Abstract
Helicobacter pylori (H. pylori) is recognized as a pathogen associated with several gastrointestinal diseases. The current treatments exhibit numerous drawbacks, including antibiotic resistance. H. pylori can adhere to and colonize the gastric mucosa through H. pylori adhesin A (HpaA), and antibodies against HpaA may be an effective therapeutic approach. The variable domain of immunoglobulin new antigen receptor (VNAR) is a novel type of single-domain antibody with a small size, good stability, and easy manufacturability. This study isolated VNARs against HpaA from an immune shark VNAR phage display library. The VNARs can bind both recombinant and native HpaA proteins. The VNARs, 2A2 and 3D6, showed high binding affinities to HpaA with different epitopes. Furthermore, homodimeric bivalent VNARs, biNb-2A2 and biNb-3D6, were constructed to enhance the binding affinity. The biNb-2A2 and biNb-3D6 had excellent stability at gastrointestinal pH conditions. Finally, a sandwich ELISA assay was developed to quantify the HpaA protein using BiNb-2A2 as the capture antibody and BiNb-3D6 as the detection antibody. This study provides a potential foundation for novel alternative approaches to treatment or diagnostics applications of H. pylori infection.
Introduction
Helicobacter pylori (H. pylori) is a Gram-negative, spiral-shaped bacterium and a significant public health concern, as it infects nearly half of the global population and can cause various gastrointestinal problems and other diseases [Citation1,Citation2]. In the 1990s, H. pylori was identified by the World Health Organization (WHO) as a class-I carcinogen, as it is the strongest identified risk factor for the development of serious gastric complications such as chronic gastritis, gastric and duodenal ulcers, and even gastric cancer [Citation3–5]. Moreover, H. pylori is associated with an increased risk of developing certain other diseases, such as cardiovascular disease, Parkinson’s disease, and certain lymphomas [Citation6]. Current therapies for H. pylori infection involve triple and quadruple therapies combining antibiotics, proton-pump inhibitors, and bismuth [Citation7]. Nevertheless, increasing antibiotic resistance is becoming a growing public health problem [Citation8]. Vaccines are common strategies to prevent infectious diseases. However, there is no H. pylori vaccine is extensively applied [Citation9,Citation10]. Therefore, the development of alternative therapeutic approaches is crucial for effective eradication of H. pylori infection.
Adhesion to and colonization of the stomach are critical stages of development in H. pylori infection [Citation11,Citation12]. Initially, H. pylori must adhere to the epithelial cells of the stomach by adhesins to cause the further release of virulence factors that damage the gastric mucosa [Citation12]. H. pylori adhesin A (HpaA), one of the adhesins expressed by H. pylori, is a lipoprotein on the surface and flagellar sheath of H. pylori. Its nucleotide and amino acid sequences are conserved across different H. pylori strains [Citation13,Citation14]. HpaA is reported as a neuraminyllactose-binding haemagglutinin (NLBH) that can attach to several glycosylated receptors on the gastric epithelial cells, playing an important role in bacterial adhesion and colonization of the gastric mucosa. Furthermore, previous studies have demonstrated the significance of HpaA in the colonization of H. pylori in mice [Citation15,Citation16]. Additionally, HpaA-based vaccines have been studied for the prevention and therapies of H. pylori infection [Citation17,Citation18]. Therefore, HpaA represents a promising candidate for the detection and treatment of H. pylori infection.
Conventional antibodies and recombinant antibody fragments have been studied to target and neutralize H. pylori infection [Citation19–22]. However, conventional antibodies face challenges in oral administration, including instability in the strong acid environment of the stomach and susceptibility to degradation by pepsin. Recently, a novel class of single-domain antibodies, known as the variable domain of immunoglobulin new antigen receptor (VNAR), can provide a potential solution [Citation23]. VNARs are derived from heavy chain-only antibodies discovered in cartilaginous fishes. VNARs possess several advantageous features, including small size (11–12 kDa), ease of engineering, high solubility, low aggregation propensity, binding to inaccessible unique epitopes, resistance to high temperature, extreme pH, and proteases [Citation24–26]. Considering these benefits, VNARs offer a promising alternative approach to detecting and neutralizing H. pylori infection.
In this study, we intended to develop VNARs against the HpaA of H. pylori. We expressed and purified the HpaA recombinant protein and immunized the white-spotted bamboo sharks. To obtain VNARs targeting HpaA, an immune phage display library was constructed using RNA extracted from peripheral blood lymphocytes (PBLs) and the spleens of the sharks. Two VNARs recognizing different epitopes of HpaA were isolated and characterized. To improve the binding affinity, we constructed bivalent VNARs and then developed a sandwich ELISA assay for the detection of HpaA. These findings may provide a foundation for the development of diagnostic reagents and oral antibody drugs for H. pylori infection.
Materials and methods
Immunogen production
The HpaA-coding DNA sequence was cloned into the Nde I and Xho I restriction sites, and then, the plasmid was transformed into E. coli BL21(DE3) cells. The isopropyl β-D-1-thiogalactopyranoside (IPTG) of 0.1 mM was used to induce the recombinant antigen HpaA overnight at 18 °C when OD600 was between 0.4–0.6. The bacterial cells were subsequently harvested via centrifugation at 6000 g for 30 min. The bacterial precipitate was resuspended, and the proteins were extracted by sonication. The HpaA protein featuring a 6 × His tag was purified utilizing Ni-NTA affinity chromatography (Cytiva) and was subsequently eluted with 10 ml of PBS containing 300 mM imidazole. Dialysis of the eluted HpaA proteins was performed using 2 L of dialysis buffer (PBS, pH 7.4), followed by desalting utilizing an Ultra-filtration column (Millipore, UFC901008). The purity of the protein was checked by SDS-PAGE.
Immunization of striped bamboo shark and VNAR library construction
After collection of normal serum from white-spotted bamboo sharks (Chiloscyllium plagiosum), the sharks were injected with recombinant antigen HpaA (400 μg) mixed with an equivalent of the volume of Freund’s complete adjuvant (Sigma-Aldrich) into the lateral fin of sharks for the first injection. The interval between the five immunological boosts (200 μg) was two weeks. Blood and spleen samples were taken one week after the last booster injection. To confirm the immune response against antigens, ELISA was performed using serial dilutions of pre-immune and immune serum. Spleens and PBLs that were isolated from bleeding plasma had the RNA extracted from them, and cDNA was synthesized by using oligo-dT primers and a PrimeScript IV kit (Takara). The PCRs were executed in a reaction volume of 50 μL, utilizing 1 μL of cDNA that had been purified from a 1% agarose gel as the template. The reactions were carried out on a thermal cycler (Applied Biosystems) to amplify VNAR fragments of approximately 500 bp in size [Citation27]. The PCR fragments and phagemid pComb3XSS were digested by Sfi I restriction enzyme (NEB) at 50 °C overnight and ligated overnight at 16 °C with T4 DNA ligase. Following ligation, electroporation was employed to transfer the ligated product into E. coli TG1 cells and colonies were subsequently obtained by plating serial dilutions on 2 × TY agar plates supplemented with ampicillin resistance at 37 °C.
Selection of anti-HpaA VNARs
The amplification of the phage display library was carried out in 200 ml of 2 × TY liquid culture medium supplemented with 100 μg/mL ampicillin. The bacterial cultures, which were in the mid-log phase, were infected with M13KO7 helper phage (NEB) with a titre of 1011 pfu. The mixture was then incubated overnight at 30 °C in a 2 × TY culture medium containing both 100 μg/mL ampicillin and 50 μg/mL kanamycin. The bacterial cultures were subsequently centrifuged at 6000 g for 40 min at 4 °C. The supernatant was precipitated using 40 ml of 20% (v/v) PEG 8000 and 2.5 M NaCl, followed by incubation on ice for 1 h. The white phage pellet was collected by centrifugation at 6000 g for 40 min at 4 °C and subsequently resuspended in 1–5 ml PBS for biopanning.
Three rounds of biopanning for VNAR enrichment against HpaA were performed by coating recombinant HpaA protein at 100 μL/well on 96-well plates in duplicate and incubated overnight at 4 °C. After washing with PBST (0.1% Tween-20 in PBS), the coated wells were blocked by 5% (w/v) skimmed milk in PBS (5% MPBS) at 37 °C for 2 h. Afterwards, the coated wells were added 1011 colony-forming units (CFU) phage particles pre-incubated on 5% MPBS wells at 37 °C for 1 h. After each round of phage incubation, the wells were washed 10 times with PBST. Plate washing was enhanced by adding PBST washing steps 15 (second round) and 20 (third round) times, respectively. To amplify the selected phage, the coated wells with specific phages infected 200 μL E. coli TG1 cells in the logarithmic growth phase for 30 min at 37 °C three times. The enrichment of each selection round was assessed by polyclonal phage ELISA. Briefly, similar amounts of phages of each output were added to HpaA-coated (200 ng/well) microwell plates and incubated at 37 °C for 1 h. Phage binders were detected with HRP-conjugated anti-M13 antibody (Santa Cruz, sc53004HRP) diluted 1:10000 in 5% MPBS and incubated for 1 h at 37 °C. Following three washing steps with PBST, colorimetric substrate tetramethyl-benzidine (TMB) solution (Solarbio) was added, and the reaction was stopped with 1 M H2SO4 after 10 min at room temperature. An ELISA reader (Molecular Devices, SpectraMax i3x) at 450 nm was used to measure the absorbance. The phage binders were identified by monoclonal phage ELISA, which procedure was similar to that of phage polyclonal ELISA except that phage from colonies was picked from the third round.
Expression and purification of anti-HpaA VNAR
The clones with high affinities towards antigen HpaA were chosen for expression. The VNAR fragments were subcloned into pET28a vectors with primers carrying Nco I and Not I restriction sites, and then the plasmid was transformed into E. coli BL21(DE3) cells. The VNAR expression was induced using 0.1 mM IPTG at 18 °C for 1 day when OD600 of 0.4–0.6. The proteins were extracted by sonication and the His-tagged VNARs were purified in the same procedure as for immunogen production. The non-specifically bound proteins were removed with 50 mM imidazole in PBS, and 200 mM imidazole was used to elute bound proteins. Purified recombinant proteins were concentrated using 3 kDa Ultra-filtration centrifuge tubes (Pall, MAP003C37), and the concentration of VNARs was tested using a BCA protein assay kit (Beyotime Biotechnology). Finally, the purity of the VNARs detected by SDS-PAGE.
ELISA for VNAR affinity detection
The binding capability of the VNAR to HpaA was compared by ELISA assay. The recombinant VNAR proteins were biotinylated by using biotin (NHS-LC-Biotin, Sigma) per the manufacturer’s protocol, as directed. The HpaA proteins were incubated with 10 μM biotin on ice for 2 h. Unreacted biotin was subsequently removed through a desalting column (Millipore, UFC200324). In brief, 96-well microplates were coated overnight at 4 °C with purified antigen diluted to a concentration of 4 μg/mL in PBS. Before blocking with 3% BSA at 37 °C for a duration of 2 h, the plates underwent triple washing with PBST. After brief washing with PBST, the plates were then incubated with 100 μL dilutions of VNAR protein at 37 °C for 1.5 h. The negative control for detection was achieved using 3% BSA in PBST. Plates were washed with PBST, followed by adding 100 μL of 1:10,000 streptavidin-conjugated HRP (Thermo Scientific, 21124) and incubated at 37 °C for 1 h. The reaction was terminated by the addition of 1 M H2SO4 after colour development with TMB solution, followed by washing with PBST. The absorbance was then determined with a microplate reader (Molecular Devices, SpectraMax i3x).
BLI for VNAR binding kinetics
The binding kinetics of VANRs were determined by using the Octet RED96e Analysis System (Pall, ForteBio). The HpaA proteins were biotinylated as above described. Biotinylated-HpaA was loaded onto streptavidin (SA) biosensors (Sartorius), typically 10 μg/mL for 90 s, in PBS containing 0.1% BSA and 0.002% Tween-20. Various concentrations of soluble VNAR ranging from 15.6 nM to 500 nM were added for an association step of 120 s followed by a dissociation step of 180 s. All steps were conducted in a volume of 200 µL in black 96-well microplates at room temperature. The response data were normalized using Octet data analysis software.
Whole-cell ELISA
The H. pylori 26695 bacteria from American Type Culture Collection (ATCC) were cultured on a brain-heart infusion medium (BHI) with 10% defibrinated sheep blood (Solarbio, TX0020) under a microaerobic environment (5% oxygen, 10% CO2, 85% nitrogen) at 37 °C for 2-3 days. Bacteria were harvested by centrifuging at 4 °C (12,000 g) for 5 min, washed two times with PBS, resuspended in 3% (v/v) formalin in PBS, and fixed overnight at 4 °C. After washing with PBS, the bacterial cells were coated on the 96-well plates at 107 CFU/well and left overnight at 4 °C [Citation25]. All the other steps were the same as for the ELISA above, except that VNARs were detected using HRP-anti-6 × His tag (Abcam, Ab3553) diluted with PBST containing 3% BSA.
Competition ELISA
The ELISA microplates were coated as previously described and incubated overnight at 4 °C. Subsequently, the plates were blocked with 3% BSA in PBST for 2 h at 37 °C. After the blocking step, the plates were washed three times with PBS. Microplates were incubated with 80 nM of biotinylated 2A2 alone as positive control or biotinylated 2A2 mixed with 100 µL VNARs at 5-fold dilution from 10 μM to 0.64 nM for 1 h at 37 °C. After appropriate washing, a 1:10,000 streptavidin-conjugated HRP diluted in 3%BSA in PBST was added. Following incubation at 37 °C for 1 h, the microplates were washed and the absorbance was measured the same as the above ELISA.
Computational model
The 3D structures VNAR and HpaA were predicted by SWISS-MODEL [Citation28] and reported by AlphaFold [Citation29], respectively, and the 3D structures of the models with the highest ranking were chosen. The complex model of VNARs to the HpaA protein was generated by ZDock [Citation30]. PDBePISA [Citation31] was utilized for the visualization of structures and screening for identifying interactions between VNARs and HpaA.
Construction of bivalent VNARs
To enhance the binding affinity between VNARs and HpaA, we designed homodimeric VNARs by fusing two VNARs with a flexible linker (G4S)3. Specifically, BiNb-2A2 and BiNb-3D6 were constructed, with a HA tag at the C-terminus of BiNb-3D6. The recombinant expression plasmids pET28a-BiNb-2A2 and pET28a-BiNb-3D6 were synthesized by Tsingke Biotechnology Co. Ltd. Then, the bivalent VNARs were expressed and purified as previously described.
pH stability of VNARs
To test the pH stability of these antibodies, the concentration of VNARs stock solution was diluted to 200 μg/mL with PBS containing 0.5% BSA. The experimental reactions were conducted with a reaction volume of 20 μL. To 18 μL of pH buffers with varying pH levels (1.2, 2.0, 4.0, and 7.4), 2 μL of VNARs was added and mixed by vortexing. The samples were then incubated for 1 h at 37 °C, followed by neutralization with 10 μL of 1 M Tris-HCl (pH 7.5). After the end of the reactions, all samples are stored at −80 °C before ELISA assay for VNAR affinity as described previously.
Bivalent VNAR-based sandwich-ELISA
The sandwich ELISA method was developed using paired bivalent VNARs. The capture antibody used was BiNb-2A2, while the detection antibody was BiNb-3D6, which has an HA tag. Briefly, BiNb-2A2 was diluted to 5 μg/mL with PBS and 100 μL/well was added to 96-well plates overnight at 4 °C. After blocking with 5% MPBS for 1.5 h, 100 μL of serial concentrations of HpaA protein were added to the well and incubated at 37 °C for 1 h. An equivalent volume of PBS was used as a negative control. After washing three times, 100 μL of BiNb-3D6 with an HA tag at a concentration of 1.25 μg/mL was added to each well. The plate was then incubated for 1 h at 37 °C, followed by the addition of 100 μL of anti-HA-HRP (Abcam, Ab1190) (diluted 1:5000 in 5% MPBS) to each well and incubation for 1 h. The microplates were washed and the absorbance was measured according to the ELISA assay as described previously.
Statical analyses
All data obtained from biopanning and ELISA assays were analysed with GraphPad Prism 8 software.
Results
Shark immunisation and phage display library construction
To obtain recombinant HpaA, it was expressed in the E. coli expression system and purified with Ni-NTA affinity chromatography (). After six times immunization (), the immune shark serum was evaluated using indirect ELISA. The serum was tested at 2-fold dilution from 1:50 to 1:3,200 dilutions, while pre-immune shark serum was a negative control. The serum antibody titre against HpaA reached about 1:1600 (). VNAR gene fragments were amplified from the total RNA of PBLs and spleen using PCR. The amplified fragments were subsequently cloned into the phagemid pComb3XSS. Based on 20 randomly selected colonies from the library, approximately 100% of the transformants contained a VNAR fragment of the expected size (). Finally, A phage display library consisting of 1.28 × 108 independent transformants was constructed.
Figure 1. Immunization and library construction. (a) SDS-PAGE analyses of HpaA purification. (b) The schematic depicts shark immunization. (c) Immune response in white-spotted bamboo shark evaluated by ELISA. The values showed the average of three replicates. (d) Detection of a positive rate of VNAR library phage by colony PCR; 1–20: randomly selected colonies.
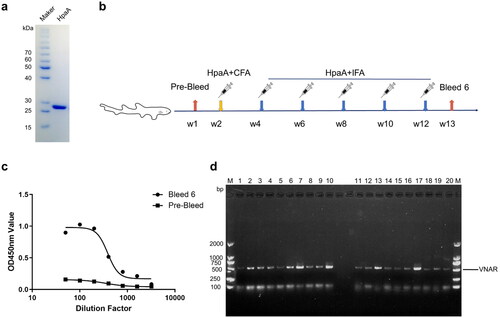
Screening of VNARs against HpaA from the immune phage display library
To obtain VNARs that bind to HpaA, we performed three rounds of biopanning of the phage display library against HpaA. After the final round of biopanning, the selected phages showed high affinity in the polyclonal phage ELISA (), and then 92 individual clones were confirmed to bind HpaA protein by monoclonal phage ELISA (). The sequences of VNARs in these individual clones were determined and aligned for comparison (). Three clones, including 2A2, 2D9, and 3D6, with unique sequences, were obtained depending on variation in their third complementarity-determining region (CDR3), suggesting that these three VNARs may recognize different antigen epitopes. All clones belong to type II VNARs based on the number and location of the conserved cysteines, which have a stabilizing disulphide bond between cysteine residues in CDR1 and CDR3 [Citation32,Citation33].
Figure 2. Screening of VNAR against HpaA from the immune library. (a) Polyclonal phage ELISA for detection of enrichment in each round of biopanning. (b) Monoclonal phage ELISA for detecting anti-HpaA phage. (c) The amino acid sequences of the selected VNARs. FR is the framework region; HV is a hypervariable region. Canonical cysteine residues are red.
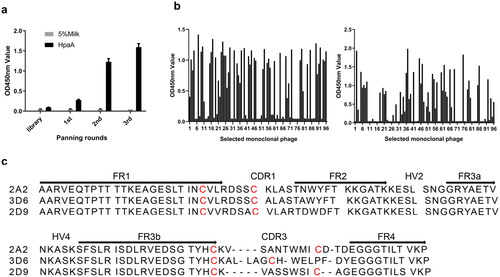
Expression and purification of VNARs
To produce recombinant VNARs, the three VNAR genes (2A2, 2D9, and 3D6) were subcloned into the pET28a expression vector and transformed into E. coli BL21(DE3) strain (). The three VNARs with 6 × His tags at their C-termini were expressed and subsequently purified by IMAC (). We quantified the amount of VNAR obtained after purification in yields ranging between 5–20 mg/L. SDS-PAGE with Coomassie staining confirmed the purity and the expected molecular weight (15 kDa), as shown in . The low cost and high yield of VNAR production from the E. coli system represent a significant advantage.
Figure 3. Expression and purification of anti-HpaA VNARs. (a) Schematic diagram of VNAR produced in E. coli BL21(DE3) strain. (b) SDS-PAGE analysis of expression and purification of VNAR. Lane M: protein marker, Lane 1: supernatant of the bacteria lysate, Lane 2: penetrating liquid, Lane 3-5: washing buffer with 20, 50, and 75 mM imidazole, and Lane 6-10: elution buffer with 100, 150, 200, 250, 300 mM imidazole; (c) SDS-PAGE analysis of the molecular weight of anti-HpaA VNAR purification.
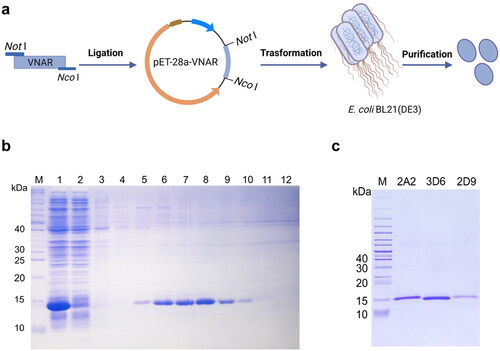
VNAR affinity determination
To evaluate the binding affinity of VNARs to HpaA, both indirect ELISA and BLI experiments were conducted. For the ELISA assay, HpaA antigens were coated onto the plate and purified VNARs were added at various concentrations. The results demonstrated that three monovalent VNARs, namely 2A2, 3D6, and 2D9, bound well to the recombinant HpaA protein with an EC50 of 1.43 nM, 94.61 nM, and 46.34 nM, respectively (). In addition to the ELISA data, the kinetic rate constants of the VNARs were determined using BLI analysis. Among them, VNAR 2A2 and 3D6 exhibited high affinity against HpaA, with an equilibrium dissociation constant (KD) of 7.73 nM and 69.3 nM, respectively (,c) and ). However, ELISA result for VNAR 2D9 was higher than the BLI result (,d) and ), probably due to the spatial effect of chemically labelled biotin on the interaction of the antigen HpaA with VNAR in the BLI assay. Generally, the KD values obtained by both BLI and ELISA assays were in good agreement.
Figure 4. The binding of VNARs to HpaA. (a) ELISA Measurement of 3 isolated VNARs against HpaA. The values were the mean ± standard deviation of three well replicates. (b)-(d) The BLI binding kinetics of 2A2 (b), 3D6 (c), 2D9 (d), respectively.
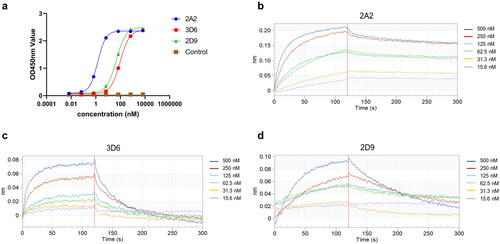
Table 1. Binding kinetics of VNARs selected against HpaA.
Binding of VNARs to H. pylori bacteria
ELISA was used to determine the binding of VNARs to native HpaA protein. H. pylori 26695 was inactivated and coated on the 96-well plates, and ELISA was performed with different concentrations of VNARs. The results indicated that the VNARs 2A2, 3D6, and 2D9 could bind to H. pylori 26695 bacteria (), indicating that these antibodies can bind to native HpaA protein.
Model of VNAR–HpaA complex
Competitive ELISA experiments were conducted to investigate whether the three VNARs bind to the same or different epitopes. The results shown in indicate that 2A2 competes partially with 2D9, while 2A2 does not compete with 3D6. These findings suggest that 2A2 and 3D6, which exhibit high affinities, likely recognize different binding epitopes.
Figure 6. Model of VNAR-HpaA complex. (a) Competitive ELISA of biotinylated 2A2 vs. 2A2, 3D6, 2D9 on HpaA. (b) Structure representations of HpaA and 2A2; HpaA is coloured cyan, and 2A2 is coloured blue. (c) Stereo view of HpaA-2A2 interactions; Hydrogen bonding is coloured red. (d) Structure representations of HpaA and 3D6, HpaA is coloured cyan, and 3D6 is coloured yellow. (e) Stereo view of HpaA-3D6 interactions; Hydrogen bonding is coloured red.
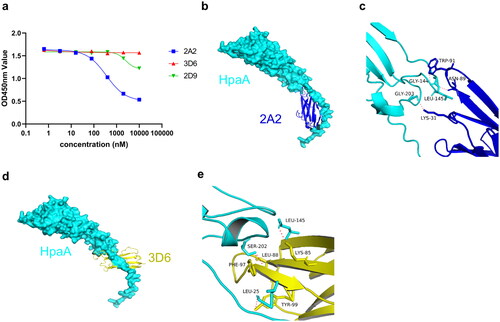
Molecular docking was utilized to investigate the binding mechanisms of VNAR 2A2 and 3D6 with the HpaA antigen and to identify key residues involved in the VNAR–HpaA interactions. The structural representations are depicted in and c), and the results show that LYS31, ASN89, and TRP91 residues in 2A2 form hydrogen bonds with GLY144, LEU145, and GLY203 residues in HpaA, respectively. In addition, 3D6 binds to HpaA, with polar interactions between LYS85, LEU88, PHE97, and THY99 residues in the CDR3 of VNAR and LEU25, LEU145, and SER202 residues in HpaA (). Previous studies have suggested that HpaA may recognize potential glycolipid receptors via the residues 139–153 in the extracellular adhesion domain of the HpaA protein [Citation34]. Therefore, VNAR 2A2 and 3D6 may have the potential to block the binding of HpaA to its receptors. Further studies are needed to validate these speculations.
Characterization of bivalent VNARs
To enhance the binding affinity of the VNARs, we constructed bivalent VNARs, BiNb-2A2 and BiNb-3D6 (). The bivalent VNARs were expressed in E. coli BL21(DE3) and subsequently purified. As shown in , a single protein band was detected, which corresponds well with the theoretical molecular weight of the bivalent VNAR. BiNb-2A2 and BiNb-3D6 showed significantly enhanced binding affinity to HpaA, with EC50 values of 0.62 nM and 0.40 nM by indirect ELISA (). The formation of bivalent VNARs increased the binding affinity of 2A2 and 3D6 by 2-fold and 230-fold, respectively.
Figure 7. Characteristics of bivalent VNAR. (a) Schematic representation of bivalent VNAR. (b) SDS-PAGE analysis of bivalent VNARs purified. (c) ELISA analysis of binding affinity of bivalent VNARs to HpaA. (d) The binding capability of bivalent VNARs to HpaA in different pH buffers. The values were the mean ± SD of three replicates.
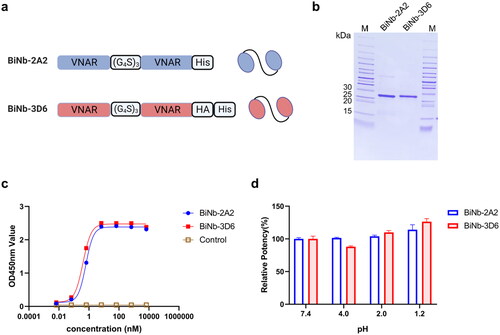
To estimate the pH stability of VNARs, we determined the binding capability of the bivalent VNAR in buffers simulating the gastrointestinal pH conditions. As shown in , BiNb-2A2 and BiNb-3D6 maintained antigen-binding activity at the conditions of pH 1.2, pH 2.0, and pH 4.0. The binding activity of BiNb-2A2 was found to be slightly higher at pH 1.2 and pH 2.0 as compared to pH 7.4. The observed increase in binding activity at acidic pH values could potentially be attributed to a conformational alteration of VNAR, which could have resulted in increased affinity towards the target molecule. The binding activities of BiNb-3D6 remained at least 85% at pH 4.0 and these results indicate that BiNb-2A2 and BiNb-3D6 can be stable in the condition of gastrointestinal pH.
Bivalent VNAR-based sandwich-ELISA
To investigate the potential use of bivalent VNARs in detecting H. pylori, a sandwich ELISA was developed. BiNb-2A2 was used as the capture and BiNb-3D6 as the detection (). The results showed a good linear correlation between the logarithm of HpaA concentrations (ranging from 0.26 to 68.96 nM) and the absorbance value at 450 nm. The standard linear equation was y = 0.7727 x + 0.6504 (R2 = 0.9860) (). The limit of detection (LOD) was calculated using the above linear equation and was determined to be 0.18 nM. These findings suggest that the combination of BiNb-2A2 and BiNb-3D6 is effective in detecting H. pylori antigen HpaA.
Discussion
H. pylori, a gastrointestinal pathogen, is implicated in the development of various stomach diseases. HpaA is an adhesin that plays an essential role in the infection of gastric mucosa by H. pylori and is a potential key target for the diagnosis and treatment of H. pylori infection. In this study, we isolated three high-affinity VNARs (2A2, 3D6, and 2D9) against the HpaA protein from a shark VNAR immune phage display library. The VNARs were efficiently produced as soluble recombinant proteins with high yields using the E. coli expression system, which showed high binding capability to H. pylori. The VNARs 2A2 and 3D6 were found to recognize different epitopes of the recombinant HpaA protein. Furthermore, the formation of homodimeric bivalent VNARs significantly increased the binding affinity of 2A2 and 3D6, which could be used for H. pylori detection. This can be attributed to the fact that bivalent antibodies possess two complementarity-determining regions that can simultaneously bind to two HpaA molecules. Also, we speculated that the fusion of two VNAR proteins may stabilize the conformation of the bivalent antibody. In addition, these bivalent antibodies could maintain binding activity at the extreme pH of the simulated gastrointestinal environment, suggesting potential oral administration therapeutic application. The bivalent VNARs were used to establish a sandwich ELISA for the HpaA antigen quality detection.
Several conventional monoclonal antibodies targeting different antigens of H. pylori, such as whole-cell bacteria, urease, and vacuolating cytotoxin A, have been developed, demonstrating the ability to impact bacterial adhesion and colonization [Citation19,Citation21,Citation35,Citation36]. However, conventional antibodies are sensitive to the extreme pH environment and pepsin degradation in the gastrointestinal tract [Citation37], and there is poor consistency between batches of antibody preparation. In contrast, VNARs possess several remarkable characteristics, such as stability, ease of modification and production, large-scale preparation with E. coli or yeast recombinant protein expression systems, and also ensure batch-to-batch consistency [Citation38]. Therefore, these VNARs had the potential as a promising tool for H. pylori diagnosis and treatment.
HpaA, encoded by the hpaA gene, is an outer membrane protein belonging to the N-acetylneuraminyl lactose-binding fibrin family. It plays a crucial role in bacterial colonization of the gastrointestinal tract, leading to the damage of gastric mucosa [Citation39]. Elisabet et al. conducted an experiment where H. pylori CCUG 17874 with the HpaA mutant gene was administered to mice via gavage. The study demonstrated that the mutant strain failed to colonize the mice’s stomachs, indicating the essentiality of HpaA as a virulence factor for H. pylori colonization in mice [Citation16]. Interestingly, previous research suggested that HpaA specifically recognized sialic acid, but it has been reported that HpaA protein can bind not only to salivated fetuin but also to non-sialylated asialofetuin [Citation40]. The specific mechanism regarding the function of HpaA in the adhesion and colonization of H. pylori in the gastric mucosa is not clear and still requires further investigation. Consequently, the impact of VNAR against HpaA cannot be assessed in vitro yet. Future studies should explore whether VNAR can impede H. pylori colonization in animal models of infection and elucidate its underlying mechanism.
HpaA is a significant detection target due to its extensively conserved nucleotide and amino acid sequences. It exhibits remarkable conservation across different H. pylori strains with limited homology to other proteins. The study constructed two bivalent VNARs with high affinities that recognized different binding epitopes. Subsequently, a sandwich ELISA was constructed for antigen quality detection that uses BiNb-2A2 as capture antibodies and BiNb-3D6 as detection antibodies. The quantification of HpaA indicated the promising potential of this assay for the detection of H. pylori infection. Moreover, these high-affinity and paired VNARs hold the potential for the development of other highly sensitive assays targeting H. pylori infection.
Author contributions
Y.G. and Y.W. conceptualised this research. Y. Gao, L.L., and R.W. performed the experiments. S.F., Y.W., X.X., and W.Y. collected and analysed the data. Y. Gao and Y.G. wrote the manuscript. Y. Gao, W.Y., and Y.G. were responsible for the accuracy of the study and the review of the article. All authors have read and agreed to the published version of the manuscript.
Ethics approval
All aspects of this study were approved by the Institutional Research Ethics Committee of Ocean University of China (Ethical Approval Numbers OUC-AE-2021-156).
Abbreviations | ||
BHI | = | brain-heart infusion medium |
BLI | = | Bio-Layer Interferometry |
CDR | = | complementarity-determining region |
CFU | = | colony forming units |
HpaA | = | H. pylori adhesin A |
WHO | = | World Health Organisation |
LOD | = | limit of detection |
IPTG | = | isopropyl β-D-1-thiogalactopyranoside |
NLBH | = | neuraminyllactose-binding haemagglutinin |
PBLs | = | peripheral blood lymphocytes |
VNAR | = | variable domain of immunoglobulin new antigen receptor. |
Acknowledgments
We thank the College of Food Science and Engineering, Ocean University of China for the use of the BLI instrument.
Disclosure statement
No potential conflict of interest was reported by the authors.
Data availability statement
The data that support the findings of this study are available from the corresponding author, Y.G., upon reasonable request.
Additional information
Funding
References
- Kakelar HM, Barzegari A, Dehghani J, et al. Pathogenicity of Helicobacter pylori in cancer development and impacts of vaccination. Gastric Cancer. 2019;22(1):23–36. doi: 10.1007/s10120-018-0867-1.
- Marshall BJ, Warren JR. Unidentified curved bacilli in the stomach of patients with gastritis and peptic ulceration. Lancet. 1984;1(8390):1311–1315. doi: 10.1016/s0140-6736(84)91816-6.
- Jiang J, Chen Y, Shi J, et al. Population attributable burden of Helicobacter pylori-related gastric cancer, coronary heart disease, and ischemic stroke in China. Eur J Clin Microbiol Infect Dis. 2017;36(2):199–212. doi: 10.1007/s10096-016-2810-x.
- Kusters JG, van Vliet AH, Kuipers EJ. Pathogenesis of Helicobacter pylori infection. Clin Microbiol Rev. 2006;19(3):449–490. doi: 10.1128/CMR.00054-05.
- Ansari S, Yamaoka Y. Helicobacter pylori virulence factors exploiting gastric colonization and its pathogenicity. Toxin. 2019;11(11):677. doi: 10.3390/toxins11110677.
- Santos MLC, de Brito BB, da Silva FAF, et al. Helicobacter pylori infection: beyond gastric manifestations. World J Gastroenterol. 2020;26(28):4076–4093. doi: 10.3748/wjg.v26.i28.4076.
- Yang JC, Lu CW, Lin CJ. Treatment of Helicobacter pylori infection: current status and future concepts. World J Gastroenterol. 2014;20(18):5283–5293. doi: 10.3748/wjg.v20.i18.5283.
- Graham DY, Shiotani A. New concepts of resistance in the treatment of Helicobacter pylori infections. Nat Clin Pract Gastroenterol Hepatol. 2008;5(6):321–331. doi: 10.1038/ncpgasthep1138.
- Talebi Bezmin Abadi A. Vaccine against Helicobacter pylori: inevitable approach. World J Gastroenterol. 2016;22(11):3150–3157. doi: 10.3748/wjg.v22.i11.3150.
- Suerbaum S. Genetic variability within Helicobacter pylori. Int J Med Microbiol. 2000;290(2):175–181. doi: 10.1016/S1438-4221(00)80087-9.
- Huang Y, Wang QL, Cheng DD, et al. Adhesion and invasion of gastric mucosa epithelial cells by Helicobacter pylori. Front Cell Infect Microbiol. 2016;6:159. doi: 10.3389/fcimb.2016.00159.
- Bonsor DA, Sundberg EJ. Roles of adhesion to epithelial cells in gastric colonization by Helicobacter pylori. Adv Exp Med Biol. 2019;1149:57–75.
- Evans DG, Karjalainen TK, Evans DJ, Jr., et al. Cloning, nucleotide sequence, and expression of a gene encoding an adhesin subunit protein of Helicobacter pylori. J Bacteriol. 1993;175(3):674–683. doi: 10.1128/jb.175.3.674-683.1993.
- Lundström AM, Blom K, Sundaeus V, et al. Hpaa shows variable surface localization but the gene expression is similar in different Helicobacter pylori strains. Microb Pathog. 2001;31(5):243–253. doi: 10.1006/mpat.2001.0466.
- Kavermann H, Burns BP, Angermuller K, et al. Identification and characterization of Helicobacter pylori genes essential for gastric colonization. J Exp Med. 2003;197(7):813–822. doi: 10.1084/jem.20021531.
- Carlsohn E, Nyström J, Bölin I, et al. Hpaa is essential for Helicobacter Pylori colonization in mice. Infect Immun. 2006;74(2):920–926. doi: 10.1128/IAI.74.2.920-926.2006.
- Banga Ndzouboukou JL, Lei Q, Ullah N, et al. Helicobacter pylori adhesins: hpaa a potential antigen in experimental vaccines for H. pylori. Helicobacter. 2021;26(1):e12758. doi: 10.1111/hel.12758.
- Xie W, Zhao W, Zou Z, et al. Oral multivalent epitope vaccine, based on ureb, hpaa, cat, and ltb, for prevention and treatment of Helicobacter pylori infection in C57bl/6 mice. Helicobacter. 2021;26(3):e12807. doi: 10.1111/hel.12807.
- Fahimi F, Sarhaddi S, Fouladi M, et al. Phage Display-Derived antibody fragments against conserved regions of vaca toxin of Helicobacter pylori. Appl Microbiol Biotechnol. 2018;102(16):6899–6913. doi: 10.1007/s00253-018-9068-4.
- Aiba Y, Umeda K, Rahman S, et al. Synergistic effect of anti-Helicobacter pylori urease immunoglobulin Y from egg yolk of immunized hens and Lactobacillus johnsonii no.1088 to inhibit the growth of Helicobacter pylori in vitro and in vivo. Vaccine. 2019;37(23):3106–3112. doi: 10.1016/j.vaccine.2019.04.045.
- Fouladi M, Sarhadi S, Tohidkia M, et al. Selection of a fully human single domain antibody specific to Helicobacter pylori urease. Appl Microbiol Biotechnol. 2019;103(8):3407–3420. doi: 10.1007/s00253-019-09674-6.
- Zhang L, Xiao Y, Ji L, et al. Potential therapeutic effects of egg yolk antibody (igy) in Helicobacter pylori infections-a review. J Agric Food Chem. 2021;69(46):13691–13699. doi: 10.1021/acs.jafc.1c05398.
- Juma SN, Gong X, Hu S, et al. Shark new antigen receptor (ignar): structure, characteristics and potential biomedical applications. Cells. 2021;10(5):1140. doi: 10.3390/cells10051140.
- Camacho-Villegas T, Mata-Gonzalez T, Paniagua-Solis J, et al. Human tnf cytokine neutralization with a vnar from heterodontus francisci shark: a potential therapeutic use. MAbs. 2013;5(1):80–85. doi: 10.4161/mabs.22593.
- Kandalaft H, Hussack G, Aubry A, et al. Targeting Surface-Layer proteins with Single-Domain antibodies: a potential therapeutic approach against Clostridium Difficile-Associated disease. Appl Microbiol Biotechnol. 2015;99(20):8549–8562. doi: 10.1007/s00253-015-6594-1.
- Ebrahimizadeh W, Mousavi Gargari S, Rajabibazl M, et al. Isolation and characterization of protective anti-Lps nanobody against V. Cholerae O1 recognizing inaba and ogawa serotypes. Appl Microbiol Biotechnol. 2013;97(10):4457–4466. doi: 10.1007/s00253-012-4518-x.
- Zhao L, Chen M, Wang X, et al. Identification of anti-Tnfα vnar single domain antibodies from whitespotted bambooshark (Chiloscyllium plagiosum). Mar Drugs. 2022;20(5):307.
- Waterhouse A, Bertoni M, Bienert S, et al. Swiss-Model: homology modelling of protein structures and complexes. Nucleic Acids Res. 2018;46(W1):W296–w303. doi: 10.1093/nar/gky427.
- Jumper J, Evans R, Pritzel A, et al. Highly accurate protein structure prediction with alphafold. Nature. 2021;596(7873):583–589. doi: 10.1038/s41586-021-03819-2.
- Pierce BG, Wiehe K, Hwang H, et al. Zdock server: interactive docking prediction of Protein-Protein complexes and symmetric multimers. Bioinformatics. 2014;30(12):1771–1773. doi: 10.1093/bioinformatics/btu097.
- Krissinel E, Henrick K. Inference of macromolecular assemblies from crystalline state. J Mol Biol. 2007;372(3):774–797. doi: 10.1016/j.jmb.2007.05.022.
- Matz H, Dooley H. Shark Ignar-Derived binding domains as potential diagnostic and therapeutic agents. Dev Comp Immunol. 2019;90:100–107. doi: 10.1016/j.dci.2018.09.007.
- Cheong WS, Leow CY, Abdul Majeed AB, et al. Diagnostic and therapeutic potential of shark variable new antigen receptor (vnar) single domain antibody. Int J Biol Macromol. 2020;147:369–375. doi: 10.1016/j.ijbiomac.2020.01.039.
- Fantini J, Garmy N, Yahi N. Prediction of Glycolipid-Binding domains from the amino acid sequence of lipid Raft-Associated proteins: application to hpaa, a protein involved in the adhesion of Helicobacter Pylori to gastrointestinal cells. Biochemistry. 2006;45(36):10957–10962. doi: 10.1021/bi060762s.
- Hong KS, Ki MR, Ullah HMA, et al. Preventive effect of anti-Vaca egg yolk immunoglobulin (igy) on Helicobacter pylori-Infected mice. Vaccine. 2018;36(3):371–380. doi: 10.1016/j.vaccine.2017.11.082.
- Shin JH, Yang M, Nam SW, et al. Use of egg Yolk-Derived immunoglobulin as an alternative to antibiotic treatment for control of Helicobacter Pylori infection. Clin Diagn Lab Immunol. 2002;9(5):1061–1066. doi: 10.1128/cdli.9.5.1061-1066.2002.
- Kovacs-Nolan J, Mine Y. Microencapsulation for the gastric passage and controlled intestinal release of immunoglobulin Y. J Immunol Methods. 2005;296(1-2):199–209. doi: 10.1016/j.jim.2004.11.017.
- Valdovino-Navarro BJ, Dueñas S, Flores-Acosta GI, et al. Neutralizing ability of a single domain vnar antibody: in vitro neutralization of Sars-Cov-2 variants of concern. Int J Mol Sci. 2022;23(20):12267.
- O'Toole PW, Janzon L, Doig P, et al. The putative Neuraminyllactose-Binding hemagglutinin hpaa of Helicobacter pylori ccug 17874 is a lipoprotein. J Bacteriol. 1995;177(21):6049–6057. doi: 10.1128/jb.177.21.6049-6057.1995.
- Lundstrom A, Bolin I, Bystrom M, et al. Recombinant hpaa purified from Escherichia Coli has biological properties similar to those of native Helicobacter pylori hpaa. APMIS. 2003;111(3):389–397. doi: 10.1034/j.1600-0463.2003.t01-1-1110203.x.