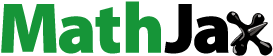
ABSTRACT
Cordierite-based ceramic is one of the most interesting engineering materials that has attractive and multidisciplinary assets, such as low thermal expansion coefficient, excellent thermal shock resistance, high refractoriness, good mechanical properties, and so on. In this work, cordierite-based ceramics were prepared mainly from stevensite-rich clay or coal fly ash (CFA) and stevensite-rich clay using a solid-state interaction process. The influence of heating temperature, in the range of 800–1250°C, and starting materials on the microstructure-temperature evolutions and mechanical strength were evaluated using TGA-DTA, XRD, FTIR, SEM, and dilatometric analysis. The results revealed, for both formulations heating in the temperature range 800–1100°C, the transformation of stevensite-rich clay into enstatite and its polymorphisms. Further heating induced the formation of the cordierite phase at 1200°C, for the formulation consisted mainly of stevensite-rich clay and CFA, and at 1250°C for that contained mainly stevensite-rich clay. The addition of CFA to stevensite-rich clay enhanced its reactivity and involved the increase in mechanical strength from 5 MPa to 15 MPa at 1200°C.
1. Introduction
In the last few years, cordierite-based ceramics have gained a lot of interest for many applications as insulator materials, catalyst supports, electronic substrates, and refractory materials, because of its very low expansion coefficient (1.5–3.10−6°C−1), high mechanical strength, good thermal and chemical stability, and so on [Citation1–5]. However, cordierite ceramic with high purity is difficult to obtain due to its narrow sintering temperature range as well as an incomplete transformation of starting material into cordierite phase with the sintering temperature below 1430°C [Citation6–8]. Therefore, many attempts have been made to prepare high-purity cordierite at low temperature testing tremendous starting materials and synthetic routes.
Several sophisticated methods have been attempted to prepare cordierite with high purity at low temperatures as it is not pure and is considered a scarce mineral. Some of these methods such as combustion, solid-state, and co-precipitation as well as sol-gel have been frequently employed [Citation9–13]. The sol-gel method provided commonly amorphous or poorly crystallized cordierite precursors, which require a pre-calcination above 600°C before being sintered at temperatures above 1300°C. In addition, this synthesis route is complex to proceed and requires expensive synthetic starting materials such as organometallic silicon, aluminum, and magnesium. Instead, solid-state reaction remains an effective method for industrial manufacturing of cordierite-based ceramics with high purity starting from low-cost materials such as abundant resources and industrial by-products rich in silica and alumina. Thus, the precursor interactions at high temperatures are strongly influenced by the nature of the starting materials and the processing parameter such as temperature, compaction, and particle size distribution. These factors affect the mechanism of crystallization and sintering temperature of cordierite as well as its purity [Citation14,Citation15].
Many abundant resources of alumina and silica precursors, such as kaolin, clay, andalusite, talc, and quartz have been used to prepare cordierite-based ceramic materials with high purity and attractive properties. While the use of magnesia resources for cordierite preparation was limited as it is less abundant [Citation16–18]. Some starting materials rich in magnesia such as talc, and stevensite-rich clay [Citation19–21] have been tested to prepare cordierite-based ceramics with high purity and attractive technological assets for tremendous applications. Recently, we have used an abundant Moroccan material named ghassoul as a new magnesia source, which is considered stevensite-rich clay and the only deposit in the world. We have found that the use of ghassoul, as a magnesia silica source for the manufacturing of porous membrane, involved the appearance of cordierite at a low temperature of 1000°C [Citation22,Citation23]. However, other research teams found the use of ghassoul and andalusite involved high sintering temperature up to 1350°C to obtain cordierite-based ceramics [Citation7]. This can be due to the low reactivity of the andalusite phase. In our other previous work, we studied the temperature-microstructure evolutions of ghassoul clay. We found that the increase in the sintering temperature from 1000°C to 1200°C induced the transformation of stevensite into the clinoenstatite phase, which involved the deterioration of the mechanical behaviors. These findings suggest the need for further investigation on the use of ghassoul clay with other highly reactive starting materials such as CFA to obtain cordierite-based ceramics at low temperatures with high purity. Recently, we found that CFA can be useful material to prepare cordierite-based ceramics at a low temperature 1200°C with high purity and high compressive strength reaching 128 MPa [Citation24–26].
This work aims to prepare cordierite-based ceramic at low temperature starting from Moroccan naturally occurring stevensite-rich clay and CFA. For this reason, the phase transformations and mechanical strength of the heated samples in the range of 1000–1200°C were investigated in detail.
2. Materials and methods
2.1. Samples preparation
Stevensite-rich clay called Ghassoul comes from the only deposit in the world, located at the east side of the middle atlas mountains, in the Moulouya valley, approximately 200 km away from Fes city, Morocco. CFA was procured from Jorf Lasfar power station, Morocco. The chemical compositions of the stevensite-rich clay and CFA are given in . Stevensite-rich clay was composed of silicon oxide (54 wt.%) and magnesium oxide (21 wt.%) while CFA consisted of silica (48.84 wt.%) and alumina (32.79 wt.%). The structural phases of both starting materials were given in our previous works [Citation26,Citation27]. The main phases of ghassoul clay were stevensite, and dolomite, while CFA mainly contained mullite and quartz phases. The loss on ignition (LOI) of Ghassoul was around 15 wt.%, which is mainly associated with organic matter. For CFA, the LOI was less than 5 wt.%, which is related to unburned carbon.
Table 1. Chemical composition of Ghassoul and fly ash (wt. %).
The particle size distribution of both materials has a bimodal distribution with a modal size located at around 0.3 µm and 50 μm and volume amount at around 30% and 70%, respectively. This particle size distribution of both starting materials can be useful for powder compacting under uniaxial pressure. Two formulations were prepared mainly from these materials according to the stoichiometric composition of the cordierite phase by solid-state reaction (). The first, denominated formulation A, consisted of stevensite-rich clay, CFA, and aluminum hydroxide. The second, formulation B, consisted mainly of stevensite-rich clay, silica gel, and aluminum hydroxide. The resulting samples were heated in the temperature range 800–1250°C using a heating rate of 10°C/min. For the mechanical testing, parallelepipedal specimens of size (1 cm*1 cm*4 cm) were prepared by using rhodoviol as an organic binder and uniaxial compression of 15 MBar. The prepared specimens were dried at 110°C for 48 h and then sintered at 1000, 1100, and 1200°C for 2 h with a heating rate of 1°C/min.
Table 2. Batch composition of the prepared samples.
2.2. Experimental techniques
The characterization of the prepared samples was carried out using several experimental techniques. The chemical composition in terms of mineral oxide was performed using a Philips PW 1666 X-ray fluorescence spectrometer. The granulometric distribution was determined in an aqueous medium using HORIBA LA-300. The X-ray diffraction analysis of the prepared powder was conducted by Philips PW diffractometer type X’Pert MPD, with copper anticathode (λKα = 1.5406 Å). The structural identification of the crystalline phases as well as their chemical formula was determined using Highscore software. The analysis by infrared spectroscopy was performed using a Perkin Elmer Spectrum, with a resolution of 4 cm−1 in the wavenumber range of [4000–400 cm−1]. A dilution of the sample with 1 wt.% of KBr was performed. Thermogravimetric (TGA) and thermal differential (DTA) analyzes were performed with an apparatus type Versa Therm thermal, under nitrogen atmosphere with a heating rate of 10°C/min. The dilatometric measurements were carried out using a SETARAM apparatus, with a heating rate of 1°C/min from room temperature up to 1000°C. A Vega 3 Tescan scanning electron microscope was employed for imaging analysis on the sintered specimens at 1250◦C. The three-point bending test was carried out at room temperature on parallelepipedic specimens using an Instron 3369 instrument with a cross-head speed of 1 mm/min.
3. Result and discussion
3.1. Characterization of raw formulations
Particle size analysis of the prepared formulations is shown in . From the trend of both formulations, the particle distribution followed a bimodal distribution of a modal size (d50) with two maximum points. In the range [0.1–1], both formulations show the presence of small particle size with a modal size (d50) located at around 0.39 μm. While, in the range of 2–200 µm, the large particle size was centered at 56 and 25 μm for formulations A and B, respectively. The difference in particle size of both formulations can be due to the nature of starting materials, while formulation A mainly consisted of CFA, which is known by its small particle size. However, formulation B, which contained mainly stevensite-rich clay, tends to agglomerate into a large-size grain.
The XRD patterns of both formulations in the raw state are shown in . It could be seen, from the reflection peaks of both formulations, the presence of characteristic peaks of main phases of stevensite-clay such as dolomite (CaMg(CO)3), stevensite (Ca0.2Mg2.9Si4O10(OH)2 . 4H2O) and quartz (SiO2), and that of aluminum hydroxide (Al(OH)3) which was added to obtain stoichiometric composition of cordierite phase. The characteristic peaks of mullite (3Al2O3 . 2SiO2) were observed only for formulation A, which contains fly ash that consisted mainly of the mullite phase.
3.2. Phase evolution of prepared samples
The evolution of the crystalline phases with heat treatment of both formulations was examined by DTA/TGA, XRD, and FTIR measurement.
The structural evolution of both formulations upon heating in the temperature range 25–1100°C, using TGA-DTA analysis is shown in . It could be seen from the TG trend of both formulations a broad peak in the range temperature 50–200°C, which is associated with a weight loss of 1.5 wt.% for formulation A and 4 wt.% for formulation B. This peak can be attributed to the removal of physisorbed water on the surface of particles and the dehydration of zeolitic water of stevensite clay [Citation28,Citation29]. The endothermic peak observed at 300°C can be allocated to the dehydroxylation of aluminum hydroxide. The endothermic peak observed at 530°C for formulation B could be assigned to the dehydroxylation of the stevensite phase [Citation30]. This peak overlapped for formulation A with the exothermic phenomenon attributed to the combustion of the unburned matter of CFA. The observed endothermic peak around 750°C for both formulations, with a weight loss of 1.5 wt.% for sample A and 1 wt.% for sample B, could be contributed to the decomposition of the dolomite phase of ghassoul clay [Citation31]. The appearance of a small exothermic peak at 1000°C for both formulations could be due to the neoformed phase.
XRD patterns of the prepared formulation heated in the range 800–1250°C are shown in . The X-ray diffraction pattern of sample A heated at 800°C revealed the presence of characteristic peaks of diopside CaMg(SiO3)2, mullite (3Al2O3.2SiO2) (PDF#79–1454), and that of quartz (SiO2) (PDF#65–0466), while the formulation B showed only that of diopside and quartz. The disappearance that of stevensite-clay such as stevensite and dolomite phases indicated their transformation into diopside phase according to the reaction suggested by Liittge and Metz [Citation32], in which dolomite can react with quartz to obtain diopside phase. A further increase in the heating temperature to 900°C caused the transformation of the diopside phase into clinoenstatite for formulation A and to enstatite phase for formulation B. The crystallization of enstatite was not observed for formulation B, which indicated the high reactivity of starting materials. The increase in temperature to 1000°C for formulation B induced the transformation of enstatite into the clinoenstatite phase. At 1100°C, the diffractograms showed the crystallization of cristobalite from amorphous silica, and the transformation of clinoenstatite into protoenstatite for formulation A. When the heating temperature reached 1200°C, X-ray intensities of formulation A indicated the formation of the cordierite phase and spinel phase, while that of formulation B showed the crystallization of corundum and the transformation of clinoenstatite into protoenstatite phase. A further increase in the heating temperature up to 1250°C, for both formulations, induced high crystallinity of cordierite with the presence of spinel as an intermediate phase.
Figure 4. XRD patterns of the heated formulations at the range 800–1250°C (d: Diopside, M: Mullite, q: Quartz, L: Clinoenstatite, c: cristobalite, p: protoenstatite, S: Spinel, I: cordierite, r: corindon, e: Enstatite).
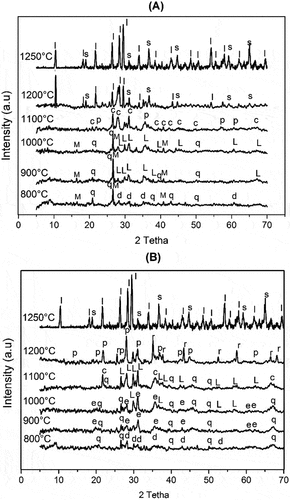
It was therefore possible to propose a justified mechanism to explain the reactions leading to the crystallization of cordierite and those that gave a rise to secondary phases during this process. This transformation can be done by the following reaction:
FTIR analysis of the heated samples in the temperature range 800–1250°C is shown in . The bands located at 1075, 970, 930, and 470 cm−1, for both samples heated at 800°C, were allocated to the diopside phase [Citation33]. The bands observed at 1100 and 797 cm−1, in the range of 800–1000°C for both samples, were linked to the silica phase [Citation10], while that observed at 670 and 560 cm−1 for sample B were attributed to the mullite phase of coal fly ash [Citation32]. The bands centered at 1090, 930,995,845, 730, 670, and 590 cm−1, for both formulations heated in the temperature range 900–1100°C, were allocated to the enstatite forms [Citation34,Citation35]. The bands centered at 1164 and 514 cm−1 were ascribed to the cristobalite formation while that appeared at 640 cm−1 was assigned to the alumina phase. At 1200°C, the band that appeared at 697 cm−1 was associated with spinel formation [Citation10,Citation36,Citation37] and the bands centered at 1160, 950,770, 670, 580, and 480 cm−1 were assigned to cordierite crystallization [Citation10,Citation34]. The absorbance of cordierite bands increased as the heating temperature increased up to 1250°C.
3.3. Dilatometric analysis
The variation of the linear shrinkage for both formulations is shown in . During heat treatment, the dilatometric curve of both formulations revealed the presence of a series of linear shrinkage and expansion domains. In the range of 25–800°C, both formulations had a plateau at around 200°C indicating phase stabilization due to the pre-calcination of starting materials up to 800°C. From 800°C to 1200°C, dilatometric curves showed a linear shrinkage of 6.52% and 5.9% for formulations A and B, respectively, which can indicate the phase transitions into enstatite forms. In addition, the crystallization of the cordierite phase can be escorted by a liquid phase formation, which leads to a quick and significant change in the slop of dilatometric cures above 800°C for formulation A and from 1000°C for formulation B. These changes approve the phase transformations revealed by X-ray diffraction and FTIR measurement. The formation of the liquid phase can be useful for the mechanical properties of sintered specimens.
3.4. SEM observation
shows the cross-section microstructure of both formulations sintered at 1250 ◦C. It could be seen that the microstructure of formulation B had a porous texture with an irregular distribution. The presence of a large hole, ranging at approximately 10 μm in the structure of this formulation can be due to the incomplete densification of the matrix body. SEM imaging analysis of sample A revealed a regular and structured texture which is known for its good mechanical behaviors. The liquid phase that appeared in the texture of both formulations can be explained by phase transitions into cordierite.
3.5. Mechanical proprieties
The flexural strength measurement of sintered samples in the temperature range of 1000–1200°C is given in . The mechanical strength of formulation A increased from 10 MPa to 15 MPa, as a function of the temperature increase from 1000°C to 1200°C, while that of formulation B increased from 2 MPA to 5 MPa. It can be seen from the trends of both formulations, a significant difference in mechanical strength values. This difference can be related to starting materials used with stevensite clay, in which formulation A consisted of CFA and stevensite. This formulation showed high reactivity and the appearance of crystalline phases known by their high mechanical strength such as the cordierite phase and spinel. Otherwise, both formulations showed high mechanical strength than that of sintered stevensite-rich clay alone, which was reported in our previous work [Citation27].
4. Conclusion
Cordierite based-ceramics have been successfully prepared mainly from ghassoul and the mixture of ghassoul clay and CFA. Silica gel and aluminum hydroxide were added to obtain the stoichiometric compositions of the cordierite phase. The heating of both formulations in the range of 900–1100°C involved the transformation of, the main phase of ghassoul, stevensite into clinoenstatite and protoenstatite phase, and the appearance of cristobalite phase. Further heating involved the crystallization of the cordierite phase at 1250°C for the formulation consisted mainly of ghassoul clay, while that of the mixture of ghassoul and CFA, cordierite phase occurred at a low temperature 1200°C. The addition of CFA to ghassoul clay enhanced its reactivity and increased the mechanical strength from 5 MPa to 15 MPa at 1200°C. The crystallization of cordierite phases at low temperature (1200°C), along with interesting technical properties, demonstrates the feasibility of using CFA and ghassoul Morrocan clay, by benefiting from its high contents of silica and magnesia, to produce clean glass-ceramics.
Acknowledgment
The authors gratefully acknowledge the center of analysis and characterization (CAC) of Cadi Ayyad University (Morocco) for providing instrumental facilities for sample characterizations.
Disclosure statement
No potential conflict of interest was reported by the authors.
References
- Nadafan M, Majidi R. Study of optical constants and dielectric properties of nanocrystalline α-cordierite ceramic. J Asian Ceram Soc. 2020;8(2):502–509.
- Krivoshapkina EF, Krivoshapkin PV, Vedyagin AA. Synthesis of Al2O3–SiO2–MgO ceramics with hierarchical porous structure. J Adv Ceram. 2017;6(1):11–19.
- Han L, Song J, Lin C, et al. Crystallization, structure and properties of MgO-Al2O3-SiO2 highly crystalline transparent glass-ceramics nucleated by multiple nucleating agents. J Eur Ceram Soc. 2018;38:4533–4542.
- Lao X, Xu X, Jiang W, et al. Effect of excess MgO on microstructure and thermal properties of cordierite ceramics for high-temperature thermal storage. Ceram Int. 2019 ;45 ;:22264–22272.
- Naga SM, Sayed M, Wassel AR, et al. Processing of electric ceramic insulators from slate rocks and MgO. Mater Manuf Process. 2020;00:1–8.
- Benhammou A, El Hafiane Y, Abourriche A, et al. Influence of sintering temperature on the microstructural and mechanical properties of cordierite synthesized from andalusite and talc. Mater Lett. 2016;172:198–201.
- Bejjaoui R, Benhammou A, Nibou L, et al. Synthesis and characterization of cordierite ceramic from Moroccan stevensite and andalusite. Appl Clay Sci. 2010;49:336–340.
- Benhammou A, El Hafiane Y, Abourriche A, et al. Influence of sintering temperature on the microstructural and mechanical properties of cordierite synthesized from andalusite and talc. Mater Lett. 2016;172:198–201.
- Hajjou H, Saâdi L, Waqif M, et al. Effect of silicon source on the crystallization temperature of the cordierite. J Mater Environ Sci. 2016;7:176–186.
- Tabit K, Waqif M, Saâdi L. Crystallization behavior and properties of cordierite synthesized by sol-gel technique and hydrothermal treatment. J Aust Ceram Soc. 2019;55:469–477.
- Ladjama S, Grid A, Touati N, et al. Synthesis and characterization of cordierite ceramic through reaction sintering kaolin and spent magnesia-carbon bricks. J Phys Conf Ser. 2021;1795:012060.
- Fuertes V, Berges C, Gallego A, et al. Tailoring dielectric properties of cordierite-mullite ceramics through ceramic injection moulding. Mater Sci Eng B Solid-State Mater Adv Technol. 2020;262:114783.
- Xu X, Lao X. Effect of MgO/SiO2 ratio and Al2O3 content on crystallization behavior and properties of cordierite-based glass–ceramics. J Eur Ceram Soc. 2021;41:1593–1602.
- Banjuraizah J, Mohamad H, Ahmad ZA. Crystal structure of single phase and low sintering temperature of alpha-cordierite synthesized from talc and kaolin. J Alloys Compd. 2009;482:429–436.
- Janković-Častvan I, Lazarević S, Jordović B, et al. Electrical properties of cordierite obtained by non-hydrolytic sol-gel method. J Eur Ceram Soc. 2007;27:3659–3661.
- Goren R, Ozgur C, Gocmez H. The preparation of cordierite from talc, fly ash, fused silica and alumina mixtures. Ceram Int. 2006;32:53–56.
- Zhu P, Wand LY, Hong D, et al. A study of cordierite ceramics synthesis from serpentine tailing and kaolin tailing. Sci Sinter. 2012;44:129–134.
- Al-Harbi OA, Özgür C, Khan MM. Fabrication and characterization of single phase cordierite honeycomb monolith with porous wall from natural raw materials as catalyst support. Ceram Int. 2015;41;3526–3532.
- Lao X, Xu X, Jiang W, et al. Effect of aluminum on performances of cordierite-SiCw composite ceramics for high-temperature sensible thermal storage. J Alloys Compd. 2019;780:378–387.
- Romero AR, Elsayed H, Bernardo E. Highly porous cordierite ceramics from engineered basic activation of metakaolin/talc aqueous suspensions. J Eur Ceram Soc. 2020;40:6254–6258.
- Yahya AM, El-Kalioby BA, Soltan AMM, et al. Cordierite ceramic through glass and ceramic routes from kaolin and talc. Egypt J Chem. 2021;64:1751–1758.
- Misrar W, Loutou M, Saadi L, et al. Cordierite containing ceramic membranes from smectetic clay using natural organic wastes as pore-forming agents. J Asian Ceram Soc. 2017;5:199–208.
- Misrar W, Loutou M, Saadi L, et al. Effect of the addition of the calcareous algae on the microstructural properties and filtration performances of membranes manufactured from Ghassoul Moroccan clay. Ceram Int. 2020;46:629–640.
- Tabit K, Waqif M, Saadi L. Anorthite-cordierite based binary ceramics from coal fly ash and steel slag for thermal and dielectric applications. Materials Chemistry and Physics. 2020;254:123472.
- Tabit K, Hajjou H, Waqif M, et al. Cordierite-based ceramics from coal fly ash for thermal and electrical insulations. Silicon. 2020;13:327–334.
- Hajjou H, Saâdi L, Waqif M. Synthesis of cordierite using industrial waste fly ash. Arab J Geosci. 2017;10:359.
- Hajjou H, Benlhachemi A, Saâdi L, et al. Proceedings reactive sintering behavior of Moroccan clay “ Ghassoul ”. Mater Today Proc. 2020;22:112–119.
- Madejová J. FTIR techniques in clay mineral studies. Vib Spectrosc. 2003;31:1–10.
- Chahi A, Duplay J, Lucas J. Analyses of palygorskites and associated clays from the origin of formation chatky dotomite pink mart pink gypsite. Clays Clay Miner. 1993;41:401–411.
- Benhammou A, Tanouti B, Nibou L, et al. Mineralogical and physicochemical investigation of mg-smectite from jbel ghassoul, Morocco. Clays Clay Miner. 2009;57:264–270.
- Dollimore D, Shively TL, Kneller WA, et al. The thermal decomposition of dolomite samples. Proc Conf North Am Therm Anal Soc. 1998;165(26th):217–218.
- Liittge A, Metz P. Mineralogy and mechanism and kinetics of the reaction : a comparison of rock-sample and of powder experiments. Contributions to Mineralogy and Petrology. 1993;2:155–164.
- Diella V, Bocchio R, Caucia F, et al. New insights for gem-quality mn-bearing diopside-omphacite, violane variety, from saint marcel (Val D’Aosta, Italy): its trace elements and spectroscopic characterization. Minerals. 2021;11:1–21.
- Kanti Naskar M, Chatterjee M. A novel process for the synthesis of cordierite (Mg2Al4Si5O18) powders from rice husk ash and other sources of silica and their comparative study. J Eur Ceram Soc. 2004;59:998–1003.
- Liu X, Liu X, Hu Y. Investigation of the thermal decomposition of talc. Clays Clay Miner. 2014;62:137–144.
- Sitarz M, Handke M, Mozgawa W. Identification of silicooxygen rings in SiO 2 based on IR spectra. Spectrochimica Acta Part A: Molecular and Biomolecular Spectroscopy. 2000;56:1819–1823.
- Tabit K, Waqif M, Saâdi L. Crystallization behavior and properties of cordierite synthesized by sol-gel technique and hydrothermal treatment. J Aust Ceram Soc. 2019;55:469–477.