ABSTRACT
Listeria monocytogenes is a high risk pathogen which can cause invasive diseases in humans. We previously reported that black-headed gulls from Dianchi Lake of Kunming carrying L. monocytogenes, while the characteristics of these isolates and the relationship with habitats of migratory gulls have not been explored. In this study, we investigated the prevalence and molecular characteristics of Listeria monocytogenes from black-headed gulls in Dianchi Lake, and phylogenetic analysis based on core genome SNPs was used to determine the genetic relationship of the strains from Dianchi Lake and other regions. Occurrence of L. monocytogenes in black-headed gull feces in 2016, 2017 and 2018 was 1.0%, 1.0% and 0.6% respectively. The predominant serotype of 28 isolates was 4b, while the predominant sequence types were ST145 and ST201. Based on their prevalence and genomic relationships, ST5 and ST87 were likely to be sourced locally while ST145 and ST201 were likely to be non-local. L. monocytogenes may travel along the bird migration route leading to transmission over a large geographical span carried by black-headed gull. Although the prevalence of L. monocytogenes was low, its carriage by the migratory black-headed gulls poses potential public health risks in regions where the migratory birds passage and reside.
Introduction
Listeria monocytogenes is a Gram-positive bacterium which can cause food-borne diseases in humans. It is an intracellular bacterium and has the ability to penetrate the intestinal, blood–brain and placental barriers, and cause a series of clinical conditions such as diarrhea, septicemia, meningoencephalitis and miscarriage [Citation1,Citation2]. L. monocytogenes is also a multihost bacterium which can cause listeriosis in many domestic animals and wild animals including cows, goats, wild rodents and wild birds with similar pathological manifestations [Citation3–9]. L. monocytogenes can cause listeriosis in sporadic cases and outbreaks. Listeriosis has a high mortality rate, and in most cases, immunocompromised, debilitated individuals are especially susceptible to L. monocytogenes. The recent outbreak caused by ready-to-eat (RTE) foods in South Africa had a mortality rate of 28.6%, and the most affected age group was the neonates ≤28 days [Citation10].
Of the 13 L. monocytogenes serotypes, serotype 1/2a, 1/2b and 4b account for most of the human clinical cases, and serotype 4b is responsible for the majority of outbreaks [Citation11]. Most of the key virulence-associated genes are distributed in pathogenicity island 1 (LIPI-1), LIPI-3 and LIPI-4. LIPI-1 is widely distributed in most L. monocytogenes strains, and harbours several important virulence genes, such as the central virulence regulator prfA, the surface protein necessary for actin assembly actA and the phospholipase gene plcA. LIPI-3 encodes listeriolysin S (LLS), which reinforce the cytotoxic and hemolytic activity of L. monocytogenes, and is only harboured by some lineage I strains [Citation12]. LIPI-4 encodes a cellobiose-family phosphotransferase system (PTS) which is present in CC4 and CC87 L. monocytogenes, and contributes to placental and central nervous infections [Citation13,Citation14].
Black-headed gull (Larus ridibundus) is a widely distributed migratory wild bird that has been shown to carry several pathogens such as Influenza A virus, Escherichia coli, Salmonella and potentially pathogenic microfungi [Citation8,Citation15–18]. In China, black-headed gulls migrate to Kunming by way of Siberia, Mongolia, Neimenggu, Xinjiang, Ningxia, Qinghai, Gansu, Shaanxi and Sichuan every year (November to March). In 2016, we isolated L. monocytogenes from black-headed gull feces in Dianchi Lake of Kunming, southwestern China, and found the isolates had potential infection risk for residents and tourists in Kunming [Citation19]. However, the genomic characteristics of L. monocytogenes from black-headed gulls and the relationship between Dianchi Lake isolates and strains from other sources (Kunming and other geographical sites) have not been explored. In this study, we investigated the longitudinal prevalence and molecular characteristics of L. monocytogenes from black-headed gulls in Dianchi Lake and food samples from nearby retail markets. Serotyping, multilocus sequence typing (MLST) and pulsed-field gel electrophoresis (PFGE) were utilized to identify the diversity of L. monocytogenes isolates, and whole genome sequencing (WGS) was utilized to analyze the genetic relationship of strains from diverse origins.
Materials and methods
Sample collection
Black-headed gull fecal samples and environmental swabs of Dianchi Lake were randomly collected in Jan 2017, Nov 2017 and Jan 2018. A total of 2135 black-headed gull fecal samples and 300 environmental swabs were collected. All fecal samples were collected on the beach ground of Dianchi Lake as soon as they were excreted from Black-headed Gull. Feces were collected at a distance of at least 5 m from each other, to avoid collecting samples from the same gull. A total of 388 food samples from 80 booths and 4 restaurants of retail markets which are distributed in three districts proximal to Dianchi Lake, were collected in Nov 2017 and Jan 2018. Samples included pork, beef, frozen meat, mutton, chicken, duck, fish, ready to eat (RTE) foods, and environmental swabs on refrigerators, tables, knives and meat trays.
The fresh feces and environmental swabs were stored in 5 mL brain heart infusion (BHI) broth containing 20% glycerol by using sterile cotton swabs, food samples were obtained with sterile bags, and stored at 4°C before isolation in the laboratory.
Isolation and identification
Listeria was isolated according to ISO 11290 method with partial modifications. Isolation was divided into two step enrichments. For fecal samples, about 2–5 g of feces were collected into 10 mL Half Fraser broth; for environmental swabs, 1 mL of samples were collected into 9 mL Half Fraser broth; for food samples, 25 g of sample was added to 225 mL Half Fraser broth, and incubated at 30°C for 24 h. Then 1 mL of primary enrichment cultures were added to 5 mL Fraser broth and incubated at 37°C for 48 h. Two loopful of secondary enrichment broths were steaked on the Chromogenic Listeria Agar (Oxoid, Basingstoke, UK), incubated at 37°C for 48 h. Five presumptive positive colonies were selected for each samples, and each colony was identified by PCR for Listeria genus-specific gene prs and species-specific gene lmo0733. Primers were shown in Table S1.
Serotyping, MLST and PFGE analysis
L. monocytogenes isolates were serotyped by multiplex PCR assay and antigen serum agglutination assay (Denka Seiken, Japan) [Citation20]. Multilocus sequence typing (MLST) analysis was performed by sequencing seven housekeeping genes. Housekeeping genes included abcZ, blgA, cat, dapE, dat, ldh, lhkA, and primers are shown in Table S1. Through submitting sequences to the Institute Pasteur L. monocytogenes MLST database (http://bigsdb.pasteur.fr/listeria/listeria.html), ST type of each isolates were confirmed [Citation21,Citation22]. For PFGE analysis, AscI restriction enzymes were used to subtyping according to the PulseNet protocol of L. monocytogenes in the Chinese Centers for Disease Control and Prevention [Citation23].
Whole genome sequencing
The 29 isolates from Dianchi Lake 10 representative market-source isolates which have the same sequence types or pulsotypes with isolates from the Black-headed gulls, ICDC-LM497 (ST14, isolated from food in Xinjiang province, preserved in the China CDC) and ICDC-LM2875 (ST145, isolated from human in Hebei province, preserved in the China CDC) were selected for sequencing (Table S2). Selected isolates were cultured in brain–heart infusion broth (BHI) at 37°C overnight. DNA was extracted by Wizard® Genomic DNA Purification Kit according to the manufacturer’s introduction. Purified DNA was detected by agarose gel electrophoresis and quantified by Qubit. Whole-genome sequencing was performed on the Illumina Hiseq PE150 platform by Novogene (Beijing, China) using L. monocytogenes EGD-e as the reference strain, and the genome sequences were assembled into a number of scaffolds by SOAP denovo (version 2.04).
SNP-based phylogenetic analysis
Sequenced strains and other strains with genome sequences available online sharing the same sequence type with isolates from Dianchi Lake (except strains from unknown region) were chosen for comparison (Table S2). SNPs were detected through comparisons of L. monocytogenes genomes using MuMer, and each SNP was not positioned at a recombination region and the distance between two SNP sites ≥5 reads. The maximum likelihood trees were constructed by MEGA7 based on the core genome SNPs. Tamura-Nei model was used, and bootstraps were performed with 1,000 replicates.
Identification of virulence genes
For virulence identification, the 29 isolates from Dianchi Lake were analyzed on website of Center for Genomic Epidemiology (https://cge.cbs.dtu.dk/services/VirulenceFinder/), and the analysis were performed with the minimum of 80% length and 85% identity [Citation24].
Results
Occurrence and molecular subtyping of L. monocytogenes isolates from Dianchi Lake black-headed gulls
Sampling was conducted for 3 years from 2016 to 2018. Data of 2016 was previously published and were included in this study for completeness [Citation19]. A total of 3030 black-headed gull feces and 300 environmental swab samples from Dianchi Lake were screened for L. monocytogenes in the three consecutive years. Occurrences of L. monocytogenes in black-headed gull feces in 2016, 2017 and 2018 were 1.0% (9/895), 1.0% (16/1612) and 0.6% (3/523) per year respectively, and only one isolate from Dianchi Lake environment was found in 2017 (0.7%, 1/150) and none in other years. The 29 L. monocytogenes isolates from Dianchi Lake were subtyped into 5 serotypes by serotyping, 9 sequence types by MLST and 11 pulsotypes by PFGE. The predominant serotype was 4b (51.9%), while the predominant sequence types were ST145 and ST201, accounted for 41.8% and 17.2% of isolates respectively. ST3, ST5, ST145 and ST201 were found in two different years (, Figure S1).
Table 1. Prevalence and subtyping characteristics of L. monocytogenes (LM) in Dianchi lake.
To determine the prevalence and genotypes of L. monocytogenes in the local retail markets, 388 food samples and environmental swabs were collected from three retail markets near Dianchi Lake and 64 samples were positive for L. monocytogenes (16.49%). The 64 isolates were divided into 4 serotypes, 14 sequence types and 25 pulsotypes. The predominant serotype and sequence type were 1/2b (42.2%) and ST87 (28.1%) respectively, and the subtypes shared between Dianchi Lake and retail markets were ST2 (PT34), ST3, ST5 (PT22) and ST87 (PT30) (, Figure S1).
Genomic relationships of L. monocytogenes isolates from Dianchi Lake black-headed gulls
The 29 L. monocytogenes isolates with 28 from the Dianchi Lake black-headed gulls and 1 from Dianchi Lake environment were chosen for whole genome sequencing. The general genome characteristics were shown in . Core genome SNPs were used to construct a phylogenetic tree by the Neighbor-joining method, and Listeria ivanovii PAM55 was used as outgroup. The 29 L. monocytogenes isolates were divided into 3 clusters which are consistent with the three Lineages (I-III) previously defined [Citation25] ().
Figure 1. Virulence profiles across the phylogeny of 29 isolates from Dianchi Lake. The phylogenetic analysis based on SNP of core genes using Neighbor-joining method with 1000 bootstrap replicates implemented in the MEGA, and PAM55 (L. ivanovii) was used as outgroup. The presence (colour line)/ absence (grey) gene matrix represents, from left to right, genes comprised of LIPI-1 (prfA, plcA, plcB, hly, mpl, atcA), LIPI-3 (llsAGHXYDP), LIPI-4 (Clip80459_02324 to Clip80459_02329) and other genes involved in internalization (inlABCFHJK), adherence (ami, dltA, fbpA, lap, lapB), invasion (aut, iap, ipeA, recA, vip), intracellular survival (clpB, clpc, clpe, clpp, dal, fri, htrA, lplA1, oppA, perR, prsA2, pycA, relA, sipZ, sod, svpA, tig, uHpt), regulation of transcription and translation (agrA, ctsR, gmar, fur, hfq, lhrC, lisK, lisR, mogR, rli55, rli60, rsbv, sigB, stp, virR), surface protein anchoring (lgt, lsp, secA2, sipX, srtA, srtB), peptidoglycan modification (degU, murA, oatA, pgdA), immune modulation (chiA, lipA, intA, pgl, tcsA), bile-resistance (bsh, bilE), teichoic acid biosynthesis (gtcA), motility (flaA, flgC, flgE), membrane integrity (ctaP, mprF) and metabolic regulator (codY).
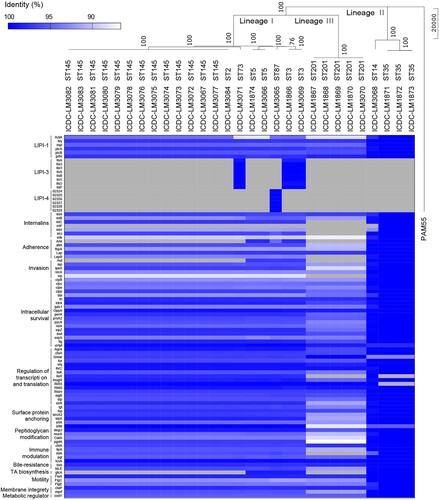
Table 2. Genome information of L. monocytogenes isolates from Dianchi lake.
To obtain the highest resolution relationships of isolates within an ST, we further constructed different phylogenetic trees based on the core genome SNPs for the 8 STs (ST2, ST3, ST5, ST14, ST35, ST87, ST145 and ST201) separately. These STs contained multiple isolates in this study and isolates from other regions or countries. We describe the results by grouping STs with potential transmission source for Dianchi Lake black-headed gull isolates.
STs that contained isolates from foreign countries
ST145 contained 15 isolates including 13 isolates from Dianchi Lake black-headed gulls, and one isolate each isolated from humans in Hebei province of China and Russia respectively. The tree was routed by an ST2 strain. The 13 Dianchi Lake black-headed gull isolates were grouped together and shared a closest relationship with the Hebei isolate with 2–3 SNP differences. The next isolate closest to was a Russian isolate with 8–9 SNP differences (A, ).
Figure 2. Phylogenetic trees of CCs/ STs based on SNP of core genes. Each CCs/ STs tree was analyzed using Maximum Likelihood method with 1000 bootstrap replicates implemented in the MEGA. (A) CC2 phylogenetic tree, VIMVR081 was used as reference strain. (B) ST3 phylogenetic tree, R2-502 was reference strain. (C) ST5 phylogenetic tree, J2-064 was reference strain. (D) ST14 phylogenetic tree, NRRL B-33805 was reference strain. (E) ST35 phylogenetic tree, EGD-e was reference strain. (F) ST87 phylogenetic tree, ICDC-LM188 was reference strain. (G) ST201 phylogenetic tree, M7 was reference strain. Each colour of node denotes the country which strains comes from. And isolates from black-headed gull feces, environmental swabs in Dianchi Lake and retail markets were shaded in red, green and yellow label background respectively. And the notes beside isolates represent the isolate date. Mar16, March 2016; Jan17, January 2017; Nov17, November 2017; Jan18, January 2018.
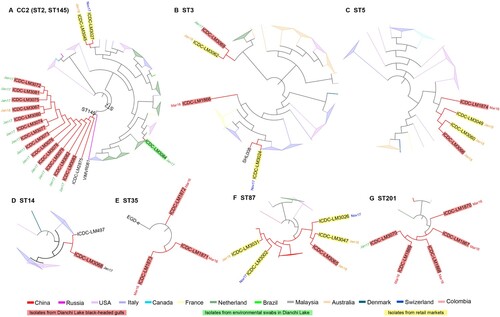
Table 3. The number of SNPs between L. monocytogenes isolates from Dianchi Lake and other regions.
ST201 contained 5 isolates from Dianchi Lake black-headed gulls and 1 isolate from other parts of china and 2 isolates from other countries. The black-headed gull isolates were more closely related to isolates from other countries (The Netherlands and USA) with 8–14 SNP differences than to another China isolate with 15–19 SNP differences (G, ).
STs that contained isolates from local markets
ST5 contained 94 isolates (including 2 isolates from Dianchi Lake black-headed gulls, 2 isolates from Kunming local retail markets, and 90 isolates from other regions). The two black-headed gull isolates and the two local market isolates were grouped together as one cluster and the smallest SNP difference between black-headed gull isolates and the local market isolates were only 8 SNPs. The Dianchi Lake black-headed gull isolates were separated from isolates from other countries by 58–262 (median, 126) SNPs (C, ).
ST87 contained 25 isolates (including 1 isolate from a Dianchi Lake black-headed gull, 4 isolates from Kunming local retail markets, and 20 isolates from other regions). All isolates from China were on one clade. The Dianchi Lake black-headed gull isolate was closest to local market isolates and shared 25–35 (median, 29) SNPs with isolates from retail markets and 26–32 (median, 23.5) SNPs with isolates from other regions of China respectively. Moreover, other isolates from four countries were grouped into 4 independent clades (F, ).
Other STs
ST3 contained 43 isolates (including 2 isolates from Dianchi Lake black-headed gulls, 2 isolates from Kunming local retail markets, and 39 isolates from other regions). The two Dianchi Lake black-headed gull isolates, ICDC-LM3069 and ICDC-LM1866 were distributed in two clades, ICDC-LM3069 was grouped together with local market isolate ICDC-LM3062 with 62 SNP differences, while ICDC-LM1866 stand by its own on the phylogenetic tree (B, ).
ST14 comprised of 15 isolates (including 1 isolates from a Dianchi Lake black-headed gull and 14 isolates from other regions). Dianchi Lake isolate ICDC-LM3068 was grouped together with a Xingjiang food isolate ICDC-LM497 with 26 SNP differences while isolates from different countries were well separated from them. (D, ).
ST35 had 4 isolates (3 isolates from Dianchi Lake black-headed gulls and a reference strain EGD-e from Europe). The four isolates were closely related to each other and share 11 SNPs (E, ).
ST2 contained 56 isolates (including 1 isolate from Dianchi Lake environment, 2 isolates from Kunming local retail markets, and 53 isolates from other regions) which formed multiple clades. The Dianchi Lake environmental isolate ICDC-LM3084 was closer to an isolate from France with 52 SNP differences than to the two isolates from the local markets with 123–130 SNP differences which were also well separated on the tree (A, ).
Virulence genes identification of L. monocytogenes isolates from Dianchi Lake black-headed gulls
All isolates from Dianchi Lake were positive for LIPI-1, and contained the majority of other virulence genes, and each ST has similar virulence profiles. LIPI-3 was found in ST3 and ST73 while LIPI-4 was only found in ST87 ().
Discussion
Migratory birds are susceptible to various pathogens such as Avian influenza virus, Salmonella and Campylobacter [Citation8,Citation15–18,Citation26]. Some wild water birds like black-headed gull could be the ultimate source of Influenza A virus and natural reservoir of potentially other pathogens, and spread the pathogens to poultry, mammals and humans [Citation18,Citation27]. In China, black-headed gulls migrate from Xinjiang, Mongolia and Siberia to Dianchi Lake of Kunming every year (). And the gulls usually perch at the beach of Dianchi Lake from November to March, there are abundant opportunities for the birds to contact with local residents and tourists. L. monocytogenes had been isolated from black-headed gull feces in Dianchi Lake previously [Citation19]. However, the relationship between the source of the L. monocytogenes isolates and the wide habitats of migratory gulls is unclear. To address these questions, we carried the surveillance on L. monocytogenes in black-headed gulls in Dianchi Lake for three years.
Figure 3. Migratory map of black-headed gulls from Kunming. The migratory information comes from International Union for Conservation of Nature, IUCN (https://www.iucnredlist.org/species/22694420/132548687#assessment-information) and Kunming Institute of Zoology, Chinese academy of sciences (http://www.kiz.cas.cn/xwzx/news5/201706/t20170623_4817599.html). Different shades of yellow on geographic range denote the black-headed gulls’ scope of activity. Phylogenetic trees of ST5, ST87 and ST145 were analyzed using Maximum Likelihood method with 1000 bootstrap replicates implemented in the MEGA. Each colour of node denotes the country which strains comes from, and the colours are consistent with . The red line represents the fecal isolates which isolated from Dianchi Lake black-headed gulls; the mazarine line represents the food isolates which isolated from the retail markets proximal to Dianchi Lake; the green line represents the Russian isolate; the wathet line represents the clinical isolate which isolated from Hebei province.
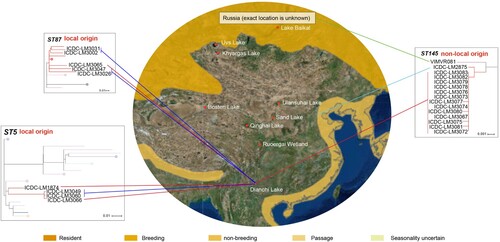
The prevalence of L. monocytogenes in black-headed gull feces averaged 0.87% with 1.0%, 1.0% and 0.6% per year from 2016 to 2018, and the occurrences in environmental samples of Dianchi Lake were 0% except in 2017 with 0.7% [Citation19]. The occurrences of L. monocytogenes in black-headed gull feces were similar to the result of a recent study of pathogen carriage by migratory birds along the Mediterranean- Black Sea Flyway at 0.6% of L. monocytogenes [Citation28]. Of the 28 L. monocytogenes isolates obtained from black-headed gulls, 50% of the isolates were serotype 4b which is known to be responsible for the majority of listeriosis outbreaks reported [Citation11]. All L. monocytogenes isolates from Dianchi Lake black-headed gulls contain LIPI-1 and inlA/B. Further ST3 and ST73 isolates carried LIPI-3 while ST87 isolates carried hypervirulent LIPI-4. Most isolates contained other virulence-related genes which are shown in . Although the incidence of L. monocytogenes was relatively low, the isolates carried by the gulls might be a potential risk to cause listeriosis in humans, and our 3 years of surveillance showed that L. monocytogenes carriage by black-headed gulls was an ongoing problem.
L. monocytogenes is a foodborne bacterium which is widely distributed in foods and food processing environments. Thus food products and environmental conditions would be reflective of the local epidemiology of L. monocytogenes. In this study, the prevalence of L. monocytogenes in retail markets near Dianchi Lake (16.49%) was close to the previous study in Kunming (16.2%) and the isolates share similar subtypes with others studies on Chinese food retail markets [Citation14,Citation29–31].
In this study, we also surveyed retail markets for L. monocytogenes to determine whether there was any link between L. monocytogenes isolates from Dianchi Lake black-headed gulls and local isolates and possible inter-transmission of L. monocytogenes between the gulls and local environment. The predominant subtypes of L. monocytogenes from black-headed gulls and retail markets were completely different, however, they shared some STs and/or PTs including ST3, ST5 (PT22) and ST87 (PT30). For ST5 and ST87, isolation rate from local food source was 7.8% and 28.1% of food isolates respectively, similar to other studies in China [Citation14,Citation30]. These STs were frequently isolates from food sources and ST87 is the predominant ST causing human listeriosis in China [Citation32,Citation33], pointing to potential local source of some STs of L. monocytogenes from the black-headed gulls.
Genome sequencing offered a higher resolution to address these questions. However, it should be noted that it is difficult to use a SNP cutoff to determine the epidemiological link of isolates in Listeria. Isolates of the same origin years apart may differ by very few SNPs while isolates from the same outbreak may differ by up to 50 SNPs [Citation34]. Reimer et al analyzed 9 outbreaks and showed that epidemiologically confirmed outbreak isolates can differ by as much as 30 SNPs [Citation35]. The mutation rate of L. monocytogenes was reported to be 0.4 SNPs per genome per year [Citation21], which cannot be used to easily reconcile these observations. Nevertheless, we used 3 criteria to assess the likely origin of the L. monocytogenes from the black-headed gulls: (1) number of SNP differences between isolates of different sources; (2) comparison with local retail market isolates, isolates from other parts of China and isolates from other countries; and (3) regional prevalence of different STs. The inference of origin doesn’t suggest a direct epidemiological link of the same point source, for example, from the retail market where we sampled.
For ST5, the 2 gull isolates and the food isolates were grouped together, with the 2016 gull isolate diverged earlier. The 2018 gull isolate differed from one of the 2018 local market isolate by only 8 SNPs. For the ST87 isolates, the gull isolate is also closest to a local market isolate from the same year with 25 SNP differences. These two STs were likely to be sourced locally.
The source of the ST145 isolates carried by black-headed gulls was likely to be non-local and possibly from its breeding location in its origin. ST145 belongs to serotype 4b and was rarely reported worldwide. Isolates from Dianchi Lake black-headed gulls were closely related to an isolate from Hebei in the north of China and isolates from Russia. ST145 has never been reported to cause disease in China before, there is no more case reported in Hebei so far, and the transmission pathway for the only isolate from Hebei was unknown due to the lack of epidemiological information and there were no more ST145 isolates reported. In contrast, ST145 strains have been reported in river waters and rodents in southern Russia [Citation36–38]. It is likely that the black-headed gulls carried ST145 L. monocytogenes from another location like southern Russia to Dianchi Lake. Further studies will be required to confirm this inference using tracking data. For ST201, the source was also likely to be non-local. The 5 gull isolates were closer to each other and closer to isolates from other countries with 8 SNP differences. The ST wasn’t sampled from the local market and was rare in China [Citation39,Citation40].
For ST3, ST14, and ST35 isolates, the source is unclear as no closely related local or foreign isolates were found. The Dianchi Lake black-headed gull ST3 isolate (ICDC-LM3069) was grouped with a local market isolate with 62 SNP differences while the ST14 isolate (ICDC-LM3068) was grouped with a food-source isolate from Xinjiang with 26 SNP differences. It is indeterminant the source of the ST3 isolates. However, for the ST14 isolate, it is possible that black-headed gull acquired the ST14 isolate in Xinjiang which was known to be a stopover locale for black-headed gull migration.
It is also interesting to note that isolates from black-headed gull from different years may be closer to each other than with other sources (for example ST145 isolates), suggesting that the same L. monocytogenes population may be maintained in the black-headed gull population or the black-headed gulls may obtain L. monocytogenes from the same source in different years. Both scenarios are possible considering that black-headed gulls are territorial with lifespan of up to 30 years and may pass through the same locale every year [Citation41]. It has been shown that L. monocytogenes can persist in birds for long periods of time by carriage [Citation42]. It is also possible that there are other sources of L. monocytogenes such as the other avian species which transmit the pathogen through the common environment. Further studies are required to address these questions.
Our results suggest that black-headed gulls could facilitate L. monocytogenes spread across geographical regions through migration, concurring with previous studies showing that pathogens could spread to another geographically distant sites through migratory birds [Citation43,Citation44]. Although the prevalence of L. monocytogenes in black-headed gulls was low, our findings underscore the importance of surveillance of L. monocytogenes in the migratory black-headed gulls, which would be helpful to reduce the infection risk to humans in regions where the migratory birds reside.
Supplemental Material
Download Zip (592.2 KB)Acknowledgement
Lin Gan, Changyun Ye and Jianguo Xu conceived and designed the research study. Lin Gan, Xiaolong Cao, Yan Wang, Yiqian Wang, Huaying Jiang and Changyun Ye performed the sample collection. Lin Gan and Xiaolong Cao performed experiments. Lin Gan and Ruiting Lan analyzed the data. Lin Gan, Changyun Ye and Ruiting Lan wrote the manuscript. All authors have read and approved the final version of the article. Thank Shijiazhuang Center for Disease Control and Prevention (ICDC-LM2875) and Xinjiang Center for Disease Control and Prevention (ICDC-LM497) for strains supply.
Disclosure statement
No potential conflict of interest was reported by the authors.
Correction Statement
This article has been republished with minor changes. These changes do not impact the academic content of the article.
Additional information
Funding
References
- Farber JM, Peterkin PI. Listeria monocytogenes, a food-borne pathogen. Microbiol Rev. 1991;55:476–511.
- Schuchat A, Swaminathan B, Broome CV. Epidemiology of human listeriosis. Clin Microbiol Rev. 1991;4:169–183. doi: 10.1128/CMR.4.2.169
- Ramos JA, Domingo M, Dominguez L, et al. Immunohistologic diagnosis of avian listeriosis. Avian Pathol. 1988;17:227–233. doi:10.1080/03079458808436441.
- Nilsson O, Soderlind O. [Listeria monocytogenes isolated from animals in Sweden during 1958–1972]. Nord Vet Med. 1974;26:248–255.
- Hellstrom S, Kiviniemi K, Autio T, et al. Listeria monocytogenes is common in wild birds in Helsinki region and genotypes are frequently similar with those found along the food chain. J Appl Microbiol. 2008;104:883–888. doi:10.1111/j.1365-2672.2007.03604.x.
- Gu Y, Liang X, Huang Z, et al. Outbreak of Listeria monocytogenes in Pheasants. Poult Sci. 2015;94:2905–2908. doi:10.3382/ps/pev264.
- Höfle U, Van de Bildt MW, Leijten LM, et al. Tissue tropism and pathology of natural influenza virus infection in black-headed gulls (Chroicocephalus ridibundus). Avian Pathol. 2012;41:547–553. doi:10.1080/03079457.2012.744447.
- Abulreesh HH, Goulder R, Scott GW. Wild birds and human pathogens in the context of ringing and migration. Ringing Migr. 2007;23:193–200. doi: 10.1080/03078698.2007.9674363
- Zaytseva E, Ermolaeva S, Somov GP. Low genetic diversity and epidemiological significance of Listeria monocytogenes isolated from wild animals in the far east of Russia. Infect Genet Evol. 2007;7:736–742. doi:10.1016/j.meegid.2007.07.006.
- Salama PJ, Embarek PKB, Bagaria J, et al. Learning from listeria: safer food for all. Lancet. 2018;391:2305–2306. doi:10.1016/S0140-6736(18)31206-6.
- Swaminathan B, Gerner-Smidt P. The epidemiology of human listeriosis. Microbes Infect. 2007;9:1236–1243. doi:10.1016/j.micinf.2007.05.011.
- Clayton EM, Daly KM, Guinane CM, et al. Atypical Listeria innocua strains possess an intact LIPI-3. BMC Microbiol. 2014;14:58), doi:10.1186/1471-2180-14-58.
- Maury MM, Tsai YH, Charlier C, Touchon M, et al. Uncovering Listeria monocytogenes hypervirulence by harnessing its biodiversity. Nat Genet. 2016;48:308–313. doi:10.1038/ng.3501.
- Wang H, Luo L, Zhang Z, et al. Prevalence and molecular characteristics of Listeria monocytogenes in cooked products and its comparison with isolates from listeriosis cases. Front Med. 2018;12:104–112. doi:10.1007/s11684-017-0593-9.
- Verhagen JH, Majoor F, Lexmond P, et al. Epidemiology of influenza A virus among black-headed gulls, the Netherlands, 2006–2010. Emerg Infect Dis. 2014;20:138–141. doi:10.3201/eid2001.130984.
- Bonnedahl J, Drobni P, Johansson A, et al. Characterization, and comparison, of human clinical and black-headed gull (Larus ridibundus) extended-spectrum beta-lactamase-producing bacterial isolates from Kalmar, on the southeast coast of Sweden. J Antimicrob Chemother. 2010;65:1939–1944. doi:10.1093/jac/dkq222.
- Coulson JC, Butterfield J, Thomas C. The herring gull Larus argentatus as a likely transmitting agent of Salmonella Montevideo to sheep and cattle. J Hyg (Lond). 1983;91:437–443. doi: 10.1017/S0022172400060472
- Tsunekuni R, Yaguchi Y, Kashima Y, et al. Spatial transmission of H5N6 highly pathogenic avian influenza viruses among wild birds in Ibaraki Prefecture, Japan, 2016-2017. Arch Virol. 2018;163:1195–1207. doi:10.1007/s00705-018-3752-7.
- Cao X, Wang Y, Wang Y, et al. Isolation and characterization of Listeria monocytogenes from the black-headed gull feces in Kunming, China. J Infect Public Health. 2018;11:59–63. doi:10.1016/j.jiph.2017.03.003.
- Doumith M, Buchrieser C, Glaser P, et al. Differentiation of the major Listeria monocytogenes serovars by multiplex PCR. J Clin Microbiol. 2004;42:3819–3822. doi:10.1128/JCM.42.8.3819-3822.2004.
- Moura A, Criscuolo A, Pouseele H, et al. Whole genome-based population biology and epidemiological surveillance of Listeria monocytogenes. Nat Microbiol. 2017;2:16185. doi:10.1038/nmicrobiol.2016.185.
- Ragon M, Wirth T, Hollandt F, et al. A new perspective on Listeria monocytogenes evolution. PLoS Pathog. 2008;4:e1000146. doi:10.1371/journal.ppat.1000146.
- Wang Y, Zhao A, Zhu R, et al. Genetic diversity and molecular typing of Listeria monocytogenes in China. BMC Microbiol. 2012;12:119. doi:10.1186/1471-2180-12-119.
- Joensen KG, Scheutz F, Lund O, et al. Real-time whole-genome sequencing for routine typing, surveillance, and outbreak detection of verotoxigenic Escherichia coli. J Clin Microbiol. 2014;52:1501–1510. doi:10.1128/JCM.03617-13.
- Orsi RH, den Bakker HC, Wiedmann M. Listeria monocytogenes lineages: Genomics, evolution, ecology, and phenotypic characteristics. Int J Med Microbiol. 2011;301:79–96. doi:10.1016/j.ijmm.2010.05.002.
- Broman T, Waldenström J, Dahlgren D, et al. Diversities and similarities in PFGE profiles of Campylobacter jejuni isolated from migrating birds and humans. J Appl Microbiol. 2004;96:834–843. doi: 10.1111/j.1365-2672.2004.02232.x
- Dynowska M, Indykiewicz P, Ejdys E. Black-headed gulls (Chroicocephalus ridibundus)-a natural reservoir of potentially pathogenic microfungi? Biologia. 2018;73:241–246. doi: 10.2478/s11756-018-0030-9
- Najdenski H, Dimova T, Zaharieva MM, Najdenski H, Najdenski H, et al. Migratory birds along the Mediterranean - Black Sea Flyway as carriers of zoonotic pathogens. Can J Microbiol. 2018;64:915–924. doi:10.1139/cjm-2017-0763.
- Wu S, Wu Q, Zhang J, et al. Listeria monocytogenes prevalence and characteristics in retail Raw foods in China. PLoS One. 2015;10:e0136682. doi:10.1371/journal.pone.0136682.
- Li W, Bai L, Fu P, et al. The epidemiology of Listeria monocytogenes in China. Foodborne Pathog Dis. 2018;15:459–466. doi:10.1089/fpd.2017.2409.
- Luo, L., Zhang, Z., Wang, H., et al. A 12-month longitudinal study of Listeria monocytogenes contamination and persistence in pork retail markets in China. Food Cont. 2017;76:66–73. doi:10.1016/j.foodcont.2016.12.037.
- Wang, Y., Jiao, Y., Lan, R., et al. Characterization of Listeria monocytogenes isolated from human listeriosis cases in China. Emerg Microbes Infect 2015;4:e50. doi:10.1038/emi.2015.50.
- Huang YT, Ko WC, Chan YJ, et al. Disease Burden of invasive listeriosis and molecular Characterization of clinical isolates in Taiwan, 2000–2013. PLoS One. 2015;10:e0141241. doi:10.1371/journal.pone.0141241.
- Datta AR, Burall LS. Serotype to genotype: The changing landscape of listeriosis outbreak investigations. Food Microbiol. 2018;75:18–27. doi:10.1016/j.fm.2017.06.013.
- Reimer A, Weedmark K, Petkau A, et al. Shared genome analyses of notable listeriosis outbreaks, highlighting the critical importance of epidemiological evidence, input datasets and interpretation criteria. Microb Genom. 2019;5. doi: 10.1099/mgen.0.000237
- Voronina Olga, L., Ryzhova Natalia, N., Kunda Marina, S. et al. Diversity and pathogenic potential of listeria monocytogenes isolated from environmental sources in the Russian Federation. 2015.
- Adgamov RR, Timchenko NF, Zaitseva EA, et al. Ecological and genetic mechanisms of development of epidemiologically significant strains of sapronosis causative agents. Biology Bulletin Reviews. 2013;3:125–138. doi: 10.1134/S2079086413020023
- Adgamov R, Zaytseva E, Thiberge JM, et al. Genetic diversity in microorganisms. InTech; 2012. Chapter 9, Genetically related Listeria monocytogenes strains isolated from Lethal human cases and wild animals.
- Chen J, Zhang X, Mei L, et al. Prevalence of Listeria in Chinese food products from 13 provinces between 2000 and 2007 and virulence characterization of Listeria monocytogenes isolates. Foodborne Pathog Dis. 2009;6:7–14. doi:10.1089/fpd.2008.0139.
- Chen J, Xia Y, Cheng C, et al. Genome sequence of the nonpathogenic Listeria monocytogenes serovar 4a strain M7. J Bacteriol. 2011;193:5019–5020. doi:10.1128/JB.05501-11.
- Evans MHR, Lihou KL, Rands SA. Black-headed gulls synchronise their activity with their nearest neighbours. Sci Rep. 2018;8:9978. doi:10.1038/s41598-018-28378-x.
- Gray ML. Listeriosis in Fowls: A Review. Avian Dis. 1958;2:296–314. doi:10.2307/1587530.
- Prosser DJ, Cui P, Takekawa JY, et al. Wild bird migration across the Qinghai-Tibetan plateau: a transmission route for highly pathogenic H5N1. PLoS One. 2011;6:e17622. doi:10.1371/journal.pone.0017622.
- Liu J, Xiao H, Lei F, et al. Highly pathogenic H5N1 influenza virus infection in migratory birds. Science. 2005;309:1206. doi:10.1126/science.1115273.