ABSTRACT
Chronic hepatitis B virus (HBV) infection due to perinatal mother-to-infant transmission (MTIT) remains a serious global public health problem. It has been shown that intrauterine exposure to HBV antigens might account for the MTIT-related chronic infection. However, whether hepatitis B surface antigen (HBsAg) intrauterine exposure affected the offspring’s immune response against HBV and MTIT of HBV has not been fully clarified. In this study, we investigated the effects and the potential mechanisms of the HBsAg intrauterine exposure on the persistence of HBV replication using a solely HBsAg intrauterine exposure mice model. Our results revealed that solely HBsAg intrauterine exposure significantly accelerated the clearance of HBV when these mice were hydrodynamically injected with pBB4.5-HBV1.2 plasmids after birth, which may be due to the increased number of HBs-specific CD8+ T cells and interferon-gamma secretion in the liver of mice. Mechanismly, HBsAg intrauterine exposure activated antigen-presenting dendritic cells, which led to the generation of antigen-specific cellular immunological memory. Our data provide an important experimental evidence for the activation of neonatal immune response by HBsAg intrauterine exposure.
Introduction
Chronic hepatitis B virus (HBV) infection remains a serious global public health problem. Currently, about 257 million people are chronically infected with HBV worldwide, resulting in approximately 1 million deaths every year [Citation1]. In most HBV endemic regions, the main transmission route of HBV is perinatal mother-to-infant transmission (MTIT), by which the chronic HBV carriers contract the virus via vertical transmission from their infected mother early in life. In contrast to horizontal transmission between adults which usually leads to self-limited acute infection, more than 90% of perinatal infections due to MTIT may turn out to be chronic infections [Citation2]. It has been suggested that intrauterine exposure of the fetal immune system to HBV antigens and/or the immature immune system of young children might account for the MTIT-related chronic infection [Citation3–5]. However, the mechanism of MTIT leading to HBV persistent infection has not been fully clarified.
A large number of studies have proved that the risk of vertical transmission is significantly higher in HBeAg-positive mothers than that in HBeAg-negative mothers if without antiviral therapy and immunoprophylaxis [Citation6,Citation7]. This phenomenon is usually attributed to the immune suppression of mother-derived HBeAg and the high level of viremia in HBeAg-positive mothers [Citation8,Citation9]. However, with the increasingly wide use of nucleos(t)ide analogues (NAs) for the treatment of chronic hepatitis B (CHB) patients, more patients achieved HBeAg seroconversion as well as low HBV DNA load, so that the number of female patients of childbearing age who are solely HBsAg positive is increasing. In addition to the lower HBV DNA load in HBeAg-negative mothers, it is not clear whether maternal-derived HBsAg could affect the fetal immune system thus affecting the risk of MTIT-related HBV infection. Therefore, exploring the role and mechanism of HBsAg in the vertical transmission is of great significance for the implementation of MTIT blockade strategies for the neonates born to HBeAg-negative mothers whose serum HBsAg was positive, and it might provide the potential immunotherapeutic targets for the treatment of persistent HBV infection.
HBsAg is the envelope protein of HBV and the important marker of HBV infection. During HBV infection, excess HBsAg can also be assembled into non-infectious subviral particles (SVP), which are much more abundant in serum than complete hepatitis B virion in CHB patients. Although previous studies have suggested that HBsAg theoretically cannot pass through the placental barrier, accumulated studies have reported that maternal HBsAg can be detected in placenta, cord blood or amniotic fluid when HBV infects maternal cells in the placenta, or due to the placental leakage [Citation10–14]. These findings suggested that the maternal-derived HBsAg might also alter the immune response against HBV in offspring. Indeed, poor vaccination effects and more chronic HBV infection in offspring have been observed after intrauterine exposure to HBsAg [Citation15–17]. However, there is no statistical difference in anti-HBs titre and response rate between the offspring born to women with HBsAg positive alone and those without HBV infection, suggesting that the immune response to the vaccine in offspring may not be inhibited by HBsAg [Citation18]. Furthermore, a recent study showed that intrauterine exposure to HBsAg may trigger a state of “trained immunity” in infants, which is manifested by promoted innate immune maturation and T-helper 1 cells (Th1) development [Citation19]. These findings raise the possibility that intrauterine exposure to HBsAg may promote the immune response of offspring. However, the effects and potential mechanisms of the HBsAg intrauterine exposure on the persistence of HBV replication and immune response of offspring still need to be clarified.
In this study, we constructed a mouse model of solely HBsAg intrauterine exposure using the HBV transgenic maternal mouse which only HBsAg is positive in its serum and liver tissue and investigated the effects of HBsAg intrauterine exposure on the persistence of HBV replication and immune response of the offspring when re-exposed to HBV after birth. We found that only HBsAg intrauterine exposure significantly increased the number of HBs-specific CD8+ T cells and interferon-gamma (IFN-γ) secretion in the liver of mice, which may account for the faster clearance of HBV when the HBsAg intrauterine exposure mice were re-exposed to HBV after birth. Mechanismly, HBsAg intrauterine exposure activated the powerful antigen-presenting dendritic cells (DCs), which led to the generation of antigen-specific cellular immunological memory. Our data provide an important experimental evidence for the activation of neonatal immune response by HBsAg intrauterine exposure.
Materials and methods
Experimental animals and model construction
C57BL/6 mice and C57BL/6-Tg (HBV Alb-1) Bri44 HBV transgenic female mice were purchased from Peking University Health Science Center. All of the mice had the C57BL/6N genetic background. The C57BL/6-Tg (HBV Alb-1) Bri44 HBV transgenic mouse is a widely used HBV transgenic mouse model, which integrated 2839nt to 1986nt of HBV subtype ayw (containing the coding regions only for the HBV pre-S, S and X antigens) immediately downstream of the mouse Alb promoter so that only HBsAg is positive in its serum and liver tissue [Citation20]. We identified the transgenic mice by PCR genotyping using the following primer: 5′- AGGCGGGGTTTTTCTTGTTG -3′ and 5′- GGAAAGCCCTACGAACCACT -3′. This PCR reaction resulted in a product of 515 bp for HBV transgenic mice. The PCR of nicotinamide nucleotide transhydrogenase (Nnt) allele was used for the identification of mouse substrain background as described [Citation21]. The experiments were conducted in accordance with the Guide for the Care and Use of Laboratory Animals and were approved by the animal ethics committee of Peking University Health Science Center (Beijing, China).
Plasmid and HBV hydrodynamic injection (HI)
The replication cloning plasmid pBB4.5-HBV1.2, which carries 1.2 times the length of genotype C HBV genome, was kept in our laboratory and used for the HI. The HI was performed as previously described [Citation22]. Two mice of each group were sacrificed at 72 h post-injection, and the liver was taken for immunohistochemistry staining (IHC) to prove the plasmid expressed successfully. Serum was collected for the analysis of HBsAg and HBeAg levels at 7 days post-injection. Mice with comparable HBsAg and HBeAg levels were used in the studies.
Vaccination and immunization strategy
For vaccine immunization and HBsAg stimulation, 4-week-old mice were subcutaneously treated with a 3 µg recombinant hepatitis B vaccine (Kangtai Biological Products Ltd, Shenzhen, China).
HBV serological assays by time-resolved fluoroimmunoassay (TRFIA)
Serum of mice in each group was collected at 1, 2, 3, 4, 6, 8, 10, 12, 16, 20, 24 and 28 weeks after HI and per week after vaccination. The levels of HBsAg, HBeAg and anti-HBs were detected by the TRFIA diagnostic kit according to the manufacturer’s instructions (PerkinElmer, Waltham, MA).
IHC for HBsAg and hepatitis B core antigen (HBcAg)
At 12 weeks after HI, 4 mice in each group were sacrificed and the liver tissues were collected. The expression of HBsAg and HBcAg were detected by IHC using our previous procedures [Citation23]. Mouse anti-HBs antibody and rabbit anti-HBc antibody were purchased from Maixin Biotechnology Development Ltd. (Fuzhou, China).
Isolation of murine splenic and hepatic mononuclear cells
The mouse liver was perfused with pre-warmed PBS via the hepatic portal vein. The liver and spleen tissues were isolated and forced through a 70 µm nylon cell strainer (BD Falcon, Franklin Lakes, NJ) with a syringe plunger. Cells were centrifuged at 500 × g for 5 min at 4°C. Liver mononuclear cells were then layered on 80% percoll solution (GE healthcare, Waukesha, WI) in 40% percoll solution, and gradient centrifuged at 780 × g for 20 min at 4°C. Cells were incubated with the red blood cell lysis buffer (BD Biosciences, San Jose, CA) at room temperature for 10 min, and washed twice in PBS. Cell count and viability detection were performed using a haemocytometer and trypan blue exclusion microscopy.
Analysis of HBs-specific CD8+ T cells
Mononuclear cells isolated from mice liver or spleen were stimulated for 8 h with Kb-restricted HBsAg-derived CD8+ epitope 190–197 (VWLSVIWM) and detected using soluble DimerX H-2 Kb: Ig fusion protein labelled with PE-conjugated rat anti-mouse IgG1 (BD Biosciences) following the previous procedure [Citation24].
Flow cytometry analysis and sorting
Cell staining was performed using anti-mouse CD3e PerCP-Cyanine5.5, anti-mouse CD8a FITC, anti-mouse CD4 RedFluor710, anti-mouse CD279 (PD-1) EFLUOR 450, anti-mouse CD62L APC-EFLUOR, anti-human/mouse CD44 APC, anti-mouse CD11C PE-Cyanine5.5, anti-mouse I-Ab PerCP-Cyanine5.5, anti-mouse CD301 (MGL1/MGL2) APC and anti-mouse CD86 APC/Fire 750. All antibodies were purchased from eBioscience (San Diego, CA) and BioLegend (San Diego, CA). Cells were incubated with Fc Block (BD Biosciences) for 30 min at 4°C in darkness to block the FcγR and then stained with fluorochrome-conjugated antibodies for 60 min at 4°C also in darkness. Data were acquired on Gallios flow cytometers (Beckman Coulter, Brea, CA) or FACS Aria II Sorp cell sorter (BD biosciences) from 1 × 106 cells per sample. The HBs-specific CD8+ T cells and CD11c+ cells were sorted out on BD FACS Aria II sorter and analysed using FlowJo software (Tree Star, Ashland, OR).
RNA extraction and quantitative real-time PCR analysis
The whole blood, fetal mouse liver tissue and cells sorted by FACS were collected and homogenized in TRIzol reagent (Invitrogen, Carlsbad, CA), and RNA was extracted according to the manufacturer’s protocol. The RNA was reverse transcribed into cDNA using Transcriptor First Strand cDNA Synthesis Kit (Roche, Basel, Switzerland) in accordance with the manufacturer’s instructions. Real-time quantitative PCR (RT-qPCR) was performed using the Roche LightCycler 480 sequence detection system (Roche, Mannheim, USA). The primers used for RT-qPCR are shown in Supplementary Table S1.
ELISPOT assay
Mouse IFN-γ precoated ELISPOT kit was purchased from DAKEWE (Shenzhen, China) and used according to the manufacturer’s instructions. Lymphocytes separated from the liver and spleen were tested in triplicate stimulated with 5 µg/mL HBsAg-derived CD8+ epitope peptides. After 36 h of substrate incubation and post-culture operation, the number of IFN-γ-producing cells and spot intensity were estimated using the automated ELISPOT plate reader (AID GmbH, Germany).
RNA sequencing
Total RNA was extracted from fetal mice liver tissue according to the standard method and three biological repeats were performed for each group. The RNA quality assessment, library preparation and sequencing were using the Illumina Novaseq 6000 platform of Majorbio Biotech (Shanghai, China). The Smart-seq of 300–1000 HBs-specific CD8+ T cells and CD11c+ cells sorted from mice livers respectively were completed by using Clontech-SMART-SeqTM v4 UltraTM Low Input RNA Kit on Illumina Hiseq4000 platform of Majorbio Biotech. Genes with corrected P values less than .05 and the absolute value of log2FC > 1 were assigned as significantly differentially expressed genes (DEGs). The Gene Ontology (GO) terms enrichment and pathway annotation using the Kyoto Encyclopedia of Genes and Genomes (KEGG) were analysed and the false discovery rate (FDR) was used to test for the statistical enrichment.
Statistical analyses
The statistical analysis was performed using GraphPad Prism V5.0 (GraphPad Software Inc., San Diego, CA) and SPSS 21.0 (Xishu Software Company, Shanghai, China). Data were presented as the mean ± SEM, and statistical differences were analysed by 2-tailed Student’s t test, Mann–Whitney U test and qualitative data using χ2 test. The P values <.05 were considered statistically significant.
Results
Intrauterine exposure of HBsAg facilitates the rapid elimination of HBV in the mouse pups
To obtain the HBsAg intrauterine exposed wild-type mice (EXP-WT group), C57BL/6-Tg (HBV Alb-1) Bri44 HBV transgenic female mice were crossed with wild-type C57BL/6 male mice, and the offspring with negative integrated HBV DNA and HBsAg were classified as EXP-WT group. The offspring produced by wild-type C57BL/6 mice were used as the control group (WT group) ((A)). To establish the HBV re-exposed models, we designed the following experimental procedure ((B)). Briefly, both EXP-WT and WT mice were injected with pBB4.5-HBV1.2 plasmid by HI to simulate the HBV re-exposure at 6-weeks after birth. On the third day after injection, two mice of each group were randomly selected and sacrificed to examine whether pBB4.5-HBV1.2 plasmid was successfully expressed in the liver by detecting HBcAg expression using IHC assays (Figure S1A). One week after injection, all mice were bled from the angular vein, and serum HBsAg and HBeAg levels were detected to confirm both groups of mice were successfully re-exposed to HBV (Figure S1B and S1C).
Figure 1. HBV in HBsAg intrauterine exposure group was cleared faster than that in the WT group. (A) Procedures for the generation of experimental mice. (B) Schematic diagram of the experimental process. (C) and (D) Dynamic changes of serum HBsAg and HBeAg levels in the EXP-WT group (n = 18) and WT group (n = 24) within 28 weeks post pBB4.5-HBV1.2 injection. (E) IHC of HBsAg and HBcAg in EXP-WT group (left panel) and WT group (right panel) at 12 weeks after HI. (F) Dynamic changes of serum anti-HBs levels in the EXP-WT group and WT group. *P < .05; **P < .01; ***P < .001.
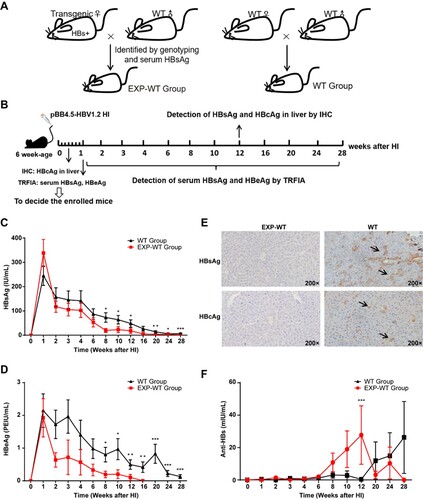
To compare the HBV clearance rate in each group, dynamic changes in serum HBsAg and HBeAg were detected at different time points in the 28-week period after injection. As shown in (C,D), a rapid decrease of both HBsAg and HBeAg levels were observed in each group post-injection. However, the rate of serum HBsAg loss at the 12th and 28th week after HI in the EXP-WT group was significantly higher than that in the WT group (27.78% vs. 4.17%, P = .030; 81.25% vs. 16.67%, P = .0017). Consistent with the trend of HBsAg loss, the clearance of serum HBeAg was also much faster in the EXP-WT group, as compared to that in the WT group ((D)). Serum HBeAg in all EXP-WT group mice became undetectable at 16 weeks after injection, while it was still detectable in 4 of 18 (22.22%) WT mice until the study endpoint at 28 weeks post-injection. Furthermore, the IHC results showed that staining of HBsAg in liver tissue of all EXP-WT mice became negative at the 12th week after HI, while it was still positive in all WT mice pups ((E)). Also, the anti-HBs titre in serum was detected after HBV re-challenge in the mouse pups. Consistent with our expectations, the anti-HBs level in the EXP-WT group was higher than that of the WT mice, and the anti-HBs production time was also earlier in the EXP-WT mice, which indicated that intrauterine exposure may affect the immunity of offspring and lead to a rapid clearance of HBV ((F)).
Previous studies have shown that gender would affect the outcome of HBV infection [Citation24]. Concordantly, we found that the overall HBsAg, HBeAg and anti-HBs levels were lower and declined much faster in female mice, as compared to that in the male mice (Figure S2). Moreover, in comparison to that of the WT mice, faster clearance of HBsAg and HBeAg levels in EXP-WT mice can still be observed either in male or female genders (Figure S3, Tables S2 and S3). Taken together, these results suggested that intrauterine exposure to HBsAg facilitated the rapid elimination of HBV in the mice.
Intrauterine exposure of HBsAg induces more HBs-specific CD8+ T cells in the mouse pups
It is known that HBV-specific T cells play a crucial role in the resolution of HBV infection. To clarify the possible mechanism relevant to the accelerated HBV clearance observed in HBsAg intrauterine exposed progeny mice, mononuclear cells were isolated from the liver and spleen tissues in both groups of 4-week-old mice and analysed by flow cytometry. The flow cytometry gating strategy was presented in Figure S4A. The results showed that EXP-WT mice contained a higher percentage of HBs-specific CD8+ T cells than WT mice in spleen lymphocytes (Figure S5). In addition, after 7 days of HBsAg stimulation, the proportion of HBs-specific CD8+ T cells in the liver of EXP-WT mice was significantly higher than that in WT mice, in both females (2.05% vs. 0.86%, P = .016) and males (1.77% vs. 0.74%, P < .001) ((A–C)).
Figure 2. The HBs-specific CD8+ T cells in the liver of EXP-WT mice were significantly more than those in WT group. (A) HBs-specific CD8+ T cells isolated from the liver of WT mice and EXP-WT mice were analysed by flow cytometry. (B) and (C) Analysis of HBs-specific CD8+ T cells percentage (left) and cell counts (right) in liver of female mice (B, n = 8) and male mice (C, n = 8) in two groups. (D) The HBs-specific PD-1+ CD8+ T cells in the liver of two groups analysed by flow cytometry. (E) Comparation of PD-1 positive HBs-specific CD8+ T cells in the liver of two group mice. Left, female (n = 5); right, male (n = 5). *P < .05; **P < .01; ***P < .001.
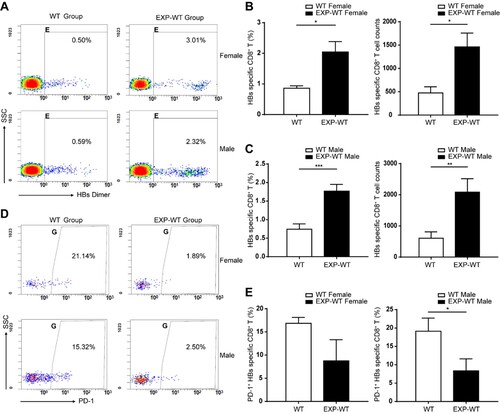
PD-1 is an important negative immunoregulatory molecule, which can promote the apoptosis and death of antigen-specific T cells [Citation25]. We found that EXP-WT mice showed a lower percentage of HBs-specific CD8+ T cells with highly expressed PD-1 than WT mice ((D)), whether male (8.35% vs. 19.13%, P = .036) or female (8.76% vs. 16.85%, P = .055) ((E)). Taken together, these results suggested that more functional HBs-specific CD8+ T cells in the liver were recruited in EXP-WT mice than in WT mice.
HBs-specific CTLs of HBsAg intrauterine exposure mice have a stronger cytotoxic function
To investigate the potential function of HBs-specific CD8+ T cells in EXP-WT mice, Smart-Seq was used to detect the transcriptome of intrahepatic HBs-specific CD8+ T cells sorted by FACS. A total of 2384 DEGs were identified between two groups of mice ((A)). Among these genes, a large number of genes related to cell proliferation and differentiation, as well as immune-related genes, were significantly up-regulated in EXP-WT mice. These differentially expressed immune-related genes include 122 up-regulated and 27 down-regulated genes ((B)). Further GO analysis and KEGG pathway analysis revealed that most of these immune-related genes were significantly enriched in “lymphocyte activation” and “cytokine-cytokine receptor interaction pathway,” which might contribute to stronger HBs-specific CD8+ T immune response ((C,D)). Furthermore, the expression of Il12a, Cd44, Il2rb, Eomes, Tgfb, Gzmb, Ifna, Tlr8 and Irf4, which were related to the antiviral function of T cells, were expressed significantly higher in the EXP-WT group (Figure S6). In consist with the results of the Smart-Seq, RT-qPCR assay was also used to confirm the upregulated expression of these genes (Figure S7A).
Figure 3. Immune-related genes expression in HBs-specific CD8+ T cells was significantly up-regulated in the liver of EXP-WT mice. (A) The volcano map of differentially expressed genes (log2FC > 1 and P-adjust <.05 as significant difference) detected by Smart-Seq of HBs-specific CD8+ T cells in the livers of two groups. Red dots indicated significantly up-regulated genes, green dots indicated significantly down-regulated genes, and gray dots indicated non-significantly genes. (B) Hierarchical clustering of differentially expressed immune-related genes. Relative log [FPKM (fragments per kilobase of transcript per million fragments mapped)] expression levels of genes are displayed in red–blue colour scale. (C) and (D) The differentially expressed immune-related genes from WT and EXP-WT groups were classified by Gene Ontology analysis and KEGG pathway analysis.
![Figure 3. Immune-related genes expression in HBs-specific CD8+ T cells was significantly up-regulated in the liver of EXP-WT mice. (A) The volcano map of differentially expressed genes (log2FC > 1 and P-adjust <.05 as significant difference) detected by Smart-Seq of HBs-specific CD8+ T cells in the livers of two groups. Red dots indicated significantly up-regulated genes, green dots indicated significantly down-regulated genes, and gray dots indicated non-significantly genes. (B) Hierarchical clustering of differentially expressed immune-related genes. Relative log [FPKM (fragments per kilobase of transcript per million fragments mapped)] expression levels of genes are displayed in red–blue colour scale. (C) and (D) The differentially expressed immune-related genes from WT and EXP-WT groups were classified by Gene Ontology analysis and KEGG pathway analysis.](/cms/asset/c05dd6d4-83f8-42ee-897c-a09621d3cf9a/temi_a_2071172_f0003_oc.jpg)
To further confirm the stronger cytotoxic function of HBs-specific CD8+ T cells in HBsAg intrauterine exposure mice, the ability of HBs-specific CD8+ T cells to produce IFN-γ was determined by ELISPOT assay. The results showed that the numbers and intensities of IFN-γ spots produced by HBs-specific CTLs from both liver (P = .0029 and P = .0015, respectively) and spleen (P = .036 and P = .0094, respectively) in 4-week-old EXP-WT female mice were significantly higher than that in WT female mice ((A,B)). While the higher number and intensity of IFN-γ spots were only observed in the HBs-specific CTLs from the liver of EXP-WT male mice (P < .001 and P < .001, respectively) ((A,C)). Similar phenomena were also observed in HBs-specific CD8+ T cells post HBsAg stimulation ((D–F)), in which the numbers and intensities of IFN-γ spots produced by intrahepatic CTLs in EXP-WT mice were significantly higher than that in WT mice, whether in female (P < .001 and P < .001, respectively) or in male (P = .010 and P = .042, respectively). However, no difference was observed in splenic lymphocytes. These results suggested that HBs-specific CD8+ T cells in the liver of EXP-WT mice had a stronger capability to secrete IFN-γ.
Figure 4. The IFN-γ-producing abilities of HBs-specific CD8+ T cells in EXP-WT mice were significantly increased than those in WT group. (A) Representative images of IFN-γ spots determined by ELISPOT in HBs-specific CTLs of 4-week-old mice. Wells added RPMI1640 without FBS were used as negative control and wells with PMA were used as positive control. (B) and (C) Comparation of the numbers (left) and intensities (right) of IFN-γ spots produced by HBs-specific CTLs between 4-week-old EXP-WT mice and WT mice. (B), female mice. (C), male mice. (D) Representative images of IFN-γ Spots in HBs-specific CTLs determined by ELISPOT assay in HBs-specific CTLs at 7 days after stimulation (n = 8). (E) and (F) Comparation of the numbers (left) and intensities (right) of IFN-γ spots produced by HBs-specific CTLs after stimulation between 4-week-old EXP-WT mice and WT mice. (E), female mice. (F), male mice. *P < .05; **P < .01; ***P < .001.
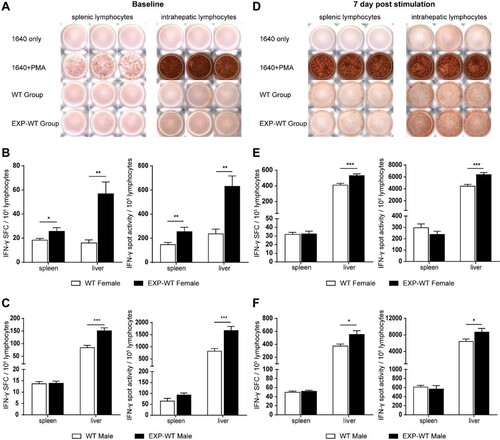
Taken together, the above results confirmed that more HBs-specific CD8+ T cells with powerful cytolytic functions in EXP-WT mice were activated than that in WT mice, which may be account for the stronger cellular immune response in EXP-WT mice.
HBsAg intrauterine exposure triggers the HBs-specific CD8+ T cellular memory
A major feature of the adaptive immune response is the presence of immune memory, which is maintained even after disease resolution. It provides the more rapid establishment of stronger immune defences when the same pathogen is encountered again. We noticed that the expression of Cd44, Il2rb and Eomes, which were considered as the master regulators of CD8+ effector and memory T cells, was significantly increased in the EXP-WT group mice in our Smart-Seq data ((B) and S6C), indicating that there might be more memory phenotype CD8+ T cells in EXT-WT group [Citation26,Citation27]. To further confirm whether more memory phenotype CD8+ T cells existed in EXT-WT mice, the percentage of memory T cell subpopulation of HBs-specific CD8+ T cells in mice livers were analysed by flow cytometry after 7 days of HBsAg stimulation. As shown in (A), the proportion of intrahepatic HBs-specific central memory T cells (Tcm, CD44+ CD62L+) of male mice and effector memory T cells (Tem, CD44+ CD62L-) of female mice in EXP-WT group was significantly higher than that in WT mice with the same gender, respectively ((B,C)). These results suggested that HBsAg intrauterine exposure resulted in the generation of cellular immunological memory, which may contribute to the quick clearance of HBV in those EXP-WT mice.
Figure 5. More HBs-specific CD8+ memory T cells in the liver of EXP-WT mice were detected than WT mice. (A) Central memory T cells (Tcm) and effector memory T cells (Tem) in HBs-specific CD8+ T cells in the livers of WT mice (left) and EXP-WT mice (right) were analysed by flow cytometry after 7 days HBsAg stimulation. (B) Analysis of the percentage of Tcm and Tem in HBs-specific CD8+ T cells in the liver of two groups of female mice. (C) Statistical analysis of the percentage of Tcm and Tem in HBs-specific CD8+ T cells in the liver of two groups of male mice. *P < .05; **P < .01.
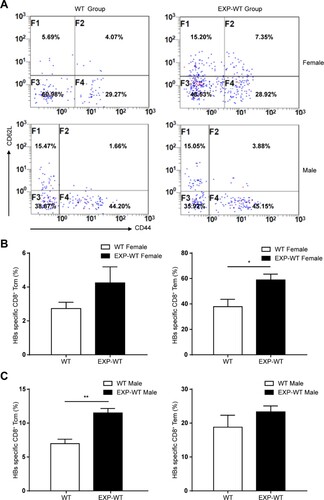
Next, the anti-HBs levels were detected in offspring mice to investigate the humoral immune response after vaccination. As shown in Figure S8A, the positive conversion rate and anti-HBs titre in EXP-WT mice after primer immunization were marginally higher than that in the WT group, and the difference in antibody positive conversion rate reached a statistic significance between the females in the two groups (P = .0096). However, such difference disappeared after subsequent booster immunizations (Figure S8B), which suggested that HBsAg intrauterine exposure would not cause adverse humoral immune to vaccination. Instead, HBsAg intrauterine exposure might promote the humoral immune response, though the effect was mild.
Increased CD8+ T cells response may attribute to antigen-presenting capability of DCs in HBsAg intrauterine exposure mice
During the priming activation of CD8+ T cells, professional antigen-presenting cells (APCs) play a critical role. DCs, matured in the second trimester, are the powerful antigen-presenting cells essential for the formation of immunological memory [Citation28]. According to the sequencing results of CD11c+ cells isolated from the liver in mice of two groups (Figure S9), we found that some of the DEGs were upregulated in EXP-WT mice, such as Cd83 and Cd86, suggesting the presence of more mature DCs with stronger function in the liver of EXP-WT mice. To further explore whether DCs involved in the formation of immunological memory in EXP-WT mice, the mononuclear cells were isolated from the liver and spleen of two groups, and both the number and function of DCs were investigated. The flow cytometry gating strategy of DCs was presented in Figure S4B. As expected, the proportion of mature DCs in the liver and spleen of 4-week-old EXP-WT mice was significantly higher than that in the WT group, with the exception of that in the liver of male mice ((A,B)). It has been reported that asialoglycoprotein receptor (ASGPR) expressed on the surface of DCs (DC-ASGPR or CD301), can bind to, take up and then present HBsAg to adaptive immune cells [Citation14,Citation29,Citation30]. Next, RT-qPCR and flow cytometry were employed to explore whether HBsAg intrauterine exposure could affect DC-ASGPR expression. The results showed that the expression of DC-Asgpr was significantly higher in female EXP-WT mice than WT mice, while marginally higher in male EXP-WT mice than WT mice ((C)). The similar trend in the proportion of CD301+ DCs was also observed, although there was no statistical difference ((D,E)).
Figure 6. The antigen presentation ability of DCs in EXP-WT group mice was stronger. (A) Analysis of mature DCs populations isolated from spleen and liver in 4-week-old WT group (left) and EXP-WT group (right) (female, n = 3; male, n = 3). (B) Comparation of the mature DCs populations of in spleen (left) and liver (right) (A) between two groups of mice. (C) Relative expression level of DC-Asgpr in 4-week-old female (left) and male (right) mice of WT group and EXP-WT Group (n = 7). (D) Analysis of the expression of CD301 on the surface of DCs in the liver of mice in the WT group and EXP-WT group (n = 3). (E) Comparation of the CD301+ DCs of (D) between two groups of mice. (F) Analysis of the CD86 expression on the surface of mature DCs in the liver of female (left) and male (right) mice in both groups per week after HBsAg stimulation. *P < .05; **P < .01.
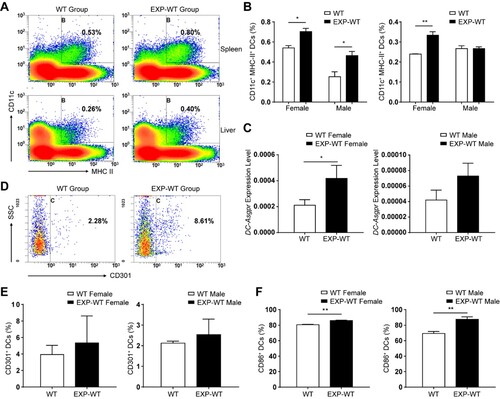
Since the activation of T cells also requires non-specific co-stimulation signals, such as CD80, CD86 and so on [Citation31], the expression of such co-stimulators in DCs after one-week HBsAg re-exposed was measured. As shown in (F), the percentage of CD86+ mature DCs in the EXP-WT group was significantly higher than that in the WT group in both males and females (P = .0048 and P = .0032, respectively), suggesting that they could induce more T cells proliferation and activation. Consistence with that, Smart-seq results also suggested that activation markers of DCs, such as CD83, CD86, and antiviral cytokines secreted by DCs were significantly upregulated in the EXP-WT group rather than the WT group (Figures S9C, S9D and S9E). And RT-qPCR was also used to verify the upregulated expression of these genes (Figure S7B). The above results indicated that EXP-WT mice had more mature DCs with increased antigen presentation capacity, which may induce stronger activation of adaptive immunity.
HBsAg exposure in utero affected the expression of immune-related genes in fetal liver
To explore whether the HBsAg intrauterine exposure could affect the gene expression in fetal mice, transcriptome of the fetal liver tissues between the two groups of mice was analysed by RNA-Seq assays. A total of 1768 DEGs were identified, including 917 up-regulated and 851 down-regulated genes in the EXP-WT mice ((A)). KEGG pathway analysis showed that the top 10 enrichment KEGG pathways of DEGs in the fetal liver included “PPAR signalling pathway,” “Insulin resistance,” “Fatty acid degradation” and so on, which were closely related to the metabolic function of the liver (Figure S10B). GO annotations enrichment analysis indicated that 134 DEGs were mainly enriched in “immune system” ((B)). Further, the GO and KEGG enrichment analysis of 134 immune-related genes showed that HBsAg intrauterine exposure significantly promoted the biological functions of “positive regulation of IFN-γ production,” “B cell activation,” and “regulation of cytokine production in immune response” in fetal liver, and so did the “JAK-STAT signalling pathway,” “Toll-like receptor signalling pathway,” and “Hematopoietic pathway” ((C,D)). Noticeably, the expression of Cd2, Gzma, Gzmb, Tgtp2, Il2rb and Cd7 in EXP-WT mice were significantly higher than that in WT mice, suggesting that the intrahepatic T cells immune response in the embryonic period has already been increased to some extent (Figure S10C). In addition, the expression of lgkv, Cd79b and Ms4a1 were also upregulated in the HBsAg intrauterine exposed fetal liver tissues, suggesting that HBsAg exposure might affect the differentiation and development of B cells. The expression level of DEGs mentioned above was also verified by RT-qPCR (Figure S7C). Taken together, these results suggested that HBsAg intrauterine exposure activated the adaptive immune response in the liver during the embryonic period.
Figure 7. Significant differences in the expression of immune-related genes in the liver tissues of fetal EXP-WT group mice and WT group mice. (A) The volcano map of 1768 differentially expressed genes (DEGs) in two groups. Each point in the figure represented one gene, 917 red dots indicated significantly up-regulated genes, 851 green dots indicated significantly down-regulated genes. (B) Histogram of GO enrichment classification statistics of DEGs. The results showed that the DEGs related to “immune system progression” were enriched in Biological process classification. (C) Bubble chart of GO enrichment of immunity-related DEGs in fetal liver of two groups. (D) Bubble diagram of the KEGG pathway of DEGs related to immune in two groups. The DEGs in each group have statistically significant differences.
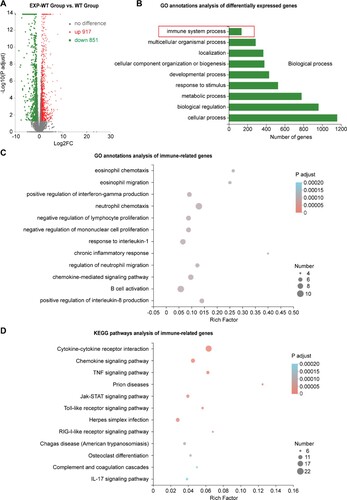
Discussion
It has been reported that HBeAg could cross the placental barrier and the maternal-derived HBeAg in offspring impaired HBV-specific T cells response, resulting in HBV persistence [Citation8]. However, the effect of HBsAg intrauterine exposure on the HBV persistence and immune response of the offspring has not been thoroughly elucidated. In this study, by use of a mouse model of solely HBsAg intrauterine exposure, we demonstrated for the first time that, contrary to the immunosuppressive effect of HBeAg, HBsAg intrauterine exposure did not cause the immune tolerance to HBV in the offspring, but instead, accelerated the clearance of HBV when re-exposed to HBV. Our data provide an important experimental evidence for the activation of neonatal immune response by HBsAg intrauterine exposure.
HBsAg can stimulate the body to produce protective antibodies, and at the same time, high HBsAg levels can also induce immune tolerance in CHB patients [Citation32–34]. However, definitive evidence for the function of HBsAg in intrauterine exposure to influence the immune response of offspring remains unclear. In our present study, a faster rate of HBV clearance was observed in the HBsAg intrauterine exposed group of mice when simulated re-exposure of HBV after birth by HI of HBV plasmid. Most importantly, we found that HBsAg intrauterine exposure increased the number and function of HBs-specific CD8+ T cells, characterized by more IFN-γ secretion and lower PD-1 expression. It is well known that IFNα and IL12 are the third signal required for the activation and differentiation of CD8+ T cells [Citation35,Citation36], IRF4 is crucial for the immune response of effector CD8+ T cells [Citation37], and GzmA and GzmB are the main effectors secreted by CTLs which can mediate target cell apoptosis and pyroptosis [Citation38,Citation39]. As expected, data of Smart-Seq and RT-qPCR indicated that the expression of all these factors was significantly increased in HBs-specific CD8+ T cells derived from the EXP-WT mice. Since HBV-specific CD8+ T lymphocytes are the most important effector cells of adaptive immune response against HBV [Citation40], these findings confirmed that HBsAg intrauterine exposure activated HBs-specific CD8+ T cells and accelerated the clearance of HBV in the offspring when they re-exposed to HBsAg after birth.
As reported by Reinhold Schirmbeck et al. and Petra Riedl et al. [Citation41,Citation42], amino acid sequence between the small HBsAg of genotype D (ayw) and genotype C (adr) HBV was similar, especially H-2 Kb-restricted S190–197 epitope, which we used in our study. Therefore, the T cell immune response induced during HBV infection of both genotypes is consistent. Considering the most common HBV genotype infected in Chinese patients are C or B and infected with genotype C HBV will lead to more severe liver damages [Citation43], we used the C genotype HBV plasmid to mimick HBV re-exposure, while the transgenic mice were integrated with genotype D HBV. Also, we compared the amino acid sequence between the small HBsAg of genotype C strain and D strain used in this study (data not shown) and found that most CD8+ T cell epitopes and “a” determinant region were highly conserved between these two strains. Therefore, we think that it would not affect the immune response in offspring when re-exposed to genotype C HBsAg in our study. Nevertheless, Riedl et al. also reported that CD8+ T cells primed by genotype D HBV showed relatively low cross-reactive recognition of the genotype A HBV epitope [Citation42], which suggested that anti-HBsAg CD8+ T cell responses to genotype A and D HBV may differ significantly. Further studies should be performed to investigate the potential difference in the immune responses between re-exposure to the same and to another strain.
As a powerful antigen-presenting cell, functional DCs play an important role in initiating strong T cell response and generation of immunological memory. Importantly, previous study has shown that the function of DCs would not be inhibited by HBV intrauterine exposure [Citation44]. Indeed, we found the presence of more mature DCs with stronger function in the liver of EXP-WT mice in this study. Previous study has reported that the DC-ASGPR expressed on the surface of DCs can co-localize with HBsAg and participate in the endocytosis and presenting process of the antigen [Citation29]. In line with it, we did find that the expression of DC-ASGPR in EXP-WT mice was increased. These results suggested that HBsAg intrauterine exposure could promote the uptake and presentation of HBsAg by DCs. In addition, significantly higher expression of Cd44 and Eomes, two master regulators of memory T cells [Citation45,Citation46], was observed in EXP-WT mice in this study. Consistently, more HBs-specific CD8+ Tcm and Tem in the liver of EXP-WT mice were found by using flow cytometry as compared to those in WT mice. These results indicated that HBsAg intrauterine exposure triggered cellular immunological memory which might account for the accelerated clearance of HBV in EXP-WT mice after re-exposed. In support of our observation, a recent literature reported that CD8+ T cells of early developmental origin have an inherent tendency to differentiate into virtual memory cells, which contribute to the aggressive and rapid response to infections after birth [Citation47]. Taken together, our findings and other literature reports suggested that HBsAg intrauterine exposure promoted the uptake and presentation of HBsAg by DCs, which in turn enhanced the cellular immune response and potentially induced antigen-specific cellular immunological memory.
Combining studies from other scholars with our results [Citation10–14,Citation48], we deduced that intrauterine exposure to HBsAg occurred and promoted the immune response in offspring in our model. To further investigate whether HBsAg intrauterine exposure could activate the adaptive immune response in the liver during the embryonic period, we analysed the changes of transcriptome in the fetal liver tissues. We found that the expression of Cd2 and Cd7, the characteristic surface markers of T cells [Citation49], was significantly higher in fetal liver tissue of the EXP-WT group. Meanwhile, the expression of Gzma and Gzmb in fetal liver tissue of EXP-WT mice was significantly higher than that of WT mice. These results suggested that more thymus-induced T cells migrated and distributed in fetal liver tissue of EXP-WT mice, and they drove a stronger function of CTLs during the embryonic period. Such activation of HBs-specific CD8+ T cells would arise the concern of immunopathological damage in fetal liver. However, a recent report suggested that after recognizing and presenting alloantigens, fetal DCs can significantly induce the production of regulatory T cells (Treg) to prevent the overactivation of CD8+ T cells [Citation28]. Additionally, a higher positive conversion rate of anti-HBs was obtained in EXP-WT mice after primer immunization in this study. In line with this, the expression of Cd79b and Ms4a1, the important regulator for B cells proliferation and differentiation [Citation50,Citation51], was significantly up-regulated in fetal liver tissue of EXP-WT mice, suggesting the more active humoral immune response happened even during the embryonic period. Therefore, the current study provided an alternative explanation for the clinical observation that the newborns of only HBsAg-positive mothers are less likely to become chronically infected, especially after NAs treatment. Meanwhile, our study also supported the notion that only HBsAg positive does not inhibit the immune response of the progeny vaccination [Citation18]. Besides, KEGG pathway analysis of all dysregulated genes in the fetal liver of two groups of mice showed that intrauterine exposure to HBsAg affected the metabolic function of the liver, which indicated that HBsAg might affect the function of glucose and lipid metabolism in liver cells. Consistent with our findings, Li et al. suggested that HBsAg seroclearance rate was associated with fatty liver [Citation52]. This was an interesting finding that we will explore further in the future study.
It has been reported that gender can affect the rate of clearance of HBV in mice, the impaired function of HBV-specific CD8+ T cells can be observed in male mice due to the presence of a greater number of Treg cells in the liver, resulting in slower clearance of HBV [Citation24]. Consistent with this report, we also found that the overall clearance rate of HBV was faster in female mice than that in male mice. Also, more HBs-specific CD8+ T cells and DCs in the liver of female mice, consistence with more IFN-γ secreted by CTLs in female mice suggested that the intrauterine exposed female mice may have a stronger HBV-specific immune response than male mice. In addition, we noticed that although the proportion of HBs-specific CD8+ T cells in the liver of EXP-WT female mice was significantly higher than that in WT female mice, the difference in HBsAg level after HBV re-exposed between the two groups of female mice was not as pronounced as that of male mice. We speculated that the immune response to HBsAg of female mice may be strong enough for HBV clearance whether with or without intrauterine exposure and thus the difference in HBsAg level was not such significant in female mice.
Certainly, our study also had its limitations. Although the placental exchange physiology of mice has been found to be similar to that of humans [Citation53], mouse experiments cannot fully simulate human intrauterine exposure to HBV, and the titre of HBsAg in this mouse model might be lower than that of pregnant women in the real world. Also, since mice cannot be infected with HBV, we can only mimic HBV postnatal infection by using the HBV replication model, which cannot fully reflect the process of virus infection in patients during the peripartum and infancy. And although Wang et al. have reported that HBsAg can be detected in the amniotic fluid of C57BL/6-Tg (HBV Alb-1) Bri44 mice [Citation48], which was exactly the mouse model we used in our study, we have not explored exactly how intrauterine exposure of HBsAg occurred. Therefore, more clinical and animal experiments are needed for verification and exploration in future studies.
In summary, our study showed that the adaptive immune response against HBV already existed in the fetal liver of solely HBsAg intrauterine exposed mice, and as a result, HBsAg intrauterine exposure could accelerate the clearance of HBV by the enhanced immune response of DCs and HBs-specific CTLs in the offspring.
Supplemental Material
Download MS Word (4.6 MB)Acknowledgements
We thank all the colleagues who assisted in this research. Jing Ning and Jianwen Wang performed the research, analysed and interpreted the data, drafted and revised the manuscript; Huiling Zheng, Siwen Peng, Tianhao Mao, Lu Wang and Guangxin Yu performed the research; Jia Liu, Shuang Liu and Ting Zhang provided administrative, technical or material support; Shigang Ding, Fengmin Lu and Xiangmei Chen designed the research, obtained funding, supervised the study and revised the manuscript for important intellectual content. All authors of this research have approved the final version of the article.
Disclosure statement
No potential conflict of interest was reported by the author(s).
Data availability statement
The Smart-seq data of 300–1000 CD11c+ cells sorted from mice liver of two groups in the study were deposited in the SRA (www.ncbi.nih.gov.sra) repository, with accession number PRJNA754108. The Smart-seq data of 300–1000 HBs-specific CD8+ T cells sorted from mice liver and RNA sequencing data of total RNA extracted from fetus mice liver of two groups in the study were deposited in the GEO (www.ncbi.nlm.nih.gov/geo) database, with accession number GSE162718 and GSE162717 respectively.
Additional information
Funding
References
- Polaris Observatory Collaborators. Global prevalence, treatment, and prevention of hepatitis B virus infection in 2016: a modelling study. Lancet Gastroenterol Hepatol. 2018;3(6):383–403.
- Chang MH. Natural history and clinical management of chronic hepatitis B virus infection in children. Hepatol Int. 2008;2(Supplement 1):28–36.
- Milich DR, Jones JE, Hughes JL, et al. Is a function of the secreted hepatitis B e antigen to induce immunologic tolerance in utero? Proc Natl Acad Sci U S A. 1990;87(17):6599–6603.
- Publicover J, Gaggar A, Nishimura S, et al. Age-dependent hepatic lymphoid organization directs successful immunity to hepatitis B. J Clin Invest. 2013;123(9):3728–3739.
- Publicover J, Goodsell A, Nishimura S, et al. IL-21 is pivotal in determining age-dependent effectiveness of immune responses in a mouse model of human hepatitis B. J Clin Invest. 2011;121(3):1154–1162.
- Piratvisuth T. Optimal management of HBV infection during pregnancy. Liver Int. 2013;33(Suppl 1):188–194.
- Gentile I, Zappulo E, Buonomo AR, et al. Prevention of mother-to-child transmission of hepatitis B virus and hepatitis C virus. Expert Rev Anti Infect Ther. 2014;12:775–782.
- Tian Y, Kuo CF, Akbari O, et al. Maternal-derived hepatitis B virus e antigen alters macrophage function in offspring to drive viral persistence after vertical transmission. Immunity. 2016;44:1204–1214.
- Burk RD, Hwang LY, Ho GY, et al. Outcome of perinatal hepatitis B virus exposure is dependent on maternal virus load. J Infect Dis. 1994;170(6):1418–1423.
- Chen Y, Wang L, Xu Y, et al. Role of maternal viremia and placental infection in hepatitis B virus intrauterine transmission. Microbes Infect. 2013;15:409–415.
- Ma L, Alla NR, Li X, et al. Mother-to-child transmission of HBV: review of current clinical management and prevention strategies. Rev Med Virol. 2014;24:396–406.
- Wong VC, Lee AK, Ip HM. Transmission of hepatitis B antigens from symptom free carrier mothers to the fetus and the infant. Br J Obstet Gynaecol. 1980;87(11):958–965.
- Wei J, Xue S, Zhang J, et al. Study of the relationship in pregnant women between hepatitis B markers and a placenta positive for hepatitis B surface antigen. J Perinat Med. 2015;43(2):191–199.
- Vyas AK, Ramakrishna U, Sen B, et al. Placental expression of asialoglycoprotein receptor associated with hepatitis B virus transmission from mother to child. Liver Int. 2018;38(12):2149–2158.
- Tan J, Liu X, Mao X, et al. HBsAg positivity during pregnancy and adverse maternal outcomes: a retrospective cohort analysis. J Viral Hepat. 2016;23:812–819.
- Wang J, He Y, Jin D, et al. No response to hepatitis B vaccine in infants born to HBsAg(+) mothers is associated to the transplacental transfer of HBsAg. Infect Dis (Lond). 2017;49(8):576–583.
- Trehanpati N, Hissar S, Shrivastav S, et al. Immunological mechanisms of hepatitis B virus persistence in newborns. Indian J Med Res. 2013;138(5):700–710.
- Kang G, Ma F, Chen H, et al. Efficacy of antigen dosage on the hepatitis B vaccine response in infants born to hepatitis B-uninfected and hepatitis B-infected mothers. Vaccine. 2015;33(33):4093–4099.
- Hong M, Sandalova E, Low D, et al. Trained immunity in newborn infants of HBV-infected mothers. Nat Commun. 2015;6:6588.
- Chisari FV, Filippi P, McLachlan A, et al. Expression of hepatitis B virus large envelope polypeptide inhibits hepatitis B surface antigen secretion in transgenic mice. J Virol. 1986;60(3):880–887.
- Nicholson A, Reifsnyder PC, Malcolm RD, et al. Diet-induced obesity in two C57BL/6 substrains with intact or mutant nicotinamide nucleotide transhydrogenase (Nnt) gene. Obesity. 2010;18:1902–1905.
- Huang LR, Wu HL, Chen PJ, et al. An immunocompetent mouse model for the tolerance of human chronic hepatitis B virus infection. Proc Natl Acad Sci U S A. 2006;103(47):17862–17867.
- Liu Y, Qi X, Zeng Z, et al. CRISPR/Cas9-mediated p53 and Pten dual mutation accelerates hepatocarcinogenesis in adult hepatitis B virus transgenic mice. Sci Rep. 2017;7(1):2796.
- Kosinska AD, Pishraft-Sabet L, Wu W, et al. Low hepatitis B virus-specific T-cell response in males correlates with high regulatory T-cell numbers in murine models. Hepatology. 2017;66(1):69–83.
- Maier H, Isogawa M, Freeman GJ, et al. PD-1:PD-L1 interactions contribute to the functional suppression of virus-specific CD8+ T lymphocytes in the liver. J Immunol. 2007;178(5):2714–2720.
- McLane LM, Banerjee PP, Cosma GL, et al. Differential localization of T-bet and Eomes in CD8 T cell memory populations. J Immunol. 2013;190(7):3207–3215.
- Miller CH, Klawon DEJ, Zeng S, et al. Eomes identifies thymic precursors of self-specific memory-phenotype CD8+ T cells. Nat Immunol. 2020;21(5):567–577.
- McGovern N, Shin A, Low G, et al. Human fetal dendritic cells promote prenatal T-cell immune suppression through arginase-2. Nature. 2017;546(7660):662–666.
- Valladeau J, Duvert-Frances V, Pin JJ, et al. Immature human dendritic cells express asialoglycoprotein receptor isoforms for efficient receptor-mediated endocytosis. J Immunol. 2001;167(10):5767–5774.
- Untergasser A, Zedler U, Langenkamp A, et al. Dendritic cells take up viral antigens but do not support the early steps of hepatitis B virus infection. Hepatology. 2006;43(3):539–547.
- Maroof A, Beattie L, Kirby A, et al. Dendritic cells matured by inflammation induce CD86-dependent priming of naive CD8+ T cells in the absence of their cognate peptide antigen. J Immunol. 2009;183(11):7095–7103.
- Mueller SN, Ahmed R. High antigen levels are the cause of T cell exhaustion during chronic viral infection. Proc Natl Acad Sci U S A. 2009;106(21):8623–8628.
- Urban S, Bartenschlager R, Kubitz R, et al. Strategies to inhibit entry of HBV and HDV into hepatocytes. Gastroenterology. 2014;147(1):48–64.
- Cornberg M, Wong VW, Locarnini S, et al. The role of quantitative hepatitis B surface antigen revisited. J Hepatol. 2017;66(2):398–411.
- Cho JH, Kim HO, Kim KS, et al. Unique features of naive CD8+ T cell activation by IL-2. J Immunol. 2013;191(11):5559–5573.
- Hervas-Stubbs S, Riezu-Boj J-I, Gonzalez I, et al. Effects of IFN-α as a signal-3 cytokine on human naïve and antigen-experienced CD8(+) T cells. Eur J Immunol. 2010;40(12):3389–3402.
- Huang S, Shen Y, Pham D, et al. IRF4 modulates CD8T cell sensitivity to IL-2 family cytokines. Immunohorizons. 2017;1(6):92–100.
- Abbas AK, Lichtman AH, Pillai S. Cellular and molecular immunology. 9th ed. Philadelphia (PA): Elsevier; 2018. p. 1–11.
- Zhou Z, He H, Wang K, et al. Granzyme A from cytotoxic lymphocytes cleaves GSDMB to trigger pyroptosis in target cells. Science. 2020;368(6494):eaaz7548.
- Balkow S, Kersten A, Tran TT, et al. Concerted action of the FasL/Fas and perforin/granzyme A and B pathways is mandatory for the development of early viral hepatitis but not for recovery from viral infection. J Virol. 2001;75(18):8781–8791.
- Schirmbeck R, Böhm W, Fissolo N, et al. Different immunogenicity of H-2 Kb-restricted epitopes in natural variants of the hepatitis B surface antigen. Eur J Immunol. 2003;33(9):2429–2438.
- Riedl P, Bertoletti A, Lopes R, et al. Distinct, cross-reactive epitope specificities of CD8T cell responses are induced by natural hepatitis B surface antigen variants of different hepatitis B virus genotypes. J Immunol. 2006;176(7):4003–4011.
- Gu X, Yang X, Wang D, et al. Comparison and significance of specific and non-specific cellular immunity in patients with chronic hepatitis B caused by infection with genotypes B or C of hepatitis B virus. Sci China C Life Sci. 2009;52(8):719–723.
- Koumbi LJ, Papadopoulos NG, Anastassiadou V, et al. Dendritic cells in uninfected infants born to hepatitis B virus-positive mothers. Clin Vaccine Immunol. 2010;17(7):1079–1085.
- Sung CC, Horng JH, Siao SH, et al. Asialo GM1-positive liver-resident CD8T cells that express CD44 and LFA-1 are essential for immune clearance of hepatitis B virus. Cell Mol Immunol. 2021;18(7):1772–1782.
- McLane LM, Banerjee PP, Cosma GL, et al. Differential localization of T-bet and Eomes in CD8T cell memory populations. J Immunol. 2013;190(7):3207–3215.
- Smith NL, Patel RK, Reynaldi A, et al. Developmental origin governs CD8T cell fate decisions during infection. Cell. 2018;174(1):117–130.e14.
- Wang R, Chen K, Wang Y, et al. Booster immunization improves the generation of T follicular helper (Tfh) cells specific to hepatitis B surface antigen (HBsAg) after prenatal HBsAg exposure. Vaccine. 2021;39(39):5571–5579.
- Mayer KE, Mall S, Yusufi N, et al. T-cell functionality testing is highly relevant to developing novel immuno-tracers monitoring T cells in the context of immunotherapies and revealed CD7 as an attractive target. Theranostics. 2018;8(21):6070–6087.
- Nance CL, Shearer WT. SDF-1alpha regulates HIV-1-gp120-induced changes in CD79b surface expression and Ig production in activated human B cells. Clin Immunol. 2002;105(2):208–214.
- Kawabata KC, Ehata S, Komuro A, et al. TGF-β-induced apoptosis of B-cell lymphoma Ramos cells through reduction of MS4A1/CD20. Oncogene. 2013;32(16):2096–2106.
- Li J, Yang HI, Yeh ML, et al. Association between fatty liver and cirrhosis, hepatocellular carcinoma, and hepatitis B surface antigen seroclearance in chronic hepatitis B. J Infect Dis. 2021;224(2):294–302.
- Dilworth MR, Sibley CP. Review: transport across the placenta of mice and women. Placenta. 2013;34(Suppl):S34–S39.