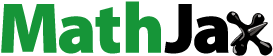
ABSTRACT
Novel ultra-low firing (Mg1-xZnx)V2O6(x = 0–0.09) ceramics have been investigated and systematically designed as promising dielectrics for ultra-low temperature co-fired ceramics applications. The X-ray diffraction patterns indicated that all samples crystallized in a monoclinic structure with a space group of C2/m. Microstructures and lattice parameters are presented and correlated with the microwave dielectric properties of the ceramics. The MgV2O6 specimen can be well-sintered at 610°C, the resulting ceramic of which featured an εr ~ 8.8, a Q × f ~ 10,300 GHz and a τf ~ – 29.7 ppm/°C. The tunable τf value can be adjusted to near zero along with a great promotion in the Q × f of MgV2O6 by using a small Zn substitution. In addition, excellent microwave dielectric properties (εr ~ 9.3, Q × f ~ 15,300 GHz @15.5 GHz and τf ~ – 3.4 ppm/°C) were obtained for the (Mg0.93Zn0.07) V2O6specimen sintered at 610°C for 4 h. The specimen is chemically compatible with Al electrodes and could be an ideal ultra-low temperature co-fired ceramics dielectric. Possible applications include the 5 G system, particularly with high frequency regions.
1. Introduction
With the progress of today’s wireless communication systems, the requirement of Low Temperature Co-fired Ceramics (LTCC) technology to miniaturize and integrate microwave passive components and devices is more needed than ever. Major requisites for LTCC dielectrics include a low dielectric constant (εr<10) to reduce the wave propagation delay of the system, a high Q × f value to lower the dielectric loss at high frequency operation, and a near-zero temperature coefficient of resonant frequency (τf) to limit the resonator frequency shift. For energy conservation, Al (melting point at 660°C) is normally preferred and selected as the electrode material for Ultra-Low Temperature Co-firing Ceramics (ULTCC) applications. Consequently, a < 660°C sintering temperature is required for the dielectrics to prevent the electrode from melting. To this end, glass addition and sol-gel processing are usually employed to reduce the sintering temperature of dielectric ceramics. However, glass-added sintering also degrades the microwave dielectric properties of ceramics. Moreover, a costly, time-consuming, and complex processing is required for the sol-gel method. Most of the ULTCC reveal a negative τf value [Citation1–3]. To compensate the negative τf value, dielectrics with a positiveτf, which also means a high εr and a low Q × f, are often added to the ceramics, resulting in the combined microwave dielectric properties also being distorted, thereby limiting possible applications at high frequency regimes, such as a 5G system [Citation4,Citation5]. For instance, high εr (>10) dielectrics increase the wave propagation delay of devices for high frequency applications. Therefore, the search for new dielectrics, particularly with a stable τf, for ULTCC applications has become a more urgent issue.
A number of V2O5-based ceramic families have been studied and reported for LTCC applications over the last decade. For instance, BaV2O6 and Ba3V4O13, belonging to the BaO-V2O5 family, have been reported to be good candidates for ULTCC applications [Citation6,Citation7]. The R2V2O7 (R = Ba, Sr and Ca), BaO-MO-V2O5 (M = Mg, Zn), and SrMV2O7 (M = Mg, Zn) families have also been found to offer good microwave dielectric properties at ultra-low sintering temperatures [Citation8,Citation9]. Moreover, Mg3(VO4)2 and BaMg2(VO4)2 have been proposed as suitable LTCC dielectrics [Citation10–12]. Nevertheless, they possess a large negativeτf, which limits their practical applications.
In this paper, a novel ULTCC (Mg1-xZnx)V2O6 (x = 0–0.09) dielectric with a near-zero τf was investigated. In doing so, the X-ray Diffraction (XRD) patterns and surface morphology were studied. Additionally, the compatibility of co-firing with an Al electrode was also reported.
2. Materials and methods
V2O5, MgO, and ZnO powders (>99.9%) were mixed according to the(Mg1-xZnx)V2O6 (x = 0–0.09) composition. The powders were ball-milled for 24 h, dried and calcined at 500°C for 2 h. The mixtures, together with PVA binder, were subsequently granulated and uniaxially pressed into pellets with 5 mm in thickness and 11 mm in diameter at 2000 kgf/cm2. All samples were then sintered at 520–640°C for 4 h. The subsequent phase identification of the specimens was conducted by XRD pattern analysis using a Siemens D5000 diffractometer (Munich, Germany) with Cu-Kα radiation (at 40kV and 40 mA). Thermal Gravity-Differential Scanning Calorimetry (TG-DSC) analyses (NETZSCH-STA409PC) were employed in analyzing the phase formation of the samples. The microstructures and the compatibility of co-firing with Al were studied using ultra-high resolution scanning electron microscopy (UHR–SEM; HitachiSU-8000) equipped with energy dispersion spectroscopy (EDS, Philips). The Archimedes method was applied to measure the densities of the specimens. Dielectric constants and Q × f values of the samples were measured using the Hakki-Coleman [Citation13] and Courtney [Citation14] methods on an Agilent 8364A network analyzer (USA). The same technique was applied to measure the τf value and can be expressed by
Where f1 and f2 represent the resonant frequencies at T1 and T2, respectively. All specimens were measured at 25–80°C. Rietveld refinement was completed by using the General Structure and Analysis System(GSAS).
3. Results and discussion
illustrates the TG-DSC curves of the MgV2O6 precursor powders performed by the thermal analyzer. There are two mass losses of the ceramic, the first of which constitutes a total weight loss of 3.7% occurring before reaching 258°C, and the second major weight loss of 10.2% observed during the second stage from 258°C to 450°C. Further increases in the temperature led to no additional obvious weight losses, suggesting that the formation of the MgV2O6 phase was complete. According to the DSC curve, the small endothermic peak appearing at 185°C was most likely caused by free-water evaporation. The second endothermic peak occurring at 245°C along with weight loss was due to the evaporation of crystal water or the decomposition of Mg(OH)2. In addition, an endothermic peak appearing at 415°C was due to the formation of the MgV2O6 phase at the final stage. As can be seen, there are some exothermic peaks observed between 450 and 550°C corresponding to the heat-release process of adjusting the lattice structure in the late stage of the reaction. Based upon the TG-DSC curves, the calcination temperature of the MgV2O6 precursor powders was then chosen to range from 450–550°C.
The XRD patterns of the (Mg1-xZnx)V2O6 (x = 0–0.09) ceramics recorded at room temperature are shown in . The specimens exhibited a monoclinic structure with a space group of C2/m [Citation11] in accordance with the standard ICDD-PDF #01-071-1651. Additionally, no second phase was detected in all cases, suggesting the formation of a solid solution. With an increase in x, peaks shifted to a lower angle due to the replacement of Mg2+ (0.72 Å, CN = 6) by the larger Zn2+ (0.74 Å, CN = 6). In addition, no peak shift was observed for the (Mg0.93Zn0.07)V2O6 specimen at various temperatures, indicating that the influence of the sintering temperature on structure could be excluded. However, the full width at half maximum (FWHM) decreased as the sintering temperature increased, which implies better crystallinity. In order to further clarify the crystal structure of the (Mg1-xZnx)V2O6 (x = 0–0.09) ceramics, GSAS software was used to conduct Rietveld refinements. illustrates the resultant refined lattice parameters, molecular volume, reliability factors, and goodness of fit indicators. As seen, the variations of lattice parameters and cell volumes were limited and within 1%, and the goodness of fit indicator values (χ2) were in the range 0.98–1.28, which suggests that the structural model is valid along with reliable refinement results. Moreover, the refined plot of the specimen using (Mg0.93Zn0.07)V2O6 at 610°C is illustrated in , which shows that the identical results were obtained as those in . These results also confirmed the formation of a single monoclinic structure phase.
Table 1. The refined lattice parameters, molecular volume, goodness of fit, and reliability factors, of the (Mg1-xZnx)V2O6 ceramics sintered at 610°C
Figure 2. Room temperature XRD patterns recorded from(Mg1-xZnx)V2O6 ceramics with (a) x = 0.07 at different temperatures and (b) x = 0–0.09 at 610°C
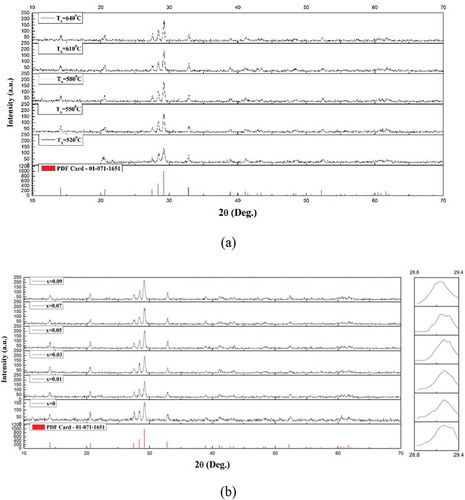
The surface micro-morphology of the (Mg1-xZnx)V2O6 (x = 0–0.09) ceramics is presented in . A porous microstructure was observed for the (Mg0.93Zn0.07)V2O6 ceramic specimen at 520°C. As the sintering temperature was increased, the number of pores decreased and a well-densified morphology was achieved at 610°C. In addition, rapid grain growth occurred at 640°C, indicating an over-sintering temperature for the specimen. Obviously, the grains exhibited a typical polygonal morphology for all specimens. Large grains and small grains co-existed, with both corresponding to a single MgV2O6 phase, as further confirmed by the EDS results (). Moreover, specimens with x = 0 and 0.01 showed rough grain boundaries, which were improved as x was increased, with smooth grain boundaries being observed for x > 0.03, suggesting Zn may aid the sintering process. In addition, the specimen with x = 0.09 was found to have an obviously larger grain size and a less uniform morphology compared to that of x = 0.07, which may have negative effects on the Q × f values of the specimen.
Table 2. Corresponding EDS results of )
Figure 4. SEM images of (Mg1-xZnx)V2O6 sintered at (a) 520°C (b) 550°C (c) 580°C (d) 610°C (e) 640°C and of (Mg1-xZnx)V2O6 with (f) x = 0 (g) x = 0.01 (h) x = 0.03 (i) x = 0.05 (j) x = 0.07 (k) x = 0.09 sintered at 610°C
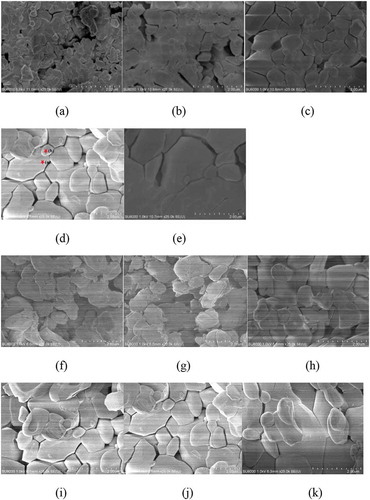
The relative densities of the monoclinic (Mg1-xZnx)V2O6 (x = 0–0.09) ceramics sintered at different temperatures are demonstrated in . The low relative density of 87%–91% observed for specimens sintered at 520°C was most likely a result of the porous morphology, as shown in . However, the density initially increased with increases in sintering temperature, and then decreased after reaching a maximum at 610°C. In all cases, the optimal sintering temperature was 610°C. A high relative density of 95.3% was obtained for the specimen with x = 0.07 sintered at 610°C.
Figure 5. Relative densities of the(Mg1-xZnx)V2O6 (x = 0–0.09) ceramics at different sintering temperatures
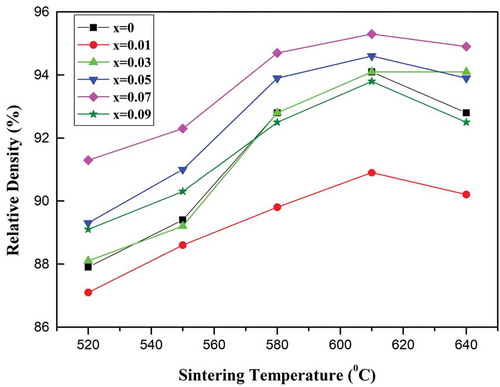
illustrates the plots of εr as a function of temperature for the (Mg1-xZnx)V2O6(x = 0–0.09) ceramics. The variations in εr were consistent with those of the relative density, indicating that density might be a primary factor affecting εr in this experiment. Moreover, a maximum εr value of ~9.3 was achieved corresponding to the highest relative density of 95.3% for the x = 0.07 specimen sintered at 610°C.Shannon reported that εr is proportional to the αD, and can be expressed by the Clausius–Mosotti equation [Citation15]:
where Vm is the molecular volume, εrc represents the corrected dielectric constant, and the constant value b = 4π/3. According to EquationEqn. (2(2)
(2) ), εrc increases when αD increases. As shown in , the variation of εrc is inversely proportional to that of Vm for x = 0–0.05, which is because the amount of Zn substitution is small. However, the change in εrc becomes consistent with that of αD at x = 0.07–0.09 due to the fact that Zn2+ (1.7 Å3) has a larger polarizability than Mg2+ (0.651Å3), which dominates the variation of εr in (Mg1-xZnx)V2O6 ceramics.
demonstrates the Q × f values of the (Mg1-xZnx)V2O6 (x = 0–0.09) ceramics sintered at various temperatures. Both intrinsic and extrinsic losses are included in the overall measured microwave dielectric losses. Typical intrinsic loss is mainly a result of the lattice vibration modes; extrinsic loss, however, can be related to density, second phases, impurities, surface morphology, and the lattice defects [Citation16,Citation17]. Accordingly, the Q × f increased with increases in sintering temperature, reaching a maximum at 610°C, which corresponds to the optimal sintering temperature mentioned earlier, and decreased thereafter. Moreover, the variations of Q × f for specimens with x = 0–0.09 at 610°C was also consistent with those of the relative density, implying that the dielectric loss of specimens was mainly attributed to the extrinsic loss corresponding to the densification of ceramics. Compared to the x = 0 specimen, the x = 0.07 specimen exhibited a 50% increase in the Q × f and a maximum value of 15,300 GHz @15.5 GHz could be achieved for the (Mg0.93Zn0.07)V2O6 specimen sintered at 610°C.
Figure 7. Q × f values of the (Mg1-xZnx)V2O6 (x = 0–0.09) ceramics at different sintering temperatures
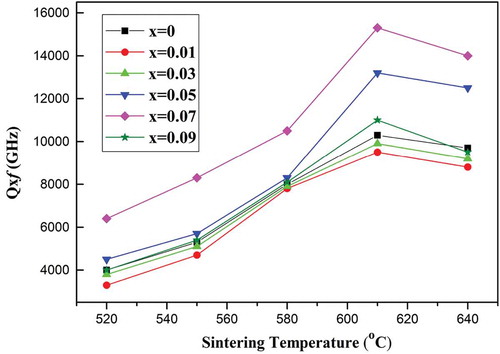
illustrates τf values of the (Mg1-xZnx)V2O6 (x = 0–0.09) ceramics sintered at different temperatures. Since there is no second phase in the pure monoclinic specimen, the remarkable shift of theτf values from −38.7 to −3.64 ppm/°C in the (Mg1-xZnx)V2O6 (x = 0–0.09) ceramics was mainly attributable to the Zn2+ substitution for Mg2+. By tuning the x to 0.07, the specimen had a τf of −3.64 ppm/°C at 610°C.
The microwave dielectric properties and sintering temperatures of some typical ceramics for ULTCC applications are listed in . All the dielectrics can be co-fired with aluminum. However, the first four compositions reveal a large τf value that limits their practical applications. In the next two ceramics, the τf was modified to a near-zero value; yet, it still suffers from a high εr, constraining its high frequency applications. In addition, tellurium oxide is toxic and not preferred for use in components. In comparison, the proposed (Mg0.93Zn0.07)V2O6 specimen not only has a near-zero τf, it also exhibits a moderate Q × f and a low εr, making it a very promising dielectric for ULTCC applications, particularly for the high frequency 5G system.
Table 3. Microwave dielectric properties and sintering temperatures of some typical ceramics for ULTCC applications
For ULTCC applications, illustrates the EDS line scan and corresponding SEM photo of the interface between the Al electrode and the (Mg0.93Zn0.07)V2O6 specimen co-fired at 610°C. As seen, a sharp decrease in the Al profile at the interface implies that Al did not diffuse into the ceramic during co-firing. Moreover, a very low amount of Mg and V in the Al electrode also suggests that no reaction occurred at the interface. Since the specimen revealed a chemical compatibility with Al, it is further confirmed as an ideal candidate dielectric for ULTCC applications.
4. Conclusion
The (Mg1-xZnx)V2O6 (x = 0–0.09) ceramics were prepared via the conventional solid-state route. Specimens sintered at 520–640°C for 4 h exhibited a monoclinic structure with a space group of C2/m without evidence of any additional second phase, suggesting the formation of a solid solution. The microwave dielectric properties were correlated to the microstructures, the temperatures, and the relative densities of the ceramics. The (Mg0.93Zn0.07)V2O6 ceramic reveals an εr of 9.3, a Q × f of 15,300 GHz and a τf of −3.4 ppm/°C. The EDS line scan of the (Mg0.93Zn0.07)V2O6 sample co-fired with aluminum also shows an excellent chemical compatibility and is presented as an ideal candidate dielectric for ULTCC applications.
Acknowledgments
The work was financially sponsored by the Ministry of Science and Technology of Taiwan under the projects MOST 109-2221-E-006-139. The authors gratefully acknowledge the use of D8 Discover equipment belonging to the Instrument Center of National Cheng Kung University.
Disclosure statement
The authors declare that they have no known competing financial interests or personal relationships that could have appeared to influence the work reported in this paper.
Additional information
Funding
References
- Zhou D, Li WB, Pang LX, et al. Sintering behavior and dielectric properties of ultra-low temperature fired silver molybdate ceramics. J Am Ceram Soc. 2014 Nov;97(11):3597–3601.
- Dhanya J, Basiluddeen AV, Ratheesh R. Synthesis of ultra low temperature sinterable Na2Zn5(MoO4)(6) ceramics and the effect of microstructure on microwave dielectric properties. Scripta Mater. 2017 Apr 15;132:1–4.
- Zhang GQ, Guo J, Wang H. Ultra-low temperature sintering microwave dielectric ceramics based on Ag2O-MoO3 binary system. J Am Ceram Soc. 2017 Jun;100(6):2604–2611.
- Jiao XQ, Zhong CW, Zhang SR, et al. Microwave dielectric properties of BaO-TiO2-TeO2 ternary system. J Mater Sci. 2010 Jun;45(12):3331–3335.
- Zhang GQ, Guo J, Yuan XF, et al. Ultra-low temperature sintering and microwave dielectric properties of a novel temperature stable Na2Mo2O7-Na0.5Bi0.5MoO4 ceramic. J Eur Ceram Soc. 2018 Feb;38(2):813–816.
- Neelakantan UA, Kalathil SE, Ratheesh R. Structure and microwave dielectric properties of ultralow-temperature cofirable BaV2O6 ceramics. Eur J Inorg Chem. 2015 Jan;(2):305–310. DOI:10.1002/ejic.201402844.
- Kalathil SE, Neelakantan UA, Ratheesh R. Microwave dielectric properties of ultralow-temperature cofirable Ba3V4O13 ceramics. J Am Ceram Soc. 2014 May;97(5):1530–1533.
- Joung MR, Kim JS, Song ME, et al. Formation process and microwave dielectric properties of the R2V2O7 (R=Ba, Sr, and Ca) ceramics. J Am Ceram Soc. 2009 Dec;92(12):3092–3094.
- Suresh EK, Ratheesh R. Structure and microwave dielectric properties of glass free low temperature co-firable SrMV2O7 (M = Mg, Zn) ceramics. J Alloy Compd. 2019 Nov 5;808. DOI: 10.1016/j.jallcom.2019.07.353.
- Umemura R, Ogawa H, Ohsato H, et al. Microwave dielectric properties of low-temperature sintered Mg-3(VO4)(2) ceramic. J Eur Ceram Soc. 2005;25(12):2865–2870.
- Umemura R, Ogawa H, Kan A. Low temperature sintering and microwave dielectric properties of (Mg3-xZnx)(VO4)(2) ceramics. J Eur Ceram Soc. 2006;26(10–11):2063–2068.
- Umemura R, Ogawa H, Yokoi A, et al. Low-temperature sintering-microwave dielectric property relations in Ba-3(VO4)(2) ceramic. J Alloy Compd. 2006 Nov 9;424(1–2):388–393.
- Hakki BW, Coleman PD, Dielectric Resonator A. Method of measuring inductive capacities in the millimeter range. IRE Trans Microw Theory Tech. 1960;8(4):402–410.
- Courtney WE. Analysis and evaluation of a method of measuring the complex permittivity and permeability microwave insulators. IEEE Trans Microw Theory Tech. 1970;18(8):476–485.
- Shannon RD. Dielectric polarizabilities of ions in oxides and fluorides. J Appl Phys. 1993;73(1):348–366.
- Silverman BD. Microwave absorption in cubic strontium titanate. Phys Rev. 1962;125(6):1921–1930.
- Penn SJ, Alford NM, Templeton A, et al. Effect of porosity and grain size on the microwave dielectric properties of sintered alumina. J Am Ceram Soc. 1997;80(7):1885–1888.