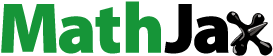
Abstract
Higher tobacco and alcohol use have led to a consistent increase in head and neck cancer incidence rates. Currently employed chemotherapeutic and surgical treatment are associated with significant drawbacks. Herein, we evaluated the anti-tumour effect of gold nanoparticles as a vehicle for the delivery of a triple chemotherapy drug formulation and elucidated the potential underlying mechanism. The hydrodynamic size of docetaxel, cisplatin, and 5-fluorouracil physically co-adsorbed on Au nanoparticles was 56 ± 0.8 nm, showing a negative zeta potential. Fourier transform infra-red spectroscopy data confirmed that the triple chemotherapy drug successfully interacted with the gold nano-carrier. Au nanoparticles exhibited high loading efficacy of docetaxel (61%), cisplatin (75%), and 5-fluorouracil (90%), with a controlled drug release profile at 24 h. The triple chemotherapy drug formulation was tested in human oral cavity cancer cell line (KB). Cytotoxicity achieved through a synergistic effect between the treatments led to apoptosis, with a lower half-maximal inhibitory concentration indicating higher cytotoxicity than that of plain docetaxel-cisplatin-fluorouracil. Taken together, we demonstrated that the docetaxel-cisplatin-fluorouracil-gold complex exhibited excellent cytotoxicity in KB cells, superior to that docetaxel-cisplatin-fluorouracil.
The docetaxel-cisplatin-fluorouracil-Au complex showed a controlled drug-release profile at 24 h.
The docetaxel-cisplatin-fluorouracil-Au complex exhibited enhanced internalisation efficiency in cells.
Au nanoparticles were biocompatible, with no change in apoptosis among cell line.
Spherical Au nanoparticles allowed a high volume of incorporated docetaxel, cisplatin, and 5-fluorouracil to steadily attach onto cells.
HIGHLIGHTS
Introduction
Together with radical surgery, chemotherapy, radiotherapy, and combined radio-chemotherapy represent powerful tools in the fight against various types of cancer, including head and neck squamous cell carcinoma (HNSCC) [Citation1]. However, conventional treatment approaches are limited by non-specific distribution and considerable toxicity. In light of these drawbacks, the combination of controlled drug release and targeted drug delivery holds great promise. Thus, the development of nanotechnology-based drug delivery systems is expected to improve treatment outcomes.
A double-drug chemotherapy regimen, with cisplatin as an antineoplastic agent and 5-fluorouracil as an antimetabolite, has been used in patients with head and neck squamous cell carcinoma. However, the frequency of cisplatin and 5-fluorouracil resistance was 33.0% and 40.2%, respectively [Citation2]. To date, triple drug chemotherapy regimens, adding docetaxel to cisplatin plus 5-fluorouracil, have been shown to achieve better response rates than the double-drug regimen. Docetaxel-based treatments suppress proliferation and induce apoptosis. Further, docetaxel could also increase the survival rates of patients with advanced, recurrent, or metastatic (R/M) head and neck squamous cell carcinoma [Citation3]. A major drawback of docetaxel treatment is its poor water solubility [Citation4]. Further, previous studies were carried out in vitro and in patients receiving high doses of the triple chemotherapy drug formulation, which cannot be achieved at the tumour site [Citation5–11].
Recently, the use of nanoparticles to transport chemotherapeutics to tumour sites has gained considerable attention, as a nanoparticulate drug delivery system would not only enhance bioavailability while lowering the required dose, but would also enable the more targeted drug delivery to tumour cells [Citation12,Citation13]. Among various nanoparticles, gold nanoparticles have outstanding physiochemical properties, allowing for easy surface modification with biomolecules and low toxicity. Based on the outstanding physiochemical properties of gold nanoparticles, gold-based chemotherapeutic regimens have been extensively used for bioimaging and targeted drug delivery. Nanoparticle-based chemotherapy drug regimen has been studied in breast, cervical, colon, liver, lung, pancreatic, prostate, and skin cancers [Citation14–21], but this approach has not yet been explored for head and neck cancer therapy. In the present study, we prepared docetaxel-cisplatin-fluorouracil mixed with gold nanoparticles and treated human oral cavity cancer cell line to evaluate its potential as a therapy for patients with head and neck cancers.
Materials and methods
Reagents and cells
Docetaxel, cisplatin, and 5-fluorouracil were obtained from Sigma Aldrich, USA. Dulbecco’s Modified Eagle’s Medium (DMEM) and phosphate-buffered saline (PBS) were purchased from Sigma Aldrich, USA. 7-amino-actinomycin D (7-AAD) reagent was purchased from BioLegend, USA. Citrate-capped gold nanospheres were bought from Nanoseedz, Hong Kong. Methanol and dimethyl sulfoxide (DMSO) were purchased from Merck, USA. Human oral cavity cancer cell line KB was obtained from the Scientific Instrument Centre, KMITL, Thailand.
Docetaxel-cisplatin-fluorouracil-Au preparation
Docetaxel in powder form was completely dissolved in 1% DMSO. A clear solution was then added to 0.9% saline solution and sonicated for 20 min to achieve a nano-suspension [Citation22,Citation23]. Cisplatin and 5-fluorouracil in powder form were dissolved in 0.9% saline solution. Triple-drug sample bound to the nanoparticle surface by sonication technique as follows. Each drug sample was simultaneously loaded in a dropwise to a fixed volume of the gold solution; then, they were stirred for 1 h using mechanical stirrer. The resultant mixture was sonicated overnight for 16 h using ultrasonic bath (Elma: P120H, Germany) in order to produce a stable docetaxel-cisplatin-fluorouracil shell around each gold core. The test drug was formulated with docetaxel: cisplatin: 5-fluorouracil: gold at a ratio of 1: 1: 10: 2 (w/w) (). After synthesis, the free drug was detached via centrifugation at 4500 rpm for 15 min. All solutions were sterilised by passing through a 450 nm pore filter. The adsorbed drugs on gold nanoparticles were re-dispersed for characterisation purposes (pH = 6.0).
Table 1. Detailed preparation of triple chemotherapy drug with Au nanoparticles.
Particle size and zeta potential analysis
One milliliter of the sample was aliquoted and examined for hydrodynamic size and zeta potential using dynamic light scattering (Malvern Zetasizer ZSP, Worcestershire, UK).
FTIR analysis
FTIR was used to analyse the interaction of functional groups in the test drug and the gold nanocarrier. 0.1 mL of sample was transferred to the aluminium plate at a wavelength range of 4000–400 cm−1 (Thermo Scientific Nicolet 6700, USA).
Spectrophotometric analysis
One milliliter of the sample (reaction product) and reagent blank (0.9% saline solution) were used to determine adsorption using a UV-Vis spectrophotometer (UV-1800, Shimadzu, Japan). The absorbance was measured at 520 nm. Absorbance was converted to concentration based on calibration curves, and the drug loading efficacy was quantified via the following formula [Citation24]:
(1)
(1)
Cytotoxicity assay
KB cells were grown using DMEM with 10% FBS and kept in a humidified incubator at 37 °C in 5% CO2 atmosphere. The cell line was seeded in a 96-well plate at a density of 10,000 cells/well and incubated overnight. Subsequently, each well was substituted with fresh medium containing different concentrations of docetaxel-cisplatin-fluorouracil, docetaxel-cisplatin-fluorouracil-Au, Au, and 0.9% saline solution (negative control), followed by incubation at 37 °C in a 5% CO2 atmosphere for 24 h. Thus, the culture medium was substituted with MTT at 5.00 mg/mL and further incubated at 37 °C in 5% CO2 atmosphere for 4 h. After MTT removal, DMSO was added to disintegrate formazan crystals, followed by the detection of absorbance at a wavelength of 570 nm using a microplate reader (Tecan Infinite M200, Switzerland). The % growth inhibition was calculated, and the test drug dose required to inhibit 50% cell growth (IC50) was determined from the dose-response data. The IC50 values were obtained via nonlinear regression analysis based on a sigmoid dose-response curve and calculated using GraphPad Prism software, version 5.0.
7-AAD staining of dead cells for flow cytometry
KB cells were seeded overnight in a 24-well plate at a density of 100,000 cells/well. The adherent cell line was treated with the IC50 for docetaxel-cisplatin-fluorouracil, docetaxel-cisplatin-fluorouracil-Au, and Au formulations, then further incubated at 37 °C in 5% CO2 atmosphere for 1, 2, and 24 h. After incubation, the cell line was trypsinized in 0.25% trypsin-EDTA, washed twice with 1 mL of cell staining buffer (1% BSA; Fraction-V in PBS) via centrifugation at 1000 rpm for 5 min, and re-suspended with 0.5 of 7-AAD reagent in 100
of cell staining buffer for 10 min at room temperature in the dark. Stained cells were washed twice with 1 mL of cell staining buffer and resuspended in 500
of the same solution. These cells were used to examine cell death via flow cytometry (Beckman Coulter: CytoFLEX S, USA). The percentage of cell death, determined based on 7-AAD-positive cells, was analysed using FlowJo software, version 10.0. The surface morphology of KB cells under white light and blue lasers was pictured to obtain phase-contrast and fluorescence micrographs using a fluorescence microscope (Nikon: Eclipse Ti2, Japan).
Statistical analysis
All experiments were performed in triplicate. The experimental data are shown as the mean ± standard deviation (SD). Statistical analyses were performed using one-way ANOVA followed by Tukey test with the significance level set at p < 0.05.
Results
Morphological characterisation
Transmission electron micrographs of the sphere-shaped citrate-capped Au nanoparticles revealed that the mean hydrodynamic diameter of blank Au nanoparticles and the docetaxel-cisplatin-fluorouracil-Au complex were 31 ± 0.4 nm and 56 ± 0.8 nm, respectively, indicative of potentially enhanced internalisation in cells [Citation25]. Au nanoparticles with or without the test drug maintained their size for 24 h on the periphery of the citrate ion, (C3H5(COO−)3.
In general, the zeta potential for steady particle suspensions should be between −30 mV and +30 mV. A value out of this range would indicate that particles have a high tendency to accumulate, resulting in particle sedimentation. In this study, the zeta potential was −27.30 ± 9.76 mV for blank Au nanoparticles and −25.50 ± 1.34 mV for the docetaxel-cisplatin-fluorouracil-Au complex. This result confirmed that Au nanoparticles exhibit good colloidal stability and do not aggregate after associating with the test drug. The 24 h particle size test also supported zeta potential data, indicating that the docetaxel-cisplatin-fluorouracil-Au complex was a stable colloidal dispersion.
FTIR data
FTIR spectroscopy was employed to investigate possible chemical structure and chemical interactions between the test drug and citrate-capped Au nanoparticles (). Docetaxel presented characteristic peaks of the benzene ring at 711 cm−1 and 1494 cm−1, a peak at 1713 cm−1 for the carbonyl group (C=O), and a peak at 3409.02 cm−1 for the primary amine group (N–H) [Citation26]. Cisplatin presented the NH/OH stretching bands at 1296−1 and 3300 cm−1 [27,28]. 5-Fluorouracil showed peaks for the primary amine groups (N–H) at 3409.02 cm−1 and 3566.89 cm−1 as well as one for the carbonyl group (C═O) at 1647.80 cm−1 [Citation29]. Citrate-capped Au nanoparticles presented a characteristic peak at 1396 cm−1, conforming to the symmetric and asymmetric stretching of (COO−) [Citation28,Citation30]. Some characteristic peaks of plain docetaxel, cisplatin, and 5-fluorouracil still existed in the docetaxel-cisplatin-fluorouracil combination. These data suggested a chemical interaction or band overlap (no new peak shown). The docetaxel-cisplatin-fluorouracil-Au complex exhibited shifts in all spectra, indicative of the substitution and the success of interactions between the test drug and the Au colloidal dispersion. The characteristic peaks of docetaxel reduced in the complex, which proved that docetaxel entered the cavity of the clathrate-like water.
Optical properties
The introduction of Au nanoparticles while maintaining docetaxel, cisplatin, and 5-fluorouracil at a weight ratio of 1:1:10 exhibited a positive effect on their co-adsorption. shows the absorbance of citrate-capped Au nanoparticles with different concentrations of test drugs. The Au nanoparticles exhibited an absorption maximum at the wavelength of 520 nm as per surface resonance. Au nanoparticles associated with docetaxel-cisplatin-5-fluorouracil molecules showed slight shifts in maximum wavelength when compared with free Au nanoparticles, suggesting a possible physical interaction at the Au-drug interface. The slight change in wavelength and no change in colour of the sample also confirmed that Au nanoparticles attached to the drug molecules were dispersed in solution and not aggregated.
Figure 2. Representative UV–Vis spectra of free Au nanoparticles and Au nanoparticles associated with different concentrations of the test drugs at 0 h (A) and 24 h (B). Docetaxel, cisplatin, 5-fluorouracil (TPF), and Au nanoparticles prepared using different chemotherapy drug and nanoparticle compositions are shown in .
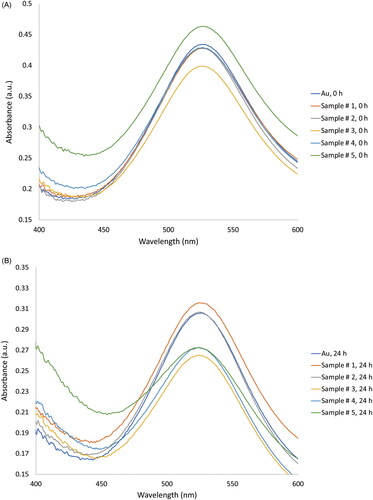
Absorbance of the docetaxel-cisplatin-fluorouracil-Au complex (Sample # 1–5) was reduced when the number of the binding drug molecules for every Au nanoparticle increased. This might be due to the lower specific area of citrate-capped Au nanoparticles that might retard the co-adsorption of the individual test drug. A mix of docetaxel, cisplatin, 5-fluorouracil (TPF), and Au nanoparticles prepared using different drug-to-nanoparticle compositions (obtained from ), were therefore used to calculate the drug loading efficacy. The TPF formulation (T:P:F) was 1:1:10 w/w for the highest efficacy reported by several groups [Citation7–11]. The docetaxel-cisplatin-fluorouracil-Au formulation (1:1:10:2 w/w) was optimised so as to acquire the maximum drug loading efficacy, with approximately 61% of docetaxel, 75% of cisplatin, and 90% of 5-fluorouracil.
In vitro cytotoxicity study
shows cytotoxicity data for KB cells exposed to different concentrations of the test drugs. Docetaxel, cisplatin, 5-fluorouracil (TPF), and Au nanoparticles prepared at different drug-to-nanoparticle compositions were used to calculate the IC50. Au nanoparticles showed the highest IC50 value of 0.83 mg/mL, indicating no cytotoxicity against KB cells. Thus, Au nanoparticles were biocompatible. Interestingly, the docetaxel-cisplatin-fluorouracil-Au complex exhibited greater cytotoxicity against KB cells than docetaxel-cisplatin-fluorouracil, with a lower IC50 value (0.06 mg/mL, 0.06 mg/mL, and 0.69 mg/mL compared to 0.08 mg/mL for docetaxel, 0.08 mg/mL for cisplatin, and 0.80 mg/mL for 5-fluorouracil). This finding suggested that the use of docetaxel-cisplatin-fluorouracil with Au nanoparticles might allow for the continuous release of docetaxel, cisplatin, and 5-fluorouracil without compromising their therapeutic effect. This enabled the intracellular accumulation of nanoparticles, as per our cellular uptake results.
Table 2. Cytotoxicity data obtained for KB cells exposed to different concentrations of the test drugs.
Live/dead cell study
The test drug was expected to induce the apoptosis of treated cells. The intact plasma membranes of viable cells exclude the 7-aminoactinomycin D (7-AAD) fluorescent dye, whereas the ruptured membranes of late apoptosis/dead cells enable the binding of 7-AAD to double-stranded DNA between base pairs within G-C-rich regions, allowing for the quantification of cell death via flow cytometry [Citation31]. Cell death rates in negative and positive control cells were 0.48 ± 0.03% and 93.20 ± 5.60% (mean ± SD, n = 3), respectively. The rate of cell death almost disappeared between 1 and 2 h of incubation time intervals of the cells treated with the IC50. After 24 h of incubation time with the test drug, late apoptosis cells accumulated (). 7-AAD was undetectable in cells treated with Au nanoparticles alone, represented by the FITC channel (KB3). Au nanoparticles were deemed biocompatible as they did not induce an increase in apoptosis. KB cells treated with the docetaxel-cisplatin-fluorouracil-Au complex (KB2) exhibited a significantly higher rate of cell death, at 85.70 ± 1.21% (mean ± SD, n = 3), as compared to those treated with docetaxel-cisplatin-fluorouracil (KB1), at 80.63 ± 0.29% (mean ± SD, n = 3), which could be attributed to the increased availability of docetaxel-cisplatin-fluorouracil in Au nanoparticles. This result was consistent with cytotoxicity analysis, suggesting that the docetaxel-cisplatin-fluorouracil-Au complex tended to enhance the rates of docetaxel-cisplatin-fluorouracil-induced apoptosis.
Figure 3. (A) Representative dot plots showing the distribution of gated cell populations and late apoptotic/dead KB cells after 24 h of incubation. (B) Percentage of dead cells after 1, 2, and 24 h of incubation. Groups: untreated cells (negative control), 95% ethanol-treated cells (positive control), docetaxel-cisplatin-fluorouracil-treated cells (KB1), docetaxel-cisplatin-fluorouracil-Au-treated cells (KB2), and Au-treated cells (KB3) (p < 0.05).
After 24 h of incubation with the test drug, control cell line displayed a smooth cellular surface with typical cytoplasmic projections (). Many viable cells were observed following treatment with Au nanoparticles (KB3). Meanwhile, severe destruction of cellular structures and a few viable cells were noted after exposure to the docetaxel-cisplatin-fluorouracil-Au complex (KB2). Further, the shape of KB cells was altered, with less cytoplasmic projections. These morphological (bright-green line in the figure) changes might be a result of disturbance in the cytoskeleton dynamics, mainly induced by the Au nanoparticles (bright-green dot in the figure) loaded with docetaxel, cisplatin, and 5-fluorouracil. Considerably more viable cells were noted after exposure to docetaxel-cisplatin-fluorouracil (KB1).
Figure 4. Representative surface morphology of KB cells. Phase-contrast image of control KB cells without treatment (A1), KB1 = cells treated with docetaxel-cisplatin-fluorouracil (A2), KB2 = cells treated with the docetaxel-cisplatin-fluorouracil-Au complex (A3), and KB3 = cells treated with plain Au nanoparticles (A4). Fluorescence image of control KB cells without treatment (B1), KB1 = cells treated with docetaxel-cisplatin-fluorouracil (B2), KB2 = cells treated with the docetaxel-cisplatin-fluorouracil-Au complex (B3), and KB3 = cells treated with plain Au nanoparticles (B4). Objective: 40×.
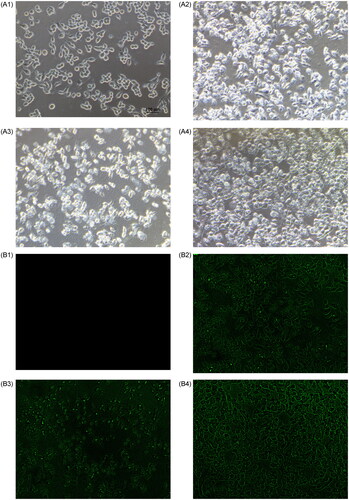
Discussion
The novel formulations that allow for the more targeted delivery of chemotherapy drugs and the use of smaller dosages are urgently needed in order to improve treatment outcomes in cancer patients, including those with head and neck cancer. This study described the development and evaluation of a triple chemotherapy drug regimen composed of docetaxel, cisplatin, and 5-fluorouracil, which were incorporated onto gold nanoparticles as vehicles for their enhanced specific delivery to the disease site and improved drug release. The citrate-capped Au nanoparticles loaded with the triple chemotherapy drug formulation exhibited a remarkable increase in the mean hydrodynamic diameter, with the compounds forming a layer on the surface of Au nanoparticles. In addition, Au nanoparticles presented a high zeta potential, conferring good colloidal stability. The surface charge was altered upon addition of chemotherapeutic compounds. These results suggested the presence of test drugs on the Au nanoparticle surface together, indicative of a system with good stability.
Surface functionality analysis determined the stability and reactivity of citrate-capped Au nanoparticles with the triple chemotherapy drug formulation, whereas drug localisation on citrate-capped Au nanoparticles influenced drug release. shows the release rates of docetaxel, cisplatin, and 5-fluorouracil with Au nanoparticles (pH = 6.0). Spherical Au nanoparticles allowed for the incorporation of a large volume of docetaxel, cisplatin, and fluorouracil onto the cells.
Table 3. Release rates of docetaxel, cisplatin, and 5-fluorouracil with Au nanoparticles (pH = 6.0).
DMSO was used to solubilise docetaxel, which is insoluble in water. The clear solution was thus mixed with water to form a nanosuspension which allowed a clathrate-like water cage to form around DMSO molecules [Citation32]. Water molecules would preferentially donate hydrogen bonds to citrate anions on the Au nanoparticle surface. Docetaxel in the nano-suspension form associated with cisplatin was transported across the cell membranes, and the synergistic effect caused mitochondrial destruction partly due to reactive oxygen species–induced alternations in the mitochondrial membrane potential. Among the outstanding characteristics of docetaxel is its cytotoxic effect against cisplatin-resistant cancers [Citation33]. The Korsmeyer-Peppas model was used to explain the localisation of docetaxel as a hydrophobic molecule in nanosuspension with citrate-capped Au nanoparticles, which resulted in a slow release rate proportional to time [Citation34–36].
Citrate anions on the Au nanoparticle surface were loosely bound and thus easy to conjugate with 5-fluorouracil. Zero-order kinetics was used to explain the localisation of polar/hydrophilic 5-fluorouracil molecules in the periphery of the citrate ion (C3H5(COO-)3 capped on Au nanoparticles, which resulted in an intermediate release rate proportional to time. 5-fluorouracil act as an antimetabolite [Citation34,Citation37].
The chloro-monoaqua form of cisplatin, [PtCl(NH3)2(H2O)]+, has cations that can bind with COO- from citrate-capped Au nanoparticles, exhibiting a slow release rate proportional to time [Citation27,Citation38]. This could ultimately give rise to DNA and protein adducts through the easy substitution of water by a nitrogen-donor ligand.
Combination chemotherapy comprised of docetaxel and cisplatin (TP) resulted in a high response rate for locally advanced cancers of the head and neck in comparison to the standard induction regimen of cisplatin and 5-fluorouracil (PF) [Citation2]. In this study, the use of chemotherapeutic agents (TPF) with Au nanoparticles enhanced the adsorption and permeability properties of TPF, thus further increasing the therapeutic efficacy, with an apoptotic rate of approximately 86%. The co-adsorption of TPF in the Au nanoparticles further reduced the threshold concentrations which were required for a synergistic effect when compared to the TPF formulation despite only 24 h of induction chemotherapy. This approach could reduce adverse effects as a result of the lower amount of chemotherapeutics applied, in addition to a better-targeted delivery of the formulation.
There are some limitations in our cellular toxicity experiments. KB cell line in the present study was used as a model of head and neck squamous cell carcinoma (HNSCC) in order to screen the efficacious antitumor treatments and to understand their synergistic effects on tumour cells before introduction into clinical use. KB cell line was actually derived from the cervical cancer cells (HeLa cell line) [Citation39]. Therefore, a better confirmation of the present study would be that other HNSCC cell lines (such as CAL-27, Detroit-562, FaDu, and SCC-25) and followed by HNSCC patients, would be treated with a docetaxel-cisplatin-fluorouracil-gold complex for different periods of time and thus tested with MTT assay and flow cytometry.
Conclusions
Herein, we reported the physicochemical characteristics of a nanocarrier-based triple chemotherapy drug formulation. The test drugs physically mixed with gold nanoparticles were subjected to various analyses, including that of zeta potential, UV-Vis spectroscopy, Fourier transform infra-red spectroscopy, and fluorescence imaging. Their surface morphologies and microstructures were evaluated via dynamic light scattering. One day after treatment, cytotoxicity and apoptosis were evaluated in human oral cavity cancer cell line. The docetaxel-cisplatin-fluorouracil-Au complex was stable for 24 h, with consistent specific distribution and high drug loading efficiency (61% for docetaxel, 75% for cisplatin, and 90% for 5-fluorouracil). The in vitro release test demonstrated continuous and sustained release of the docetaxel-cisplatin-fluorouracil-Au complex. Further, the complex exhibited high cytotoxicity against human oral cavity cancer cell line, at a lower dose when compared with plain docetaxel-cisplatin-fluorouracil. The docetaxel-cisplatin-fluorouracil-Au complex entered cells via diffusion. In conclusion, our findings support the potential of the docetaxel-cisplatin-fluorouracil-Au complex as a promising chemotherapy formulation against with head and neck cancers, allowing for the administration of lower chemotherapeutic doses and a more specific targeting of the disease site.
Authors’ contributions
Wannisa Khamaikawin participated in the research design, data collection, data analysis, and wrote the manuscript. Kitsakorn Locharoenrat devised the research design, data collection, data analysis, research summary, and wrote the manuscript. Both authors read and approved the final manuscript.
Acknowledgments
The authors thank the Scientific Instrument Center, King Mongkut’s Institute of Technology Ladkrabang, for providing cancer cell line.
Disclosure statement
No potential conflict of interest was reported by the author(s).
Data availability statement
The authors confirm that the data supporting the findings of this study are available within the article.
Additional information
Funding
References
- Paul BF, Kenneth DT. Advances in cancer research. Cambridge (MA): Academic Press; 2021.
- Fatemeh A, Nafiseh V, Parya E, et al. Drug resistance against 5-fluorouracil and cisplatin in the treatment of head and neck squamous cell carcinoma: a systematic review. J Dent Res Clin Dent Prospects. 2021;15(3):219–225.
- Andreas EA, Regina G, Xu Q, et al. Efficacy and toxicity of docetaxel combination chemotherapy for advanced squamous cell cancer of the head and neck. Mol Clin Oncol. 2017;7(1):151–157.
- da Rocha MCO, da Silva PB, Radicchi MA, et al. Docetaxel-loaded solid lipid nanoparticles prevent tumor growth and lung metastasis of 4T1 murine mammary carcinoma cells. J Nanobiotechnol. 2020;18(1):43.
- Sindhu VG, Safeena K, Ramanan SP, et al. Establishment and characterization of triple drug resistant head and neck squamous cell carcinoma cell lines. Molecular Med Reports. 2015;12(2):3025–3032.
- Tae MY, Sun-Ae K, Dong HL, et al. Livin enhances chemoresistance in head and neck squamous cell carcinoma. Oncol Reports. 2017;37(6):3667–3673.
- Haddad RI, Posner M, Hitt R, et al. Induction chemotherapy in locally advanced squamous cell carcinoma of the head and neck: role, controversy, and future directions. Ann Oncol. 2018;29(5):1130–1140.
- Devale T, Vini S, Rajesh K, et al. Randomized controlled study comparing efficacy and toxicity of weekly vs. 3-weekly induction chemotherapy in locally advanced head and neck squamous cell carcinoma. Front Oncol. 2020;10:1284.
- Maureen B, Michel L, Anne-Françoise D, et al. Predictive factors of toxicity of TPF induction chemotherapy for locally advanced head and neck cancers. BMC Cancer. 2021;21:360.
- Jan BV. Where and when to use induction chemotherapy in head and neck squamous cell cancer. In: Vermorken JB, Budach V, Leemans CR, Machiels JP, Nicolai P, O’Sullivan B, editors. Critical issues in head and neck oncology. Cham: Springer; 2021. p. 155–179.
- Katsunori K, Kiyoto S, Hisanori A. Preliminary study of chemoradiotherapy using modified docetaxel, cis-diaminodichloroplatinum, and 5-fluorouracil for sinonasal squamous cell carcinoma. OTO Open. 2021;5(3):2473974X211045298.
- Nahid R, Anjana P. Engineered nanoparticles as drug delivery systems. Boca Raton (FL): CRC Press; 2022.
- Yashwant VP, Jayvadan KP. Pharmacokinetics and pharmacodynamics of nanoparticulate drug delivery systems. New York: Springer Nature; 2022.
- Wang Z, Dong J, Zhao Q, et al. Gold nanoparticle-mediated delivery of paclitaxel and nucleic acids for cancer therapy (review). Mol Med Rep. 2020;22:4475–4484.
- Shrestha B, Wang L, Zhang H, et al. Gold nanoparticles mediated drug-gene combinational therapy for breast cancer treatment. Int J Nanomed. 2020;15:8109–8119.
- Vodyashkin AA, Rizk MGH, Kezimana P, et al. Application of gold nanoparticle-based materials in cancer therapy and diagnostics. Chem Eng. 2021;5(4):69.
- Tomşa AM, Răchişan AL, Aldea AA, et al. Perspectives of gold nanoparticles and their applications in pancreatic cancer (review). Exp Ther Med. 2021;21:258.
- Faid AH, Shouman SA, Badr YA, et al. Enhanced cytotoxic effect of doxorubicin conjugated gold nanoparticles on breast cancer model. BMC Chem. 2022;16:90.
- Shahidi M, Abazari O, Dayati P, et al. Aptamer-functionalized chitosan-coated gold nanoparticle complex as a suitable targeted drug carrier for improved breast cancer treatment. Nanotechnol Rev. 2022;11(1):2875–2890.
- Yang Z, Wang D, Zhang C, et al. The applications of gold nanoparticles in the diagnosis and treatment of gastrointestinal cancer. Front Oncol. 2022;11:819329.
- Alle M, Sharma G, Lee SH, et al. Next-generation engineered nanogold for multimodal cancer therapy and imaging: a clinical perspectives. J Nanobiotechnol. 2022;20:222.
- Archana SP, Riya H, Anand PG, et al. Exploring the solvent-anti-solvent method of nanosuspension for enhanced oral bioavailability of lovastatin. Turk J Pharm Sci. 2021;18(5):541–549.
- Fariba P, Elaheh R, Sima AA, et al. Solubilization methods for paclitaxel and docetaxel: an overview. Pharm Sci. 2022;28(2):208–223.
- Locharoenrat K. Efficacy of nanodiamond–doxorubicin complexes on human breast adenocarcinoma cell lines. Artif Cells Nomed Biotechnol. 2019;47(1):4053–4058.
- Sanità G, Carrese B, Lamberti A. Nanoparticle surface functionalization: how to improve biocompatibility and cellular internalization. Front Mol Biosci. 2020;7:587012.
- Lai YH, Chih-Sheng C, Chin-Hao H, et al. Development and characterization of a fucoidan-based drug delivery system by using hydrophilic anticancer polysaccharides to simultaneously deliver hydrophobic anticancer drugs. Biomolecules. 2020;10(7):970.
- Teruo H, David RB, Tsuyoshi M, et al. Blue moon ensemble simulation of aquation free energy profiles applied to Mono and bifunctional platinum anticancer drugs. J Comput Chem. 2020;41(22):1973–1984.
- González-López MA, Gutiérrez-Cárdenas EM, Sánchez-Cruz C, et al. Reducing the effective dose of cisplatin using gold nanoparticles as carriers. Cancer Nanotechnol. 2020;11:4.
- Farhat J, Aisha L, Fiza A, et al. Synthesis of 5-fluorouracil cocrystals with novel organic acids as coformers and anticancer evaluation against HCT-116 colorectal cell lines. Cryst Growth Des. 2020;20(4):2406–2414.
- Indiani CDV, Bethina TS, Mauricio LF, et al. Reducing the effective dose of cisplatin using gold nanoparticles as carriers. J Nanoparticle Res. 2020;22:133.
- Zembruski NC, Stache V, Haefeli WE, et al. 7-Aminoactinomycin D for apoptosis staining in flow cytometry. Anal Biochem. 2012;429(1):79–81.
- Panuszko A, Bruździak P, Śmiechowski M, et al. DMSO hydration redefined: unraveling the hydrophobic hydration of solutes with a mixed hydrophilic–hydrophobic characteristic. J Molecular Liquids. 2019;294:111661.
- Imamura Y, Tanaka K, Kiyota N, et al. Docetaxel plus cisplatin in recurrent and/or metastatic non-squamous-cell head and neck cancer: a multicenter phase II trial. Med Oncol. 2021;38(11):128.
- Aarti M, Shridhar N, Swaminathan S, et al. Novel resveratrol and 5-fluorouracil coencapsulated in PEGylated nanoliposomes improve chemotherapeutic efficacy of combination against head and neck squamous cell carcinoma. Biomed Res Inter. 2014;2014:424239.
- Yuchen B, Qinfang , Yongyong L, Songwen Z, et al. Engineering docetaxel-loaded micelles for non-small cell lung cancer: a comparative study of microfluidic and bulk nanoparticle preparation. RSC Adv. 2018;8:31950–31966.
- Roghayyeh VG, Seyed MR, Reza FM, et al. Optimization of docetaxel loading conditions in liposomes: proposing potential products for metastatic breast carcinoma chemotherapy. Sci Reports. 2020;10:5569.
- Huan Y, Qi Y, Yuan L, et al. Co-encapsulation of paclitaxel and 5-fluorouracil in folic acid-modified, lipid-encapsulated hollow mesoporous silica nanoparticles for synergistic breast cancer treatment. RSC Adv. 2022;12(50):32534–32551.
- Ahmed K, Alexandra BT, Idan S, et al. Biodegradable controlled-release device for localized chemotherapeutic treatment of bladder cancer. ACS Biometer Sci Eng. 2021;7(6):2548–2557.
- National Center for Biotechnology Information. National Library of Medicine. KB cells. [cited 2023 Feb 21]. https://www.ncbi.nlm.nih.gov/mesh/?term=KB+Cells.