ABSTRACT
For Pseudomonas aeruginosa (PA), infection control and appropriate antimicrobial treatment have become important issues. Diagnosis is critical in managing PA infection, but conventional methods are not highly accurate or rapid. We developed a new PA quantification system based on 23S rRNA-targeted reverse transcription quantitative PCR (RT-qPCR). We confirmed that RT-qPCR can quantify PA directly from clinical samples quickly (within 6 h) and with high sensitivity (blood, 1 cell/mL; stool, 100 cells/g) and without cross-reaction. Also, under antibiotic treatment, PA viable counts detected by this system correlated well with the inflammatory response of infected Caco-2 cells compared to other methods such as culturing and qPCR. Next, we utilized this system on fecal samples collected from 65 septic ICU patients and 44 healthy volunteers to identify ICU infection status. We confirmed that the PA detection ratio in ICU patients was significantly higher than that in healthy volunteers (49.2% vs. 13.6%, P < 0.05). Additionally, we monitored drug-resistant PA in 4 ICU patients by this system. The trends in PA counts accurately reflected various treatment backgrounds such as antibiotic use and mechanical ventilator use. Our results suggest that this RT-qPCR system is beneficial for the early diagnosis and evaluation of appropriate antibacterial treatment and may be a useful tool in combating PA infection.
Introduction
Pseudomonas aeruginosa (PA), a ubiquitous bacterial species found in a wide variety of environmental settings, causes nosocomial infection [Citation1] that can be critical and inflict high mortality [Citation2].
Recently, the prevalence of multidrug-resistant PA (MDRP), i.e. strains that are resistant to antibiotics such as fluoroquinolones, carbapenems, and aminoglycosides, has become a serious problem [Citation3]. Infection with MDRP can limit antibiotic therapy, resulting in inappropriate empirical therapy or delays in initiating appropriate therapy, which can lead to high mortality [Citation4,Citation5]. Thus, in order to select appropriate antibacterial agents for treating PA infection, it is necessary to rapidly and accurately identify PA, including MDRP strains, in clinical specimens.
Generally, bacterial culture methods are the gold standard for detecting PA; however, culture methods have several limitations: (i) they take time (2–3 days) [Citation6]; (ii) they only detect culturable pathogens that have the ability to form colonies [Citation7]; and (iii) they can underestimate the number of pathogens due to selection bias induced by administered antibiotics that are present in human blood [Citation8]. Accordingly, culture methods can produce false-negative results. Culture-negative sepsis is commonly observed in ICU patients: previous studies have shown negative blood cultures in 30% of septic shock patient [Citation9]. It has also been reported that blood cultures from febrile neutropenic patients can identify the pathogens in only 17%–42% of cases [Citation10,Citation11]. Thus, to overcome the problems associated with bacterial culture, we adopted Yakult Intestinal Flora-SCAN (YIF-SCAN®), a highly sensitive and rapid system based on reverse transcription quantitative PCR (RT-qPCR) [Citation12–14]. YIF-SCAN® targets rRNA molecules that exist in bacteria abundantly (approximately 104 copies per actively growing cell), and the sensitivity of RT-qPCR is 100 times higher than that of qPCR assays that target rRNA genes (more than 10 copies/bacterial genome) [Citation12]. Therefore, RT-qPCR is a more sensitive assay for detecting occult bacteria. Also, it requires only 6 h for the quantification of bacteria and detects only live bacteria from specimens [Citation14,Citation15].
In the present study, in order to establish a useful tool to combat PA infection, we developed a new PA quantification system based on 23S rRNA-targeted RT-qPCR. We assessed the performance and potential impact of RT-qPCR on antibiotic therapy administered to ICU patients.
Materials and methods
Ethics statement
Human samples were anonymously coded in accordance with local ethical guidelines (as stipulated by the Declaration of Helsinki). Written informed consent was obtained from the patients and healthy volunteers. This study was approved by the Ethical Review Board of Osaka University (approval number: 09184-5) and Yakult Central Institute (FY2017-025, FY2018-028).
Strains and culture conditions
The bacterial strains used are listed in . Each strain was grown under the conditions listed in Table S1. The colony forming units (CFU) of PA were determined by culturing aerobically on trypticase soy agar (TSA, Beckton Dickinson, Franklin Lakes, NJ, USA) or the newly developed selective medium, NCC agar (Supplemental information), at 37°C for 24 h.
Table 1. Specificity tests with the newly designed primer set.
Development of 23S rRNA gene-targeted primers specific to P. aeruginosa
First, we newly sequenced the 23S rRNA genes of 6 strains of PA and 8 species related to PA. Sequence analysis was performed using a previously described method [Citation16]. Each primer set used in the amplification is described in Table S2. The 23S rRNA gene sequences were deposited in the DNA Data Bank of Japan (DDBJ) nucleotide sequence database under accession numbers listed in Table S3. Next, a multiple alignment of the 6 PA strains and 8 related species was performed with the CLUSTAL_X program [Citation17] using the obtained 23S rRNA gene sequences. After a comparison of the sequences in silico, target sites for PA species-specific detection were identified, and a primer set, s-Pa-F (5′-GTCGTCTTTTAGATGACGAAGTGG-3′) and s-Pa-R (5′-TGGTATCTTCGACCAGCCAGA-3′), was newly constructed. The potential target sites for specific detection were identified (Table S4). The specificity of the designed primer pair was then confirmed by submitting the sequences to the BLAST program of the National Center for Biotechnology Information (NCBI) (http://blast.ncbi.nlm.nih.gov/Blast.cgi).
Total RNA extraction and RT-qPCR
For RNA stabilization, 2 volumes of RNAprotect Bacteria Reagent (QIAGEN, Hilden, Germany) were added to samples. After being kept for 10 min at room temperature, the samples were centrifuged at 13,000 × g for 10 min. The supernatant was discarded, and the pellet was stored at −80°C until used for RNA extraction. RNA extraction and RT-qPCR using a QIAGEN OneStep RT-PCR kit were performed as described elsewhere [Citation12]. In the case of bacterial cultures or blood samples, assays were performed in 96-well optical plates (WATSON, Tokyo, Japan) using a 7500 Real-Time PCR System (Thermo Fisher, Waltham, MA, USA). For fecal samples, 384-well optical plates (Life Technologies, Carlsbad, CA, USA) with a QuantStudioTM 12K Flex Real-Time PCR System (Thermo Fisher) were used. The reaction mixture was incubated at 50°C for 30 min for reverse transcription. In either case, the continuous amplification program consisted of one cycle at 95°C for 15 min and 45 cycles at 94°C for 20 s, 60°C for 20 s, and 72°C for 35 s. Standard curves were generated using the threshold cycle (Cq) values and the corresponding cell counts, which were determined with DAPI staining [Citation18].
DNA extraction and qPCR
Samples were centrifuged at 13,000 × g for 10 min, and the supernatant was discarded. The pellet was stored at −80°C until used for DNA extraction. DNA extraction was performed using a previously described method [Citation19]. qPCR was carried out using a QIAGEN OneStep RT–PCR kit. Each reaction mixture contained the same components as those for RT-qPCR, except for the replacement of template RNA with the same amount of template DNA. The reaction mixture was incubated at 95°C for 15 min and 45 cycles at 94°C for 20 s, 60°C for 20 s, and 72°C for 35 s. The subsequent procedures were the same as those for RT-qPCR.
Determination of primer specificity
Total RNA fractions extracted from the bacterial cells of each strain (shown in ) at a dose corresponding to 105 cells were assessed by RT-qPCR using the primer set of s-Pa-F and s-Pa-R.
Determination of RT-qPCR sensitivity
Total RNA and DNA fractions of the 6 strains of PA were extracted from culture samples, and bacterial counts were determined with DAPI staining. Serial RNA and DNA dilutions corresponding to bacterial counts ranging from 10−2 to 104 cells were assessed by RT-qPCR and qPCR assays, respectively.
Quantification of P. aeruginosa spiked to human blood and human feces by RT-qPCR and culture methods
Commercially available human blood type A (Kohjin Bio Co., Saitama, Japan) and fecal samples collected from three healthy adult volunteers that had been confirmed in advance not to include PA were used. As for fecal samples, weighed samples were suspended in 9 volumes of trypticase soy broth (TSB, Beckton Dickinson). PA ATCC 10145T was serially diluted with TSB and then spiked to make final concentrations ranging from 100 to 104 cells/mL of blood and 102 to 107 cells/g of feces. For RT-qPCR assays, 1 mL of the blood sample and 120 μL (4 mg equivalent feces) of the fecal homogenate was used for RNA extraction. For the culture method, human blood samples (1 mL) or fecal homogenates (100 μL of each appropriate dilution series) spiked with PA were cultured on NCC agar plates.
Monitoring of MDRP exposed to antibiotics by three different methods
MDRP ATCC BAA-2108TM adjusted to a final concentration of 106 CFU/mL was cultured in Dulbecco’s modified Eagle’s medium (DMEM, Thermo Fisher) containing 10% fetal bovine serum (FBS, Thermo Fisher), 1% MEM nonessential amino acids (Thermo Fisher), 5 μg/mL colistin sulfate salt (Sigma-Aldrich Co., St. Louis, MO, USA), and 100 μg/mL doripenem hydrate (SHIONOGI & CO., LTD., Osaka, Japan) at 37°C with shaking (140 rpm). After the start of culture, 10 mL of the culture solution was periodically sampled and washed by centrifugation at 13,000 × g for 10 min, and the supernatant was discarded. The precipitated pellets were resuspended in 10 mL of antibiotic-free and serum-free DMEM. Then, the culture solution was distributed for RNA extraction (1 mL), DNA extraction (1 mL), culturing for CFU counts (1 mL), cell infection (2 mL), and acquisition of fluorescent images (3 mL). Nucleic acid extraction, RT-qPCR, and qPCR were performed as described above. For culturing for CFU counts, 1 mL of the appropriate dilution series was cultured on TSA plates.
Quantification of inflammatory response in infected cells
Caco-2 cells (86010202) were plated on 6-well plates (Costar, Corning, NY, USA) at a density of 3.0 × 105 cells/well and used in the infection assays. For cell infection, 2 mL of antibiotic-treated MDRP suspension was applied to the cells, and cells were incubated at 37°C with 5% CO2 in air for 6 h. The bacterial proinflammatory effect on Caco-2 cells was assessed. After a 6-h incubation, 2 mL of the cell culture medium was sampled and centrifuged at 2,300 × g for 5 min. The supernatant was stored at −80°C until IL-8 production was measured. IL-8 production was assayed using an ELISA Quantikine kit (BioLegend). Total RNA was extracted from infected Caco-2 cells using TRIzol reagent (Thermo Fisher). IL-8 [Citation20] and GAPDH [Citation21] gene expression analyses were performed by RT-qPCR.
Observation and acquisition of fluorescent images
For acquisition of fluorescent images, the bacterial suspension was centrifuged at 13,000 × g for 10 min and then concentrated 100-fold. Fluorescence in situ hybridization (FISH) analyses were performed as described previously with minor modifications [Citation22].
Monitoring of drug-resistant P. aeruginosa in clinical samples and fecal microbiota analysis
For the analysis of clinical fecal samples, we used total RNA collected in a previous study [Citation23], which were stored at −80°C until used in this study. In the previous study, fecal samples were acquired from 65 patients who were more than 16 years old and had been placed on a ventilator within 3 days after admission to the ICU, and who were diagnosed as having sepsis in the Department of Traumatology and Acute Critical Medicine, Osaka University Medical School, and Osaka General Medical Center during the period from November 2011 to September 2016. Also, we collected fecal samples from 44 healthy adults and prepared total RNA. Then, three serial dilutions of each extracted RNA sample were used for RT-qPCR using the primer set of s-Pa-F and s-Pa-R. Furthermore, when monitoring drug-resistant PA in fecal samples of 4 ICU patients by RT-qPCR, drug-resistance gene-specific primers (blaIMP [Citation24], ampC [Citation25], and mexA [Citation26]) were also used. The fecal microbiota compositions of the 4 ICU patients were analyzed using the YIF-SCAN® system as described previously [Citation12].
Statistical analysis
Statistical analyses were performed using IBM SPSS Statistics Desktop version 22.0 software (IBM Japan Ltd.). P values <0.05 were considered statistically significant. Data are presented as mean and standard deviation of representative experiments.
Results
Specificity tests with the newly designed primer set
We evaluated the specificity of the newly designed primer set. Among 39 strains, including 6 strains of PA, 12 species related to PA, and 21 strains of enteric bacteria or causative agents of human intestinal tract infectious disease, the primer set reacted only with the 6 strains of PA and showed no reaction with the other 33 bacterial strains investigated (). This confirmed that the primer set targeting PA is specific for the target strains.
Quantification of P. aeruginosa by RT-qPCR and comparison of sensitivity with qPCR
The counts of PA in pure cultures obtained by DAPI staining and Cq values obtained by RT-qPCR showed a good correlation over the range of RNA amounts corresponding to 10−2–104 bacterial cells/reaction (A–F). The counts of PA also correlated well with the Cq values obtained by qPCR; however, the detection limit was 100 cells/reaction (A–F). These results indicated that RT-qPCR is approximately 100-fold more sensitive than qPCR. The parameters from the linear regression analyses for the 6 different PA strains were highly similar, indicating they had the same quantitative performance (G).
Figure 1. Quantification of P. aeruginosa by RT-qPCR and comparison of sensitivity with qPCR. PA ATCC 10145T (A), ATCC 9027 (B), ATCC 15442 (C), JCM 2776 (D), JCM 5961 (E), and DSM 6195 (F) were cultured separately. RNA and DNA were extracted from cultured samples in the early stationary phase. Bacterial counts were determined microscopically with DAPI staining. Based on bacterial counts, 10-fold serial dilutions of RNA or DNA from 10−2 to 104 cells were assessed. The Cq values obtained were plotted against the log10 number of bacterial cells subjected to each reaction (n = 3). Values are the mean ± SD. (G) The parameters from the linear regression analyses for the 6 different PA strains.
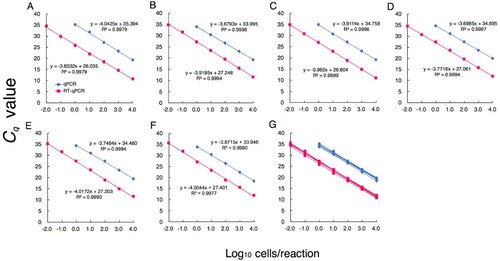
Quantification of P. aeruginosa spiked to human blood or feces using RT-qPCR and culture methods
The spiked bacterial counts could be detected by RT-qPCR at concentrations as low as 100.3 cells/mL of blood and 102.4 cells/g of feces. Also, the bacterial counts detected by the culture method and those determined by RT-qPCR showed a good correlation (A and B).
Figure 2. Quantification of P. aeruginosa spiked to human blood or feces using RT-qPCR and culture methods. Commercially available human peripheral blood (A) and fecal samples collected from 3 individuals (B) were spiked with serial dilutions of PA ATCC 10145T at final concentrations ranging from 100 to 104 cells/mL of blood and 102 to 107 cells/g of feces. The total counts of PA were determined by RT-qPCR and the culture method (n = 4). For the culture method, an appropriate dilution series of each blood sample was cultured on NCC agar plates (selective medium).
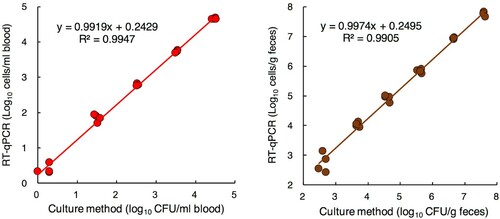
Comparison of detection methods in generating time-kill curves for MDRP exposed to antimicrobials
Changes over time in the counts of antibiotic-treated MDRP differed greatly depending on the measurement method (A). The counts detected with RT-qPCR gradually decreased until 336 h after antibiotic treatment. In contrast, the counts detected with qPCR showed almost no change. The counts detected with the culture method decreased rapidly after antibiotic treatment and were no longer detected 48 h after treatment.
Figure 3. Comparison of detection methods in generating time-kill curves for MDRP exposed to antimicrobials. (A) Time-kill curves for PA ATCC BAA-2108TM exposed to colistin and doripenem combinations (n = 4). Bacterial counts in the culture solutions sampled over time were determined by RT-qPCR, the culture method, and qPCR. For RT-qPCR and qPCR, the primer set targeting PA-specific 23S rRNA was used. For the culture method, the appropriate dilution series were cultured on TSA plates. When the bacterial count was not detected (<1 cell/mL), the circles were rendered as open. (B) Parts of the culture solutions were subjected to measurement of bacterial counts and assayed by FISH with FITC-labeled Eub338. Green and red images of PA were obtained by DAPI and FISH, respectively. Scale bars: 10 μm. (C) After Caco-2 cells were infected with a part of the culture solution used for determination of bacterial counts, relative amounts of IL-8-encoding transcripts were measured by RT-qPCR. Expression was normalized to GAPDH, and the relative gene expression values were compared to those of uninfected cells (n = 4). The production of IL-8 was measured by ELISA (n = 4). The red and green arrows indicate the point at which PA was thought to have disappeared by RT-qPCR and the culture method, respectively. Data indicate the mean ± SD.
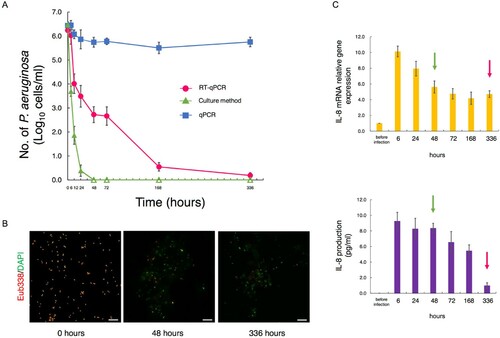
When the PA solution was tested with FISH, a large number of viable bacteria were observed at the start of antibiotic treatment; however, the number of bacteria dramatically decreased, and almost no viable bacteria could be observed after 336 h (B).
PA solutions sampled to measure bacterial counts were also used for infection of Caco-2 cells. The relationship between the inflammatory response and the PA counts obtained with each measurement method was evaluated. When Caco-2 cells were infected with 6-h antibiotic-treated PA, the IL-8 gene expression and production levels in cells increased more than 9-fold compared to before infection. Even at 48 h after antibiotic treatment, when PA was no longer detectable by culturing (C, green arrows), the IL-8 gene expression and secretion levels were very high. At 336 h, the point when no decrease in cell counts was seen with qPCR, but most PA were thought to have disappeared by RT-qPCR (C, red arrows), IL-8 gene expression and production had decreased remarkably. Only the trend in the PA count as monitored by RT-qPCR was similar with the level of Caco-2 cell IL-8 production (C).
Quantification of P. aeruginosa in fecal samples of ICU patients and healthy volunteers
To examine whether RT-qPCR can be applied to clinical samples and to identify the ICU infection status, RT-qPCR analysis was performed using fecal samples collected from 65 ICU patients (). PA was detected 49.2% at an average count of 105.48±1.90 cells/g of feces. In contrast, in 44 healthy volunteer samples, 13.6% were positive for PA and the average count was 104.38±1.21 cells/g of feces (). The detection ratio in ICU patients was significantly higher than that in healthy volunteers (P < 0.05). As for bacterial counts, while some patients showed high levels of PA in the feces (108 cells/g of feces), some patients had very low levels (102 cells/g of feces) as observed in healthy volunteers.
Table 2. Quantification of P. aeruginosa in fecal samples of ICU patients and healthy volunteers.
Monitoring of drug-resistant P. aeruginosa in clinical samples
When PA counts in the feces of ICU patients undergoing antibiotic treatment were measured with RT-qPCR, we could monitor abnormal proliferation and excessive decreases of PA in the intestines (A–D). Monitoring of drug-resistant PA was also possible with the combined use of drug-resistance gene amplification primers and the PA-specific primers (A–D). In the 4 patients in whom PA was detected from the feces, a drug efflux pump gene (mexA) and the AmpC β-lactamase gene (ampC) were detected from the feces of all patients (A–D). From Patient B, who was receiving meropenem (a carbapenem antibiotic), a metallo-beta-lactamase (MBL)-encoding gene (blaIMP) was detected (B).
Figure 4. Monitoring of drug-resistant P. aeruginosa by RT-qPCR and clinical treatment. RT-qPCR quantification of PA (brown) and simultaneous detection of drug resistance genes (blaIMP, ampC, mexA) using drug-resistance gene amplification primers (+, red-square; −, white-square) in fecal samples from 4 ICU patients (A–D). The abscissa of each graph represents the days of hospital stay. The microbiota composition was analyzed using the YIF-SCAN® version of a 16S and 23S rRNA-targeted RT-qPCR system. The size of the pie charts shows the total bacterial counts (circles indicate 1011, 1010, 109, 108, and 107 cells/g feces in this order from the outside to the inside), and the colour of the pie charts shows the percentage of obligate anaerobes (black) and facultative anaerobes and aerobes (light gray). The duration of ventilator use is indicated by a broken black line, and the duration of antibiotic use during the hospital stay is indicated by the various coloured solid lines. (E) Association between PA counts and fecal occupation rate of representative microorganisms. Spearman's rank correlation coefficient was applied to the dataset. *P < 0.05, **P < 0.01, ***P < 0.001. SBT/ABPC, sulbactam/ampicillin; MCFG, micafungin; MEPM, meropenem; LZD, linezolid; VCM, vancomycin; LVFX, levofloxacin; CEZ, cefazolin; CAZ, ceftazidime; CFPM, cefepime; L-AMB, amphotericin B.
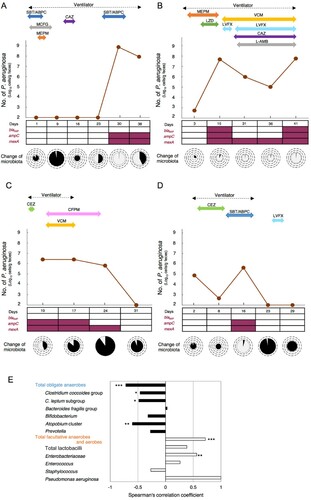
The trends in PA counts in the feces of ICU patients monitored by RT-qPCR were related to the administration of antibiotics, the use of an artificial ventilator, and the composition of intestinal microbiota ( and Table S5). In Patient A, explosive proliferation of PA in the intestines was induced at the time (Day 30) when obligate anaerobe groups rapidly decreased with the administration of antibiotics (A). Similar trends were seen in Patient B (Day 15, 41) and Patient D (Day 16) (B and D). In contrast, when the administration of antibiotics was ceased, the PA count decreased (Patient A: Day 38, Patient C: Day 31, Patient D: Day 23) (A, C and D). In two patients taken off of artificial ventilators, intestinal counts of drug-resistant PA decreased to below the detection limit at the time when the counts of the most prevalent obligate anaerobes recovered after ventilator withdrawal (Patient C: Day 31, D: Day 23) (C and D).
Negative correlations were seen between the PA counts and the proportion of total obligate anaerobes (E). In contrast, positive correlations were seen between PA counts and the proportions of total facultative anaerobes and aerobes (E).
Discussion
In this study, we developed a new PA quantification system based on 23S rRNA-targeted RT-qPCR. Using the RT-qPCR method, PA was detected from as little as 1 cell/mL of blood and 102 cells/g of feces, with high specificity and quantifiability. When this RT-qPCR method was applied to fecal samples from ICU patients, it demonstrated that PA had colonized some patients at very low levels in the intestine (102 cells/g of feces). RT-qPCR was shown to be useful for accurately determining the infection status or screening of patients who carry minute amounts of PA, which are easy to miss with conventional methods.
The results of microorganism tests such as culturing and qPCR and patients’ clinical conditions do not necessarily coincide [Citation9–11]. In our experimental system using PA-infected cells, the counts of antibiotic-treated PA by RT-qPCR correlated well with the inflammatory response of infected cells. As RT-qPCR targets rRNA that is rapidly degraded in dead cells [Citation12], it can measure only live bacteria that are highly associated with infectivity, inflammation induction, and pathogenicity. In contrast, qPCR targets DNA that continues to remain even in dead bacteria. By DAPI staining, which targets DNA, we observed that fluorescence microscopy images remained fairly constant even at the death phase, so dead PA continued to be observed after antibiotic treatment (B). Given this, the high number of PA detected by qPCR, despite the significant reduction in the inflammatory response of the cells, is thought to be due to the detection of both viable and dead cells. The discrepancy between bacterial count results with culture methods and the inflammatory response of infected cells is thought to be due mainly to a decrease in the ability of PA to form colonies in a viable but non-culturable state [Citation7] under antibiotic treatment. From the above, since RT-qPCR can accurately detect PA even during the use of antibiotics, RT-qPCR is considered to be very useful in choosing appropriate antibiotics and determining the timing of treatment.
Previous reports have indicated that more than 70% of ICU patients receive antimicrobial treatment and are therefore susceptible to the development of drug-resistant PA infection [Citation27]. In this study, we demonstrated that intestinal infections of drug-resistant PA in ICU patients can be quickly detected using RT-qPCR. It has been reported that meropenem is associated with the risk of resistance emergence [Citation28]. In Patient B, who had received meropenem for more than 2 weeks, an elevated fecal PA count was observed, and upregulation of the MBL-encoding gene was detected. About 80% of MDRP have been reported to be MBL-positive isolates [Citation29]. Furthermore, PA can actually proliferate in the intestines during the administration of levofloxacin [Citation30]. Therefore, there is a high likelihood that the bacterium detected in the feces was MDRP. This indicates that the RT-qPCR method may also be applicable for rapid and highly sensitive monitoring of such MDRP.
The PA counts with RT-qPCR closely reflected the treatment background of ICU patients. Specifically, the induction of abnormal proliferation of PA in the intestines was observed with the long-term use of various types of antibiotics. It has been shown that the eradication of PA is actually facilitated by discontinuing the administration of sulbactam/ampicillin, which is not active against PA [Citation31], or broad spectrum cephem antibiotics (cefepime), for which drug-resistant PA has low sensitivity [Citation32]. The eradication of PA in the intestines was also observed after the withdrawal of mechanical ventilator use. Thus, we demonstrated that the timely monitoring of fecal PA accompanying medical interventions is possible with this system.
In this study, abnormal proliferation of PA in the intestines occurred in ICU patients with decreased obligate anaerobes in the intestines after antibiotic administration, and a negative correlation was observed between obligate anaerobe counts and PA. In ICU patients, disruption of the gut microbiota has been reported to be a high-risk factor for carbapenem-resistant PA acquisition [Citation33] and mortality [Citation34]. Also, Robak et al. showed that microbiota-dependent IgA production is required for antibacterial immunity during acute bacterial pneumonia [Citation35]. These findings indicate the importance of intestinal microbiota in ICU patients. Since both intestinal dysbiosis and PA infection dynamics can be understood simultaneously, the RT-qPCR method may be a very effective means of controlling the risk of infection in patients.
Our study has several limitations. First, while we attempted to monitor drug-resistant PA using 3 drug-resistance gene primers, PA has various drug-resistance mechanisms. In the future, we anticipate that definitive diagnosis and monitoring of MDRP will also be possible by generating other kinds of resistance gene detection primers. Second, horizontal transfer of the blaIMP gene has been reported; thus, the possibility that other carbapenem-resistant pathogens such as Enterobacteriaceae or Acinetobacter baumannii had the blaIMP gene cannot be ruled out. It will also be possible to estimate gene origins by preparing other pathogen-specific primers and monitoring these pathogens. Third, in the monitoring of drug-resistant PA in the feces of ICU patients, the sample size was small. Further studies with larger sample sizes are necessary to clarify the relationship between the fluctuations of PA detected by RT-qPCR and the clinical course. Fourth, although this new method enables very sensitive detection compared to the conventional method, it still cannot quantify the amount of PA below the detection limit (∼102 cells/g) for feces.
In summary, we demonstrated the potential of a new RT-qPCR method as an effective tool for controlling nosocomial infections of PA as well as for judging the efficacy of antibiotic treatment and determining whether to continue or withdraw other medical actions.
20210317_Supplemental-Material_TEMI-2021-0107-R1_editable.docx
Download MS Word (45.3 KB)Disclosure statement
No potential conflict of interest was reported by the author(s).
Acknowledgments
We thank Yukihiro Yokoyama, MD for critical reading of the manuscript. We also thank Hirotaka Haibara, Toshihiko Takada, and Ryoko Iizuka for assistance with cell culture, FISH analyses, and cytokine assays, respectively.
Data availability
The 23S rRNA gene sequences deposited in the DDBJ (http://www.ddbj.nig.ac.jp/) nucleotide sequence database are listed in Table S3.
References
- Gellatly SL, Hancock REW. Pseudomonas aeruginosa: new insights into pathogenesis and host defenses. Pathog Dis. 2013;67:159–173.
- Kim HS, Park BK, Kim SK, et al. Clinical characteristics and outcomes of Pseudomonas aeruginosa bacteremia in febrile neutropenic children and adolescents with the impact of antibiotic resistance: a retrospective study. BMC Infect Dis. 2017;17:500.
- Horcajada JP, Montero M, Oliver A, et al. Epidemiology and treatment of multidrug-resistant and extensively drug-resistant Pseudomonas aeruginosa infections. Clin Microbiol Rev. 2019;32:e00031–19.
- Martinez-Nadal G, Puerta-Alcalde P, Gudiol C, et al. Inappropriate empirical antibiotic treatment in high-risk neutropenic patients with bacteremia in the Era of multidrug resistance. Clin Infect Dis. 2020;70:1068–1074.
- Zhang Y, Chen XL, Huang AW, et al. Mortality attributable to carbapenem-resistant Pseudomonas aeruginosa bacteremia: a meta-analysis of cohort studies. Emerg Microbes Infect. 2016;5:e27.
- Loonen AJM, Wolffs PFG, Bruggeman CA, et al. Developments for improved diagnosis of bacterial bloodstream infections. Eur J Clin Microbiol Infect Dis. 2014;33(10):1687–1702.
- Li L, Mendis N, Trigui H, et al. The importance of the viable but non-culturable state in human bacterial pathogens. Front Microbiol. 2014;5:258.
- Scheer CS, Fuchs C, Gründling M, et al. Impact of antibiotic administration on blood culture positivity at the beginning of sepsis: a prospective clinical cohort study. Clin Microbiol Infect. 2019;25:326–331.
- Kethireddy S, Bilgili B, Sees A, et al. Culture-negative Septic Shock compared With culture-positive Septic Shock. Crit Care Med. 2018;46:506–512.
- Sakaguchi S, Saito M, Tsuji H, et al. Bacterial rRNA-targeted reverse transcription-PCR used to identify pathogens responsible for fever with neutropenia. J Clin Microbiol. 2010;48:1624–1628.
- Van de Wyngaert Z, Berthon C, Debarri H, et al. Discontinuation of antimicrobial therapy in adult neutropenic haematology patients: A prospective cohort. Int J Antimicrob Agents. 2019;53:781–788.
- Matsuda K, Tsuji H, Asahara T, et al. Sensitive quantitative detection of commensal bacteria by rRNA-targeted reverse transcription-PCR. Appl Environ Microbiol. 2007;73:32–39.
- Nishigaki E, Abe T, Yokoyama Y, et al. The detection of intraoperative bacterial translocation in the mesenteric lymph nodes is useful in predicting patients at high risk for postoperative infectious complications after esophagectomy. Ann Surg. 2014;259:477–484.
- Yokoyama Y, Fukaya M, Mizuno T, et al. Clinical importance of “occult-bacterial translocation” in patients undergoing highly invasive gastrointestinal surgery: A review. Surg Today. 2020 Aug 28. Epub ahead of print. PMID: 32857253.
- Kurakawa T, Kubota H, Tsuji H, et al. Development of a sensitive rRNA-targeted reverse transcription-quantitative polymerase chain reaction for detection of vibrio cholerae/mimicus, V. parahaemolyticus/alginolyticus and campylobacter jejuni/coli. Microbiol Immunol. 2012;56:10–20.
- Sato T, Matsumoto K, Okumura T, et al. Isolation of lactate-utilizing butyrate-producing bacteria from human feces and in vivo administration of Anaerostipes caccae strain L2 and galacto-oligosaccharides in a rat model. FEMS Microbiol Ecol. 2008;66:528–536.
- Thompson JD, Gibson TJ, Plewniak F, et al. The CLUSTAL_X windows interface: flexible strategies for multiple sequence alignment aided by quality analysis tools. Nucleic Acids Res. 1997;25:4876–4882.
- Jansen GJ, Wildeboer-Veloo AC, Tonk RH, et al. Development and validation of an automated, microscopy-based method for enumeration of groups of intestinal bacteria. J Microbiol Methods. 1999;37:215–221.
- Matsuki T, Watanabe K, Fujimoto J, et al. Quantitative PCR with 16S rRNA-gene-targeted species-specific primers for analysis of human intestinal bifidobacteria. Appl Environ Microbiol. 2004;70:167–173.
- Jiang M, Zhang F, Wan C, et al. Evaluation of probiotic properties of Lactobacillus plantarum WLPL04 isolated from human breast milk. J Dairy Sci. 2016;99:1736–1746.
- Giribaldi G, Prato M, Ulliers D, et al. Involvement of inflammatory chemokines in survival of human monocytes fed with malarial pigment. Infect Immun. 2010;78:4912–4921.
- Takada T, Matsumoto K, Nomoto K. Development of multi-color FISH method for analysis of seven Bifidobacterium species in human feces. J Microbiol Methods. 2004;58:413–421.
- Shimizu K, Yamada T, Ogura H, et al. Synbiotics modulate gut microbiota and reduce enteritis and ventilator-associated pneumonia in patients with sepsis: a randomized controlled trial. Crit Care. 2018;22:239.
- Poirel L, Walsh TR, Cuvillier V, et al. Multiplex PCR for detection of acquired carbapenemase genes. Diagn Microbiol Infect Dis. 2011;70:119–123.
- Juan C, Moyá B, Pérez JL, et al. Stepwise upregulation of the Pseudomonas aeruginosa chromosomal cephalosporinase conferring high-level β-lactam resistance involves three AmpD homologues. Antimicrob Agents Chemother. 2006;50:1780–1787.
- Shu JC, Chia JH, Siu LK, et al. Interplay between mutational and horizontally acquired resistance mechanisms and its association with carbapenem resistance amongst extensively drug-resistant Pseudomonas aeruginosa (XDR-PA). Int J Antimicrob Agents. 2012;39:217–222.
- Li Y, Xia X, Li X, et al. Correlation between the use of antibiotics and development of a resistant bacterial infection in patients in the ICU. Biosci Trends. 2018;12:517–519.
- Ong DS, Jongerden IP, Buiting AG, et al. Antibiotic exposure and resistance development in Pseudomonas aeruginosa and Enterobacter species in intensive care units. Crit Care Med. 2011;39:2458–2463.
- Kouda S, Ohara M, Onodera M, et al. Increased prevalence and clonal dissemination of multidrug-resistant Pseudomonas aeruginosa with the blaIMP-1 gene cassette in Hiroshima. J Antimicrob Chemother. 2009;64:46–51.
- North DS, Fish DN, Redington JJ. Levofloxacin, a sond-generation fluoroquinolone. Pharmacotherapy. 1998;18:915–935.
- Campoli-Richards DM, Brogden RN. Sulbactam/ampicillin. A review of its antibacterial activity, pharmacokinetic properties, and therapeutic use. Drugs. 1987;33:577–609.
- Bassetti M, Vena A, Croxatto A, et al. How to manage Pseudomonas aeruginosa infections. Drugs Context. 2018;7:212527.
- Pettigrew MM, Gent JF, Kong Y, et al. Gastrointestinal microbiota disruption and risk of colonization with carbapenem-resistant Pseudomonas aeruginosa in intensive care unit patients. Clin Infect Dis. 2019;69:604–613.
- Shimizu K, Ogura H, Asahara T, et al. Probiotic/synbiotic therapy for treating critically ill patients from a gut microbiota perspective. Dig Dis Sci. 2013;58:23–32.
- Robak OH, Heimesaat MM, Kruglov AA, et al. Antibiotic treatment–induced secondary IgA deficiency enhances susceptibility to Pseudomonas aeruginosa pneumonia. J Clin Invest. 2018;128:3535–3545.