J Mater Res. 2020;35(4):430–441.
- Singh H, Du J, Singh P, et al. Ecofriendly synthesis of silver and gold nanoparticles by Euphrasia officinalis leaf extract and its biomedical applications. Artif Cells Nanomed Biotechnol. 2018;46(6):1163–1170.
- Singh NP, McCoy MT, Tice RR, et al. A simple technique for quantitation of low levels of DNA damage in individual cells. Exp Cell Res. 1988;175(1):184–191.
- Sriranjani R, Srinithya B, Vellingiri V, et al. Silver nanoparticle synthesis using Clerodendrum phlomidis leaf extract and preliminary investigation of its antioxidant and anticancer activities. J Mol Liq. 2016;220:926–930.
- Stepanovic S, Vukovic D, Hola V, et al. Quantification of biofilm in microtiter plates: overview of testing conditions and practical recommendations for assessment of biofilm production by Staphylococci. APMIS. 2007;115(8):891–899.