ABSTRACT
Laser chemical vapor deposition (LCVD) was applied to prepare SmBa2Cu3O7-δ (SmBCO) superconductive films on single-crystalline LaAlO3 (100) substrates. The effect of oxygen partial pressure (PO2) on the crystal orientation, microstructure, and superconducting property of SmBCO film were investigated thoroughly. The results of X-ray diffraction (XRD) and Raman showed that the crystal orientation of SmBCO film was pure c-axis orientation at the PO2 of 200 Pa, and the a-axis orientation grains gradually emerged in SmBCO film with the increase of PO2. The deposition rate, critical temperature (Tc), critical current density (Jc) firstly increased and then decreased with the increase of PO2. At the PO2 of 200 Pa, the SmBCO film had smooth and dense surface, the lowest FWHM of φ-scan (1.1°), the highest Tc (89.2 K) and Jc (2.04 MA/cm2), which indicated that SmBCO superconductive films possessed excellent crystal structure and superconducting performance.
1. Introduction
The second generation of high-temperature superconducting tape REBCO has been attracted much attention in recent years, which has become a hot topic in present research, such as SmBa2Cu3O7−δ (SmBCO) [Citation1,Citation2]. SmBCO superconductor has been applied in different fields (e.g. cable transmission, motor, generator, magnetic energy storage system), which was due to its high critical temperature (Tc) and critical current density (Jc) [Citation3,Citation4]. The common physical deposition method of SmBCO film, such as pulsed laser deposition (PLD) [Citation5–7], was not suitable for the large-scale commercial application, because of its high vacuum degree requirement and low deposition rate. Compared with PLD, metal organic chemical vapor deposition (MOCVD) is more adapted to industrial production of thin films. The vacuum degree requirement of MOCVD is not high, which is beneficial to reduce the cost of equipment. Moreover, the preparation process is simple and the preparation parameters are easier to control [Citation8–10]. Therefore, the preparation of SmBCO film by MOCVD has a better application prospect. However, the deposition rate of traditional MOCVD method still could not satisfy the large-scale and commercial preparation of SmBCO films. In order to increase the deposition rate, laser has been introduced into the chemical vapor deposition [Citation11–14]. Laser chemical vapor deposition (LCVD) can remarkably improve chemical reaction of precursors on substrates, which is conducive to enhance the deposition rate of film.
In addition, except for the deposition rate, the excellent crystal structure, and superconducting performance are also necessary for the commercial application of SmBCO film. The main impacts of the structure and performance of SmBCO film are deposition temperature, deposition time, oxygen partial pressure and so on. The oxygen partial pressure is particularly important, which directly determines the pure c-axis orientation structure and oxygen content of SmBCO film [Citation15,Citation16]. In recent years, some researches showed that the suitable oxygen partial pressure contributed to optimize the structure of SmBCO film and improve the superconducting properties [Citation17,Citation18]. Jin et al. found that the low oxygen partial pressure was required to successfully obtain GdBCO films without impurity phases and a-axis-oriented grains [Citation17]. Li et al. discovered that the morphology, growth rate, and degree of texture formation of YBCO films were affected obviously by oxygen partial pressure deposited by photo-assisted MOCVD [Citation19]. Hence, it is necessary to investigate the effect of the oxygen partial pressure on the structure and superconducting performance of SmBCO films by LCVD, which is beneficial to accelerate the commercial application of SmBCO film.
For this purpose, in this paper, SmBCO film was prepared on single-crystalline LAO (100) substrates by LCVD. The effect of oxygen partial pressure on the phase composition, crystal orientation, microstructure, and critical current density of SmBCO superconducting films were thoroughly investigated.
2. Materials and methods
The vertical cold-wall laser chemical vapor deposition system (LCVD) was applied to prepare SmBCO films. The laser adopted in LCVD was a kind of semiconductor laser, which had continuous wave (wavelength: 808 nm). The substrate was single crystalline LaAlO3 (LAO) substrates (10 × 5 × 0.5 mm). The precursors were Sm(dpm)3, Ba(dpm)2 and Cu(dpm)2 (dpm: 2,2,6,6-tetramethyl-3,5-heptanedionato, Toshima Manufacturing Co. Ltd., JP). The precursors were placed in independent vessels and heated in vacuum. The vaporizing temperatures of Sm(dpm)3, Ba(dpm)2 and Cu(dpm)2 were set to 192, 300, and 168°C, respectively. The precursor gases were transported by Ar gas. In order to prevent the precursor gases condensing in the pipelines, the gas pipelines and nozzle were generally heated to higher than 350°C. The precursor gases and O2 gas were mixed before entering the nozzle. The substrates were placed directly below the vessel and heated by the laser with beam diameter of 15 mm. When the substrate reached 780°C, opening the nozzle to let the reaction gases enter the reaction chamber. The total pressure (Ptot) of the reaction chamber was fixed at 500 Pa. The flow rate of Ar gas (FAr) in each line (3 line) was fixed to 100 standard cubic centimeter per minute (sccm). The flow rate of O2 (FO2) was 100, 200, 300, and 450 sccm, the corresponding oxygen partial pressure (PO2) are shown in . The film growth time was set as 10 min. After the deposition, the SmBCO films were heat-treated at laser power of 30 W for 1 h in pure O2 gas at an atmosphere pressure.
Table 1. The oxygen partial pressure (PO2) of SmBCO films deposited by LCVD
X-ray diffraction (XRD; Ultima-Ш, Rigaku, JP) was used to measure the crystalline phases of the SmBCO films with Cu-Kα radiation. The in-plane orientation of films was estimated by φ-scan on the (116) reflection. The Raman scattering spectra were measured in a quasi-backscattering geometry at the wavelength of 633 nm with an Ar ion laser (INVIA, Ger). The surface and cross-sectional micromorphology of the films was characterized by field emission scanning electron microscopy (SEM; FEI Quanta-250, USA). Atomic force microscopy (AFM; Innova, Bruker, USA) working at the tapping mode was used to characterize the surface and to measure the roughness of the films. The superconducting critical temperatures (Tc) of the SmBCO films were tested by standard four-point-probe at the temperature range of 10–300 K by using a physical properties measurement system (PPMS 9; Quantum Design, USA). The critical current (Ic) were obtained at 77 K and self-field, and the voltage criterion was 1 μV/cm [Citation20]. The Jc was calculated from the ratio of critical current (Ic) to the film thickness.
3. Results and discussion
The XRD patterns of SmBCO films deposited on LAO substrate by LCVD at varied PO2 were portrayed in . It could be observed that only c-axis oriented peaks (00 l) of SmBCO film peaks appeared in , which was corresponding to the PO2 of 125 Pa and 200 Pa, respectively. The intensity of c-axis oriented peaks of the SmBCO film enhanced with the increasing of PO2. Furthermore, it was noteworthy that the c-axis-oriented peaks intensity of the SmBCO film was the highest at the PO2 of 200 Pa, which indicated that the c-axis preferred orientation of SmBCO film was strongest at this PO2. When the PO2 exceeded 200 Pa (), a-axis-oriented peaks (h00) appeared in the XRD patterns of SmBCO film, and the intensity of a-axis-oriented peaks was gradually increased with the PO2 increasing. The results indicated that the SmBCO film transitioned from pure c-axis to a- and c-axis coorientation with the increase of PO2, moreover, the proportion of a-axis orientation particles increased.
Figure 1. XRD patterns of SmBCO films deposited on LAO substrate with PO2 at (a) 125, (b) 200, (c) 250 and (d) 300 Pa, (e) the crystal structure of SmBCO
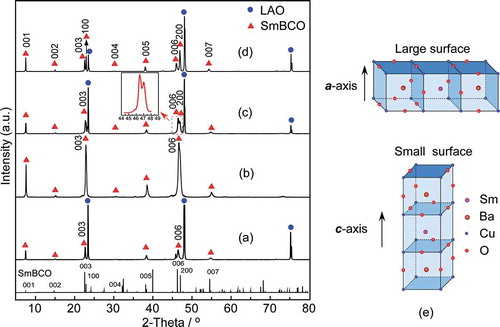
The orientation change of SmBCO film was related to the crystal growth mode [Citation21]. The crystal structure of SmBCO was a kind of defect perovskite structure as shown in ). The length of a-axis was similar to that of b-axis, and the length of c-axis was about 3 times the length of a-axis, which resulted in the growth orientation of SmBCO film transforming with the change of PO2. When the oxygen partial pressure was low, it was beneficial to the nucleation of SmBCO grains along the vertical direction of small surface area, namely the c-axis direction. When the oxygen partial pressure was relatively high, it would promote the nucleation of SmBCO grains along the vertical direction of large surface area (the a-axis direction). Hence, it could be concluded that the low PO2 was conducive to the growth of SmBCO grains along the c-axis direction and restrained the growth along the a-axis direction, especially at the PO2 of 200 Pa, the c-axis preferred orientation of SmBCO grains was the strongest.
exhibits XRD φ-scan for (116) reflection of SmBCO film deposited at PO2 of 125–300 Pa and the effect of PO2 on the full width at half maximum (FWHM) of φ-scan. At the PO2 of 125 and 200 Pa ()), there were four strong peaks appearing in the φ-scan for (116) reflection of SmBCO film, the interval between adjacent peak was 90°. The four peaks were attributed to c-axis-oriented SmBCO grain. Compare with the PO2 of 125 and 200 Pa, it was obviously observed that extra eight lower peaks appeared in the φ-scan for (116) reflection of SmBCO film at the PO2 of 250 and 300 Pa (). The eight peaks were divided into two groups, each group had four peaks and the interval between adjacent peaks was 90°, each group of peaks represented the a-axis orientation in the SmBCO film. The eight peaks indicated that twinning structure of a-axis orientation appeared in SmBCO films.
Figure 2. φ-scan for (116) reflection of SmBCO films with PO2 at (a) 125, (b) 200, (c) 250 and (d) 300 Pa, (χc = 54° for c-axis orientation, χa = 24° for a-axis), (e) FWHM of φ-scan for (116) reflection of SmBCO with PO2 at 125–300 Pa
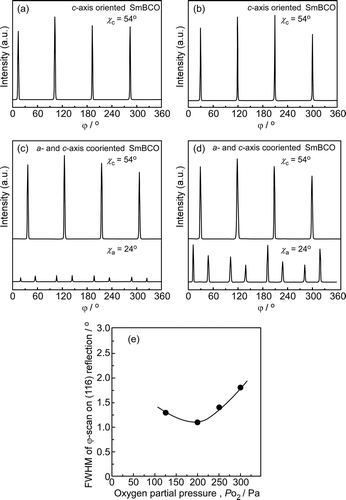
FWHM of φ-scan was utilized to evaluate the in-plane orientation consistency of SmBCO film, the lower the FWHM value, the better the in-plane orientation consistency of SmBCO film, that was, the better the crystallization quality of SmBCO film. The φ-scan on the (116) reflection was measured in all SmBCO films, and the FWHM of φ-scan was the FWHM of average value of FWHM of four c-axis oriented peaks. In ), it could observe the FWHM of φ-scan of SmBCO film (116) reflection decreased firstly and then increased with the increase of PO2. The FWHM value was the minimum (1.1°) at the PO2 of 200 Pa, which implied that the grain-to-grain ordered alignment was relatively excellent. At high PO2 (250 and 300 Pa), the FWHM value increased to 1.4° and 1.8°, which was due to the appearance of the a-axis coorientation in SmBCO films. Although the length of c-axis was similar to three times the length of a-axis, there was 1.4% lattice mismatch between them (according to JCPDS#89-8900). The lattice mismatch would generate a large number of boundaries, which led to the poor in-plane arrangement of the grains in the film. The formation of a-axis-orientated grains deteriorated the in-plane alignment of SmBCO film, resulting in that the FWHM of φ-scan for (116) reflection SmBCO film was higher at PO2 of 250 and 300 Pa.
displays the Raman scattering spectra of SmBCO film deposited at the PO2 of 125–300 Pa. The peak around at 140 cm−1 was the stretching modes of Cu2-Cu2 in the CuO2 plane. The peak of 220 cm−1 was broken-chain mode or oxygen disorder, which was related to the imperfect grain connectivity and instability of original phases. The peaks located at 317 cm−1 and 445 cm−1 were the out-of-phase bending mode of O(2)+/O(3)- and the in-of-phase bending mode of O(2)+/O(3)+, respectively [Citation22]. The peak intensity of O(2)+/O(3)- should be maximum if the films had a c-axis orientation in an orthorhombic phase [Citation23,Citation24]. The peak placed at 500 cm−1 was apical O(4) mode, which was connected with the oxygen stoichiometry. The intensity relative ratio of the O(2)+/O(3)- peak and O(4) peak in c-axis-oriented REBCO film was regarded as an indication of tilted grains and a-axis grains [Citation25]. In ), a broad peak around 530 cm−1 was detected at PO2 of 125 Pa. There was no enough oxygen for the chemistry reaction during the SmBCO film deposition process, the peak of the apical O(4) mode (the peak around 500 cm−1) moved to the right [Citation26]. The O(2)+/O(3)- peak at around 317 cm−1 was higher than that of 500 cm−1 ()), indicating the orientation of SmBCO film was mainly c-axis at PO2 of 200 Pa, and the peaks located at 220 cm−1 were extremely weak, which manifested that the SmBCO film deposited at the PO2 of 200 Pa had excellent crystal structure. At the PO2 of 250 and 300 Pa (), it could be observed that the peak at 500 cm−1 enhanced obviously, which implied that the a-axis-oriented and tilted SmBCO grains formed easily with the increasing PO2. Furthermore, at PO2 of 300 Pa, there was stronger peak at 220 cm−1, which was due to the poor crystallinity.
Figure 3. Raman scattering spectra of SmBCO films prepared at different PO2 of (a) 125, (b) 200, (c) 250 and (d) 300 Pa
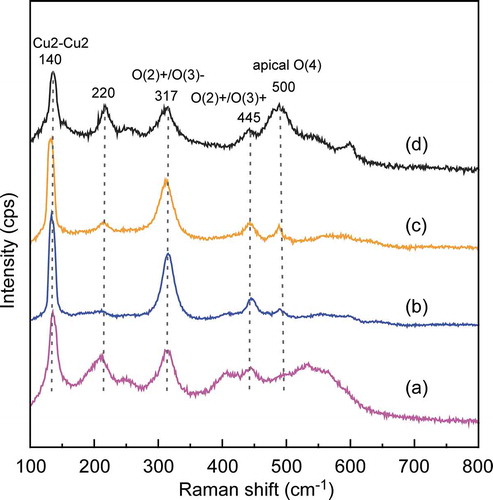
The surface morphologies of SmBCO films prepared at different PO2 were displayed in . It could be seen that SmBCO film deposited at PO2 of 125 Pa had rough surface with random arranged grains ()), the irregular grain growth produced large-angle grain boundaries and pores, which resulted in the poor crystallization quality of SmBCO films. The low PO2 was not conducive to the orientation deposition of SmBCO film. When the PO2 increased to 200 Pa, the surface morphology of SmBCO film became smooth and dense ()), indicating that the SmBCO deposited at PO2 of 200 Pa had relatively excellent orientation and less impure phase. At PO2 of 250 Pa ()), it could be seen obviously that needlelike grains on a flat surface appeared in the SmBCO film, the needlelike grains was due to the a-axis-oriented growth of SmBCO [Citation27]. Moreover, when the PO2 increased to 300 Pa ()), the quantity and size of needlelike grains noteworthy increased. Under the condition of high PO2, the deposition rate of SmBCO films along the <100> and <010> directions (a-axis-oriented SmBCO grains) was faster than that along the <001> direction (c-axis oriented SmBCO films), which led to a lot of needlelike grains on SmBCO films [Citation28]. The results of surface morphologies of SmBCO films were consistent with the change of orientation at varied PO2.
Figure 4. Surface SEM images of SmBCO films prepared on LAO substrate with PO2 of (a) 125, (b) 200, (c) 250 and (d) 300 Pa
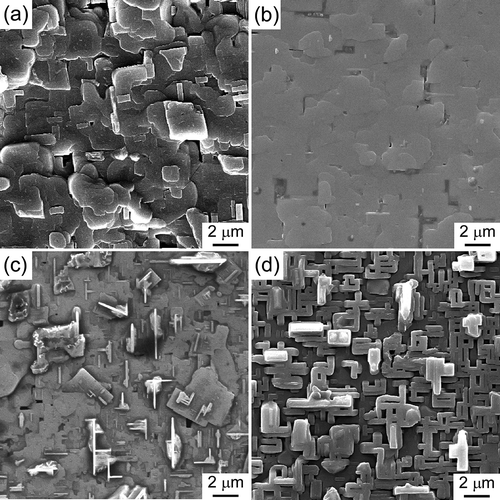
exhibits the three-dimensional AFM images of 5 × 5 μm area of the SmBCO films prepared at different PO2. It could be obviously observed that the surface roughness (Ra) of SmBCO films changed remarkably with the PO2 increase. At the PO2 of 125 Pa, the Ra of SmBCO films was 124 nm, when the PO2 increased to 200 Pa, the Ra of SmBCO films decreased to 44.6 nm, which indicated that the surface roughness of the films became smoother with the PO2 increase. However, when the PO2 exceeded 200 pa, the Ra of SmBCO films rapidly increased, and the Ra of SmBCO films at 250 pa and 300 pa were 64.2 nm and 160 nm, respectively, which was due to the a-axis-oriented grains growing faster on the surface of SmBCO films than that of c-axis oriented grains at higher PO2. The result showed that SmBCO films deposited at the PO2 of 200 pa possessed the smooth surface and excellent orientation.
Figure 5. AFM images of SmBCO films prepared at different PO2 of (a) 125, (b) 200, (c) 250 and (d) 300 Pa
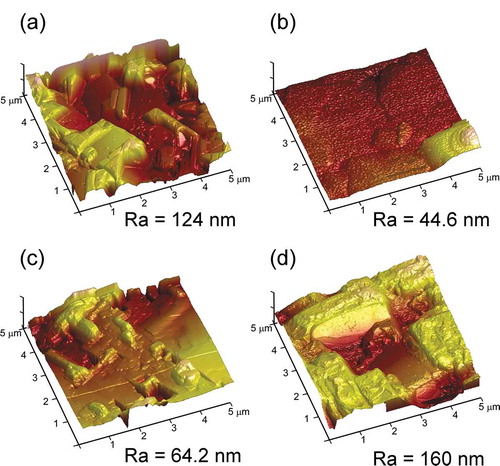
The cross-sectional images of SmBCO films and the deposition rate dependence on PO2 were displayed in . All of the cross-sections of SmBCO films were dense without gap between the film and substrate, indicating that LCVD was appropriate for preparing SmBCO film on LAO substrates. Noticeably, the upper edge of the cross-sections of the SmBCO film deposited at the PO2 of 200 Pa ()) was more smooth, some protrusions could be observed on the cross-sections of the SmBCO film at other PO2 (), especially the PO2 of 300 Pa, the appearance of protrusions on the cross section of SmBCO film were due to the faster-growing a-axis-oriented particles [Citation29].
Figure 6. Cross-sectional SEM images of SmBCO films prepared on LAO substrate with PO2 of (a) 125, (b) 200, (c) 250 and (d) 300 Pa, (e) the comparison of Rdep of the REBCO films deposited by LCVD and other CVD (PA-CVD: photo-assistant CVD)
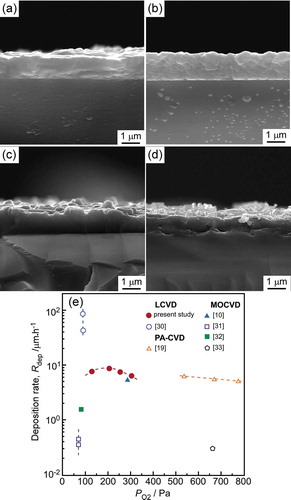
The deposition rate of SmBCO film was the ratio of film thickness and the corresponding deposition time, and the deposition rates at different PO2 are shown in ). It could be seen that the deposition rate of SmBCO film was increased with the Po2 increasing at low PO2 (ranging from 125 to 200 Pa). The deficiency of oxygen led to the slow deposition rate at low PO2, and this situation was noticeably alleviated with the PO2 increasing, the deposition rate reached the maximum (8.76 μm·h−1) at the PO2 of 200 Pa. However, when the PO2 exceeded 200 Pa, the deposition rate decreased with the increasing of PO2. The higher PO2 resulted in the oxygen content increase in the reaction chamber, which would surely decrease the other precursor gases content at the constant total pressure (500 Pa). The imbalance stoichiometric ratio of oxygen and precursor ultimately induced the slow deposition rate and the appearance of impure phase. In addition, in ), it could observed that the Rdep of the REBCO films deposited by LCVD was obviously higher than those deposited in other CVD [Citation10,Citation19,Citation30–33]. In the CVD process, high O2 and precursors supply were essential to the high Rdep. However, in the thermal CVD, excess O2 and precursors supply would lead to the premature reaction in the gas phase above the substrate and insufficient reaction on the substrate, which would cause the decline of Rdep and the formation of a second phase and degraded orientation [Citation34,Citation35]. Compared with the thermal CVD, the Rdep of REBCO film deposited by LCVD was high at low PO2, which was due to the laser irradiation improving the decomposition of O2 and activating the chemical reaction in the gas phase, giving rise to significantly high Rdep even under low PO2. On the other hand, the heating mode of LCVD (surface heating) could avoid the temperature difference in the thickness direction, which was beneficial to improve the orientation consistency [Citation36].
The electrical resistivity to temperature (R-T) curve of the SmBCO film deposited at the PO2 of 200 Pa and the effect of PO2 on the Tc were exhibited in . It could be observed that the electrical resistivity of the SmBCO films reduced with the decrease of temperature above 89.2 K ()), that was, the SmBCO films showed strong metallic temperature dependence above 89.2 K. When the temperature was below 89.2 K, the electrical resistivity of the SmBCO films reduced sharply to zero, which indicated the critical temperature (Tc) value of the SmBCO films deposited at the PO2 of 200 Pa was 89.2 K. As showed in ), the Tc of SmBCO film increased from 86.5 to 89.2 K with the increasing of PO2 from 125 to 200 Pa. When the PO2 was over 200 Pa, the Tc of SmBCO film decreased with the increasing of PO2, the Tc dropped to 79.6 K at the PO2 of 300 Pa. Notably, the Tc of SmBCO film was the highest at the PO2 of 200 Pa, which manifested that the superconducting performance of SmBCO film was relatively easy to achieve at the PO2 of 200 Pa.
Figure 7. (a) Temperature dependence of electrical resistance of the SmBCO films deposited at PO2 of 200 Pa, (b) the critical temperature (Tc) of the SmBCO film as a function of PO2.
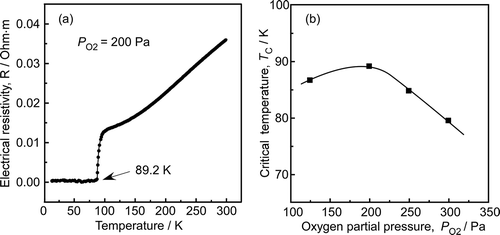
The critical current density (Jc) was utilized to evaluate the current carrying capacity of superconductive film. The higher the Jc, the better the current-carrying capacity. The Jc could be calculated from the ratio of critical current (Ic) to the thickness. exhibits the current-voltage (I–V) curve (@77 K, self-field) for SmBCO films deposited at PO2 of 200 Pa and the Jc of the SmBCO films dependence on the PO2. The SmBCO film deposited at the PO2 of 200 Pa was superconducting state at 77 K (as shown in ), the voltage of SmBCO film should be zero with the increasing of current under this situation. However, in ), it could be observed that the voltage of SmBCO film increased rapidly when the current exceeded a certain value, which indicated that the superconducting performance of SmBCO film was lost. The voltage reaching 1 μV/cm was considered as the criterion of the critical superconducting performance, and the corresponding current was Ic. The Ic of SmBCO film deposited at the PO2 of 200 Pa was 297 A/cm-w at 77 K and self-field. In the ), the Jc of the SmBCO films gradually increased with the variation of PO2 (from 100 to 200 Pa), and the Jc was the maximum (2.04 MA/cm2) at the PO2 of 200 Pa. It indicated that the increase of PO2 was beneficial to improve the current carrying capacity of the SmBCO films. However, when the PO2 was more than 200 Pa, the Jc decreased with the increase of PO2, which was due to the appearance of a-axis-oriented grains in the SmBCO films at higher PO2. The transmission of electric current in SmBCO film was on the Cu-O plane, which was parallel to ab-plane of c-axis-oriented SmBCO film. Hence, the appearance of the a-axis-oriented grains would obstruct the transmission of electric current [Citation37,Citation38]. The results showed that the SmBCO films deposited at the PO2 of 200 Pa had the best superconducting performance.
4. Conclusions
SmBCO films were deposited by LCVD on LAO (100) substrates at PO2 of 125–300 Pa. The effect of PO2 on the orientation, crystallinity and superconductivity performance of SmBCO film was investigated. The following conclusions can be drawn from the results:
(1) The SmBCO films gradually transformed from c-axis to a- and c-axis coorientation with the increasing of PO2, and the appearance of a-axis-oriented grains in SmBCO films led to the poor in-plane arrangement in the film. The SmBCO films deposited at the PO2 of 200 Pa had the strongest c-axis preferred orientation and excellent in-plane arrangement (the FWHM value of 1.1°).
(2) The deposition rate of SmBCO films first increased and then decreased with the increasing of PO2, which was due to the imbalance stoichiometric ratio of oxygen and precursor at high PO2. The reaction system possessed the optimal stoichiometric ratio of reaction system at the PO2 of 200 Pa, whose deposition rate reached the maximum (8.76 μm·h−1).
(3) At the PO2 of 200 Pa, the SmBCO film had the highest Tc (89.2 K) and Jc (2.04 MA/cm2), which indicated that the SmBCO superconductive films deposited at the PO2 of 200 Pa exhibited the best superconducting performance.
Acknowledgments
This work was supported by National Natural Science Foundation of China (52002075), State Key Laboratory of Advanced Technology for Materials Synthesis and Processing (Wuhan University of Technology: 2019-KF-12), Talent Introduction Fund Project of Fuzhou University (XRC-18090) and Fuzhou University Testing Fund of Precious Apparatus (2019T029). The authors gratefully acknowledge their financial support. Thanks are also due to Testing Center of Fuzhou University for the experienced tests.
Disclosure statement
No potential conflict of interest was reported by the authors.
Additional information
Funding
References
- Senatore C, Alessandrini M, Lucarelli A, et al. Progresses and challenges in the development of high-field solenoidal magnets based on RE123 coated conductors. Supercond Sci Technol. 2014;27(10):103001.
- Knibbe R, Strickland NM, Wimbush SC, et al. Structure property relationships in a nanoparticle-free SmBCO coated conductor. Supercond Sci Technol. 2016;29(6):0066006.
- Shiohara Y, Taneda T, Yoshizumi M. Overview of materials and power applications of coated conductors project. Jpn J Appl Phys. 2012;51(1):010007.
- Obradors X, Puig T. Coated conductors for power applications: materials challenges. Supercond Sci Technol. 2014;7(4):044003.
- Yoshida Y, Ozaki T, Ichino Y, et al. Progress in development of advanced PLD process for high Jc REBCO film. Phys C. 2008;468(15–20):1606–1610.
- Tsuruta A, Yoshida Y, Ichino Y, et al. Flux pinning properties at low temperatures in BaHfO3 doped SmBa2Cu3Oy films. IEEE Trans Appl Supercond. 2013;23(3):8001104.
- Miura S, Yoshida Y, Ichino Y, et al. Flux pinning properties of a SmBa2Cu3Oy film including high number density of BaHfO3 nano-rods on LaAlO3 substrate. J Supercond Nov Magn. 2015;28(2):367–369.
- Wang T, Zhang S, Yang M, et al. Effect of precursors’ ratio on c-axis-oriented SmBCO film by MOCVD. Ceram Int. 2017;43(1):S488–S492.
- Wang T, Tu R, Ke W, et al. Morphology study of oriented SmBCO film deposited by MOCVD. J Wuhan Univ Technol. 2016;31(1):15–19.
- Xiong J, Zhang F, Liu X, et al. Epitaxial growth of MOCVD-derived YBCO films by modulation of Cu(tmhd)2 concentration. Rare Met. 2014;33(1):70–74.
- Zhu P, Xu Q, Chen R, et al. Structural study of β-SiC (001) films on Si (001) by laser chemical vapor deposition. J Amer Ceram Soc. 2017;100(4):1634–1641.
- Cheng H, Tu R, Zhang S, et al. Preparation of highly oriented β-SiC bulks by halide laser chemical vapor deposition. J Eur Ceram Soc. 2017;37(2):509–517.
- Zhao P, Ito A, Tu R, et al. High-speed growth of YBa2Cu3O7−δ film with high critical temperature on MgO single crystal substrate by laser chemical vapor deposition. Supercond Sci Technol. 2010;23(12):125010.
- Zhang S, Tu R, Goto T. High-speed epitaxial growth of β-SiC film on Si (111) single crystal by laser chemical vapor deposition. J Amer Ceram Soc. 2012;95(9):2782–2784.
- Yurtcan MT, Simsek O, Yilmaz M, et al. Influence of deposition pressure (O2) on the YBCO (Y123) thin films prepared by pulsed laser deposition. J Supercond Nov Magn. 2013;26(5):1873–1877.
- Ichino Y, Yoshida Y, Takai Y, et al. Influences of oxygen pressure and substrate temperature on the quality of NdBa2Cu3Ox thin films prepared by pulsed laser deposition. Supercond Sci Technol. 2004;17(6):775–780.
- Jin L, Lu Y, Yan W, et al. Fabrication of GdBa2Cu3O7-x films by TFA-MOD process. J Alloys Compd. 2011;509(7):3353–3356.
- Geo-Myung S, Kim D, Song K, et al. MOD-processed SmBCO films on LaAlO3(100) substrates. Trans Appl Supercond. 2007;17(2):3561–3563.
- Li W, Li G, Zhang B, et al. Fabrication of GdBa2Cu3O7-δ films by photo-assisted-MOCVD process. Phys C. 2014;501:1–6.
- Matsuno S, Uchikawa F, Utsunomiya S, et al. Metalorganic chemical vapor deposition using a single solution source for high Jc Y1Ba2Cu3O7-x superconducting films. Appl Phys Lett. 1992;60(19):2427–2429.
- Araki T, Niwa T, Yamada Y, et al. Growth model and the effect of CuO nanocrystallites on the properties of chemically derived epitaxial thin films of YBa2Cu3O7-x. J Appl Phys. 2002;92(6):3318–3325.
- Jee YA, Ma B, Maroni VA, et al. Texture development and superconducting properties of YBa2Cu3Ox thin films prepared by a solution process in low oxygen partial pressure. Supercond Sci Technol. 2001;14(5):285–291.
- Kim G, Jo W, Ha H, et al. Characterization of co-evaporated coated conductors by polarized-Raman scattering spectroscopy. IEEE Trans Appl Supercond. 2011;21(3):3352–3355.
- Venkataraman K, Baurceanu R, Maroni V. Characterization of MBa2Cu3O7-x thin films by Raman microspectroscopy. Appl Spectrosc. 2005;59(5):639–649.
- Fainstein A, Etchegoin P, Guimpel J. Raman study of photoinduced chain-fragment ordering in GdBa2Cu3Ox thin films. Phys Rev B. 1998;58(14):9433–9439.
- Hong S, Kim K, Cheong H, et al. Raman analysis of asymmetrical chains in YBa2Cu3O7-δ films. Phys C. 2007;454(1–2):82–87.
- Li S, Li W, Li G, et al. Morphology and superconducting properties of photo-assisted MOCVD processed YBCO film by variation of sublimation temperature of the Cu-based precursor. Phys C. 2012;478:60–66.
- Granozio F M, Sctti Di Uccio U. Model for the nucleation of (001) and (100) oriented grains in YBCO films. J Cryst Growth. 1997;174(1–4):409–416.
- Zhao P, Ito A, Goto T. Effect of deposition temperature on the orientation and electrical properties of YBa2Cu3O7-δ films prepared by laser CVD using liquid-source evaporation. Ceram Int. 2014;40(1):2057–2061.
- Zhao P, Ito A, Kato T, et al. High-speed growth of YBa2Cu3O7-δ superconducting films on multilayer-coated Hastelloy C276 tape by laser-assisted MOCVD. Supercond Sci Technol. 2013;26(5):055020.
- Sujiono E, Arifin P, Barmawi M. YBa2Cu3O7-δ thin films deposited by a vertical MOCVD reactor. Mater Chem Phys. 2002;73:47–50.
- Abrutis A, Bartasyte A, Saltyte Z, et al. Thick SmBCO layers and SmBCO/YBCO structures grown by pulsed injection MOCVD. Phys C. 2004;415(1–2):21–28.
- Ma Y, Watanabe K, Awaji S, et al. New Metalorganic chemical vapor deposition process in a high magnetic field for YBa2Cu3O7. J Appl Phys. 2000;35(7B):L726–L729.
- Zhao P, Ito A, Goto T. Rapid deposition of YBCO films by laser CVD and effect of lattice mismatch on their epitaxial growth and critical temperature. Ceram Int. 2013;39(7):7491–7497.
- Kanehori K, Sughii N, Fukazawa T, et al. Low temperature growth of superconducting YBa2Cu3O7-x thin films by organometallic chemical vapour deposition. Thin Solid Films. 1989;182(1–2):265–269.
- Wang T, Wang K, Tu R, et al. Thickness dependence of structure and superconductivity of the SmBa2Cu3O7 film by laser CVD. RSC Adv. 2017;7(89):56166–56172.
- Sieve S, Mattheis F, Krebs HU, et al. Grain orientation in thick laser-deposited of c-axis orientation Y1Ba2Cu3O7-δ films: adjustment of c-axis orientation. Am Inst Phys. 1995;78(9):5545–5548.
- Ibi A, Iwai H, Takahashi K, et al. Investigations of thick YBCO coated conductor with high critical current using IBAD-PLD method. Phys C. 2005;43:910–914.