ABSTRACT
Ceftazidime-avibactam resistance attributable to the blaKPC-2 gene mutation is increasingly documented in clinical settings. In this study, we characterized the mechanisms leading to the development of ceftazidime-avibactam resistance in ST11-K47 hypervirulent Klebsiella pneumoniae that harboured the blaKPC-135 gene. This strain possessed fimbriae and biofilm, demonstrating pathogenicity. Compared with the wild-type KPC-2 carbapenemase, the novel KPC-135 enzyme exhibited a deletion of Glu168 and Leu169 and a 15-amino acid tandem repeat between Val262 and Ala276. The blaKPC-135 gene was located within the Tn6296 transposon truncated by IS26 and carried on an IncFII/IncR-type plasmid. Compared to the blaKPC-2-positive cloned strain, only the MIC of ceftazidime increased against blaKPC-135-positive K. pneumoniae and wasn’t inhibited by avibactam (MIC 32 μg/mL), while clavulanic acid and vaborbactam demonstrated some inhibition. Kinetic parameters revealed that KPC-135 exhibited a lower Km and kcat/Km with ceftazidime and carbapenems, and a higher (∼26-fold) 50% inhibitory concentration with avibactam compared to KPC-2. The KPC-135 enzyme exerted a detrimental effect on fitness relative to the wild-type strain. Furthermore, this strain possessed hypervirulent determinants, which included the IncHI1B/FIB plasmid with rmpA2 and expression of type 1 and 3 fimbriae. In conclusion, we reported a novel KPC variant, KPC-135, in a clinical ST11-K47 hypervirulent K. pneumoniae strain, which conferred ceftazidime-avibactam resistance, possibly through increased ceftazidime affinity and decreased avibactam susceptibility. This strain simultaneously harboured resistance and virulence genes, posing an elevated challenge in clinical treatment.
Introduction
The widespread epidemic of carbapenem-resistant Klebsiella pneumoniae (CRKP) in recent years has presented significant challenges to anti-infective treatment strategies in clinical settings [Citation1–3]. The production of carbapenemases, particularly class A KPC-type serine carbapenemases, represents the primary resistance mechanism [Citation4]. Ceftazidime-avibactam demonstrates excellent in vitro activity and safety against serine carbapenemases, leading to its recommendation as the first-line treatment for CRKP-related infections in China since 2019 [Citation5,Citation6]. However, the widespread clinical use of ceftazidime-avibactam has resulted in mutations in KPC-producing K. pneumoniae under strong antimicrobial selection pressure, leading to the emergence of ceftazidime-avibactam-resistant strains [Citation7,Citation8].
The resistance mechanisms of ceftazidime-avibactam predominantly involve hydrolytic enzymes, modifications or substitutions at target sites, and alterations in cell permeability or efflux pump activity, with amino acid substitutions in the KPC enzyme constituting a primary mechanism [Citation9]. The incidence of novel KPC gene subtypes has risen significantly since 2019. Globally, over 200 variants of the blaKPC gene have been identified, with half potentially conferring ceftazidime-avibactam resistance, as per the NCBI database. Amino acid mutations in the blaKPC gene are primarily located in the loop region near the active site [Citation10]. In addition, the transmission of these blaKPC genes is primarily mediated by plasmids or small genetic elements on them, particularly the Tn4401 transposon [Citation11]. Such resistance poses challenges to the clinical management of CRKP infections.
Previously, most the hypervirulent Klebsiella pneumoniae (HvKp) strains were susceptible to a broad range of antimicrobial agents. However, due to the continuous evolution of plasmids that confer hypervirulence or carbapenem resistance, hypervirulent and carbapenem-resistant K. pneumoniae strains have emerged in China, predominantly with serotype K1 or K2 [Citation12,Citation13]. In this study, we reported the blaKPC-135 gene located in truncated Tn6296, a novel blaKPC variant that confers resistance to ceftazidime-avibactam and restores susceptibility to carbapenems. This ST11-K47 type Klebsiella pneumoniae isolate exhibited both virulence and drug resistance, posing significant challenges for clinical practice.
Materials and methods
Isolate and patient data
The patient, a 38-year-old male, presented a lower respiratory tract infection. In 2022, he received treatment at a tertiary hospital in Anhui Province, China. The clinical strain of K. pneumoniae HSD, isolated from the sputum culture, exhibited resistant to ceftazidime-avibactam with an MIC >128 μg/mL. Tentative identification of the strain was made using MALDI-TOF MS (bioMérieux, Marcy-l’Étoile, France), with confirmation via whole genome sequencing. K. pneumoniae ATCC 13882, E. coli DH5α, E. coli J53 and E. coli BL21 were used in the genetic procedures.
The K. pneumoniae HSD strain, in its exponential phase, was resuspended and washed using phosphate buffered saline (PBS). Images of the K. pneumoniae HSD strain, subjected to methylamine tungstate negative staining and embedding experiment, were captured using FEI TECNAI G 20 TWIN (200 kV) transmission electron microscopy [Citation14].
Galleria mellonella killing assay
The K. pneumoniae HSD strain, in its exponential phase, was washed and adjusted to a 0.5 McFarland standard using PBS. G. mellonella larvae, each weighing between 150 and 200 mg, were injected with 10 μL of either bacterial suspension or PBS (negative control). The larvae were placed in petri dishes, kept in the dark at 37 ℃, and monitored daily for mortality. K. pneumoniae ATCC 700603 and NTUH-K2044 were used as low- and high-virulence control strains, respectively. Each experimental group consisted of 10 larvae and was independently repeated three times.
Abiotic biofilm formation assay
200 μL of Luria–Bertani (LB) broth was thoroughly mixed with 2 μL of a 0.5 McFarland standard bacterial suspension of K. pneumoniae HSD or PBS (negative control). The broth was inoculated into a 96-well flat-bottomed microplate and incubated at 37 ℃ for 24 h, then washed with PBS and dried. The microplate was stained with 100 μL of 0.5% crystal violet solution for 15 min, then washed and dried. Absorbance was measured at OD570 nm after adding 200 μL of ethanol to the wells and allowing it to dissolve for 10 min.
Antimicrobial susceptibility testing
Minimal inhibitory concentrations (MICs) were determined using the broth microdilution method, as prescribed by the Clinical and Laboratory Standards Institute (CLSI) reference [Citation15]. Antimicrobial agents, including β-lactams, β-lactam/β-lactamase inhibitor combinations, aminoglycosides, quinolones, sulfonamide antibiotics, were tested. Quality control and interpretation of the results adhered to the 2023 CLSI breakpoints [Citation15]. The MICs of amoxicillin, tigecycline, and polymyxin were interpreted according to the breakpoints established by the U.S. Food and Drug Administration and the European Committee on Antimicrobial Susceptibility Testing (EUCAST) [Citation16,Citation17].
Plasmid transformation and conjugation experiments
The conjugation experiment was conducted to assess the transferability of the ceftazidime-avibactam resistance from the donor strain K. pneumoniae HSD to azide-resistant E. coli J53 as the recipient. The transconjugants were selected using Luria–Bertani agar plates supplemented with ceftazidime-avibactam (1 μg/mL) and sodium azide (150 μg/mL). In the transformation experiment, plasmid DNAs were extracted from strain K. pneumoniae HSD via the phenol–chloroform method and subsequently electroporated into the recipient E. coli DH5α. These transformants were selected on LB agar plates containing ampicillin (50 μg/mL), subjected to PCR for detection of the blaKPC gene, and later sequenced and analyzed using the BLAST programme (http://blast.ncbi.nlm.nih.gov/Blast.cgi) [Citation18].
blaKPC cloning experiments
Cloning experiments were conducted as previously described [Citation19]. The wild-type blaKPC-2 and blaKPC-135 sequences, which included the wild promoter, were amplified, purified, and subsequently cloned into the pHSG398 vector. Both recombinant plasmids were then chemically transformed into K. pneumoniae ATCC 13882. LB agar plates containing 50 μg/mL chloramphenicol were used to select transformants, which were then verified by PCR and sequencing.
Pulsed-field gel electrophoresis (PFGE)
Using Salmonella braenderup H9812 as a reference marker, bacterial DNA was digested with Xba I and S1-nuclease and then analyzed by PFGE and S1-PFGE. PFGE was performed using the CHEF Mapper system (Bio-Rad Laboratories, Hercules, CA, USA) at 14°C, 6 V/cm, and alternating pulses at a 120° angle over a pulse time gradient of 2.16 - 54.17 s for 19 h. Results were then interpreted using BioNumerics software version 6.5.
Purification of KPC carbapenemase, steady-state enzyme kinetic measurements and determination of the 50% inhibitory concentration (IC50) values
The KPC-2 and KPC-135 enzymes were expressed, purified, and concentrated to 10–20 mg/mL as previously described [Citation20,Citation21]. The kinetic parameters of the KPC enzymes were determined by spectrophotometry at 25℃ in PBS with pH 7.4, and subsequently, Km and kcat values were derived by fitting the Michaelis–Menten equation. The IC50 values for the inhibition of KPC enzymes by avibactam, relebactam, and vaborbactam, and clavulanic acid were determined using nitrocefin as the substrate. The enzymes and inhibitors were co-incubated for 10 min before being added to nitrocefin. Absorbance at 482 nm was recorded over 30 min and analyzed using GraphPad Prism software version 9.
Fitness cost evaluation
The blaKPC-positive cloning strains were cultured in LB broth until the turbidity reached 0.5 McFarland standard. For the non-competitive growth kinetics analysis, the bacterial culture was incubated at 180 rpm and 37 ℃ following a 200-fold dilution, with growth recorded over 18 h by measuring OD600 at specified time points. In the competition assay, cloning strains expressing KPC-2 and KPC-135 were diluted 1:200 in LB broth and mixed at a 1:1 ratio. Following overnight incubation at 180 rpm, and 37 ℃, the culture was plated on LB agar containing meropenem or ceftazidime-avibactam, and colony counts were used to calculate relative fitness [Citation22].
Whole genome sequencing and analysis
Genomic DNA from K. pneumoniae HSD strains underwent whole-genome sequencing (WGS) using both Illumina (San Diego, CA, USA) short-read and Nanopore (Oxford, UK) long-read sequencing technologies. Antimicrobial resistance genes, virulence genes, plasmid replicons, serotype predictions, and multilocus sequence typing (MLST) of the strains were obtained through single genome analysis facilitated by BacWGSTdb 2.0 (http://bacdb.cn/BacWGSTdb/) and Pathogenwatch (https://pathogen.watch). Putative promoters were identified within 500 bp immediately upstream of the blaKPC gene using the online software rools, BPROM (https://www.softberry.com/berry.phtml?topic = bprom&group =programs&subgroup = gfindb) and Neural Network Promoter Prediction (http://www.fruitfly.org/seq_tools/promoter.html).
Results
Characteristics of K. pneumoniae HSD clinical strain
The clinical strain of K. pneumoniae HSD, producing the KPC-135 carbapenemase, appeared as a non-mucoid, white form with a negative string test result. Using negative staining and TEM imaging, the cells were short, rod-shaped, and displayed some heterogeneity, with slender, dense fimbriae and capsule-like structures throughout (a-c). The strain was capable of forming biofilms on abiotic surfaces and exhibited pathogenicity, leading to survival rates similar to the high-virulence strain NTUH-K2044 of G. mellonella (d).
Figure 1. Morphological characteristics and virulence of clinical isolate K. pneumoniae HSD. (a) Transmission electron microscopy (TEM) image of K. pneumoniae HSD with negative staining using methylamine tungstate. (b) TEM image of a transverse section of embedded K. pneumoniae HSD. (c) Colony morphology of K. pneumoniae HSD on Columbia blood agar. (d) Survival rates of G. mellonella infected with K. pneumoniae HSD and biofilm formation in K. pneumoniae HSD. Statistical analysis was performed using unpaired t-tests. ****, P < 0.0001.
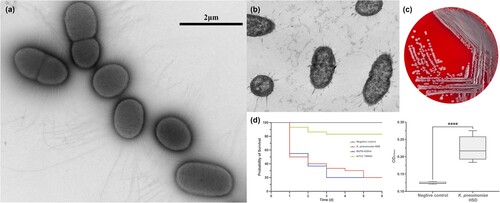
K. pneumoniae HSD, classified as sequence type (ST) 11 and serotype K47, showed high level resistance to the β-lactams (except for carbapenems), cephalosporins, aminoglycosides, and quinolones, among which resistance to aztreonam was inhibited by avibactam but not ceftazidime. It demonstrated susceptiblility to imipenem and resistant to meropenem and ertapenem with MICs of 4 and 32 μg/mL, respectively. Furthermore, trimethoprim-sulfamethoxazole, tigecycline, colistin, and polymyxin B had good in vitro activity against K. pneumoniae HSD (). The transformation experiment indicated that the blaKPC-135 gene was located on the plasmid pK. pneumoniae HSD-KPC, which was approximately 100 kb in length (Figure S1). Repeated failures in mating-out assays, conducted more than three times, suggested that pK. pneumoniae HSD-KPC might be a non-conjugative plasmid. Compared to the donor strain K. pneumoniae HSD, the transformant E.coli DH5α-K. pneumoniae HSD exhibited similar yet reduced resistance (except for quinolones), with resistance to aztreonam and amoxicillin being inhibited by avibactam and clavulanic acid, respectively. Mutation of the chromosomally encoded gyrA and parC genes could confer quinolone resistance in K. pneumoniae HSD.
Table 1. Minimal inhibitory concentrations (MICs) of K. pneumoniae HSD, blaKPC-135-positive transformants, and recipient strains.
Characteristics of the KPC-135 enzyme
Compared to the wild-type KPC-2 carbapenemase, KPC-135 had a deletion of Glu168 and Leu169, along with a 15-amino acid tandem repeat between amino acids Val262 and Ala276. This mutation caused significant changes in the Ω- and 270- loop structures, likely resulting in the loss of an α-helix and an expanded binding pocket (a). The empty vector and recombinant plasmids harbouring blaKPC were transferred into K. pneumoniae ATCC 13882 (). In addition to demonstrating an MIC of 4 μg/mL for cefepime, K. pneumoniae 13882-KPC-2 was resistant to the β-lactams tested. This resistance could be suppressed by inhibitors such as avibactam, relebactam, and vaborbactam, while amoxicillin maintained an MIC of 128 μg/mL, regardless of the presence of clavulanic acid. In contrast to K. pneumoniae clone strain 13882-KPC-2, the MICs of K. pneumoniae 13882-KPC-135 generally decrease; only the MIC of ceftazidime increased from 16 μg/mL to 128 μg/mL, accompanied by a reduced inhibitory effect of avibactam. In addition, clavulanic acid and vaborbactam showed some inhibition to K. pneumoniae 13882-KPC-135.
Figure 2. Structure and function of the β-lactamases KPC-135 and KPC-2 and their fitness costs. (a) Structure of KPC-2 (PDB ID: 3DW0) and KPC-135 enzyme (modelled by homology). The catalytic pockets of enzymes were depicted. (b) Kinetic parameters of purified KPC-2 (blue) and KPC-135 (yellow). The bar graph: MICs of blaKPC cloning strains are shown on the left vertical axis in base-2 logarithmic scale. The line graph: kcat/Km values of the enzymes were plotted on the right vertical axis. (c) IC50 of β-lactamase inhibitors against KPC-2 (blue) and KPC-135 (yellow). The bar graph: The reduced multiples of MICs of blaKPC cloning strains after addition of inhibitors to antibiotics are shown on the left vertical axis in base-2 logarithmic scale, with ceftazidime highlighted in blue. The line graph: The IC50 values were represented on the right vertical axis in an inverted base-10 logarithmic scale. (d) Relative fitness and (e) the bacterial growth curve of the K. pneumonia 13882-KPC-2 and 13882-KPC-135 cloning strains. Statistical analysis was performed using unpaired t-tests. ****, P < 0.0001.
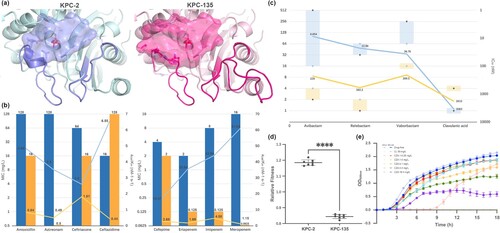
The kcat/Km values of the KPC-135 enzyme were generally lower than those of wild-type KPC-2, showing reductions of 9.6- to 53.03-fold and 1.44- to 14.89-fold with carbapenems and other β-lactams, respectively. Except for ceftriaxone and amoxicillin, KPC-135 exhibited a lower (1.11- to 35.8- fold) Km than KPC-2, indicating that the KPC-135 enzyme demonstrated reduced hydrolysis and generally higher affinity for β-lactams compared to wild-type KPC-2 (b). The IC50 values of the inhibitors against the enzymes, as well as the fold decrease in MICs of the antibiotics with added inhibitors against the cloning strains, were inversely correlated and were assessed to evaluate inhibitory activity (c). Avibactam and relebactam showed approximately 26-fold higher IC50 values against KPC-135 than against KPC-2, while vaborbactam exhibited a 5.70-fold increase, indicating that KPC-135 was associated with low affinity and reduced susceptibility to these inhibitors. Conversely, clavulanic acid showed a 1.87-fold lower IC50 value against KPC-135 than KPC-2, suggesting enhanced inhibitory activity. In addition, K. pneumonia 13882-KPC-2 exhibited a higher growth rate than K. pneumoniae 13882-KPC-135 under 50 μg/mL chloramphenicol or drug-free conditions, indicating the apparent fitness cost associated with the KPC-2 mutation (d). Under ceftazidime-avibactam pressure, the strains demonstrated markedly slow growth, even in the absence of MIC concentrations.
Genomic comparison of Tn6296-associated blaKPC-bearing plasmids
pK. pneumoniae HSD-KPC was a 98,672 bp IncFII/IncR plasmid that carried the blaKPC-135 gene (). It contained two fused resistance regions: the blaKPC region carrying blaKPC-135 and the MDR region harbouring blaTEM-1B, blaCTX-M-65, rmtB, and fosA3. Many published IncFII/IncR-type plasmids closely resembled pK. pneumoniae HSD-KPC, among which p2b2 and p15WZ61-KPC were representative, each harbouring blaKPC-2 with identity exceeding 99.95% (). Compared to pK. pneumoniae HSD-KPC, these plasmids had three similar gene insertions, mainly comprising genes from the F plasmid-encoded transfer (Tra) systems, resistance genes (blaSHV-12 and cat), and surrounding transposable elements. Plasmid p2b2 also exhibited two deletions, including blaCTX-M-65, fosA3, and maintenance modules (psiB, ardA, parAB, and umuCD).
Figure 3. Comparative plasmid map of pK. pneumoniae HSD-KPC, p2b2, and p15WZ61-KPC. From the inside to the outside circles: circle 1, scale; circle 2, GC content; circle 3, GC Skew; circle 4, ring diagram of p15WZ61-KPC (GenBank accession number ON777848); circle 5, ring diagram of p2b2 (GenBank accession number CP034125); circle 6, functionally classified genes. MDR, blaKPC, and insertion regions of aligned sequences have been annotated.
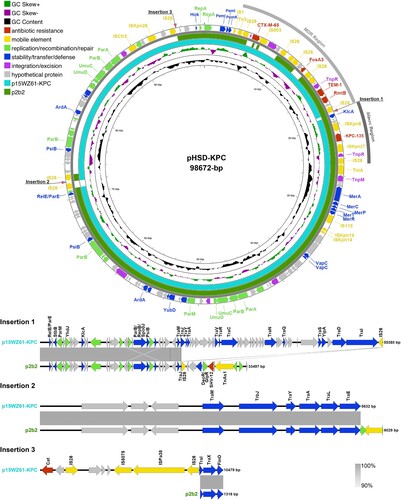
The blaKPC-135 region of plasmid pK. pneumoniae HSD-KPC consisted of IS26 and △Tn6296 with truncated ends (). BLAST analysis identified ten blaKPC-2 variants within Tn6296-associated transposons in plasmids, predominantly located in China. The blaKPC-2-positive pP10159-3 was utilized as the reference, organized in the order of a Tn6376 transposon, blaKPC-2, truncated ISKpn6, kroC, klcA, orf, △repB, and a truncated TnAs1 transposon. Plasmids harbouring blaKPC-2 variants showed two evolutionary paths. One path involved the truncation of Tn6376, leaving only the resolvase (tnpR) residue and ISKpn27; the other entailed the replacement of △TnAs1 by IS6100 or △Tn2 carrying blaTEM-1B. Plasmid pK. pneumoniae HSD-KPC and the blaKPC-24-positive pUC331 demonstrated both evolutionary paths simultaneously. Interestingly, mutations in the transposase (tnpA) and tnpR genes resulted in synonymous mutations within some transposons. Three promoters, PY, PX, and P1, spanned the IRR of ISKpn27 and the non-coding region upstream of blaKPC-135. Some plasmids featured extended promoter regions that included the blaTEM-1B remnant and a putative promoter named PZ, whereas others lacked PX or P1. Mutations in the following genes encoding KPC occurred mainly in the Ω- and/or 270-loop.
Figure 4. Genetic environment of Tn6296-associated blaKPC regions. Schematic structures of Tn6296 transposons surrounding the blaKPC-135 gene in plasmid pK. pneumoniae HSD-KPC are presented, along with a comparison to related genetic elements. Plasmids encoding KPC-2 mutants are compared as follows: pK. pneumoniae HSD-KPC (Anhui, China, OP205646), pZHKPC2 (Shanghai, China, OM928503), pLWKPC (Shanghai, China, MT875328 and MT550690), pZHKPC1 (Shanghai, China, OM928502), pCK1008-KPC-123 (Hangzhou, China, ON209376), pKPC-H2 (Shanghai, China, MT550691), pR20-14 (Hangzhou, China, CP094852), pP10159-3 (Chongqing, China, MF072963), pZYPA187 (Hangzhou, China, CP133754), pE31A (Jinan, China, CP067418), and pUC331 (Temuco, Chile, KR052099). The phylogenetic tree of the blaKPC region is constructed. Resistance genes are shown in red, resolvase genes (tnpR) in green, transposase genes (tnpA) in yellow, other genes in white, inverted repeat left (IRL) and inverted repeat right (IRR) in black boxes. Among them, the tnpA of IS26, the mutated tnpA of Tn2, and mutated tnpA and tnpR of TnAs1 are presented. Shading below the nucleotide sequences indicates nucleotide identity (grey: ≥ 99%, light grey: 85%−99%). Truncated and inserted sequences are represented by ellipses dots and triangles, respectively. Comparative analysis of the alignment of the (putative) promoter regions of blaKPC isoforms and the amino acids they encode. Highlighted regions include the putative – 35 and – 10 boxes of the promoters, the transcription start site (TSS, + 1), the putative ribosome binding site (RBS), the blaKPC gene start codon (ATG) and the truncated blaTEM-1B gene. The alignment quality of the KPC is based on Blosum62 scores.
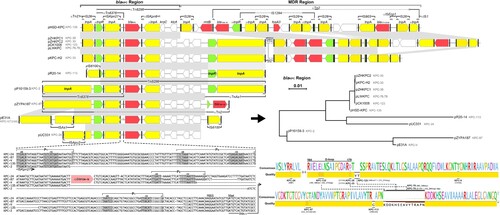
The MDR region of plasmid pK. pneumoniae HSD-KPC comprised IS26, rmtB, and △Tn2. Tn2, a member of Tn3-family transposons, which was interspersed with an IS1294-IS26 chimera and an IS26-IS903-blaCTX-M-65-△ISEcp1-IS26 unit. These MDR regions demonstrated considerable diversity, notably through the IS26-mediated deletion of fosA3 and blaCTX-M-65.
Virulence factors of K. pneumoniae HSD
The ST11-K47 K. pneumoniae HSD harboured multiple virulence determinants. On one hand, K. pneumoniae HSD carried a large virulence plasmid, approximately 200 kb in length (Figure S1). It was an IncHI1B/FIB-type plasmid containing genes associated with heavy metal resistance (copper, silver) and virulence genes that encoded capsular polysaccharide synthesis regulators (rmpA2) and iron acquisition systems (iucABCD-iutA). This plasmid, similar to the widely distributed pLVPK-like plasmids (reference sequence GenBank accession number CP094941), lacked a ∼25-kb pathogenicity island comprising rmpA, fecAIR and iroBCDN. On the other hand, the bacterial chromosome possessed the fim and mrk gene clusters, which encode type 1 and 3 fimbriae, respectively. This represented the primary virulence mechanism of K. pneumoniae HSD, involving the production of fimbriae and capsules. Another chromosome-mediated virulence factor was the bacterial type VI secretion system (T6SS), which encodes proteins such as HCP, ClpV, VgrG, and ICMF. Besides, the copy number variations (CNVs) of the mrkA decreased slightly, whereas those of pgaA increased.
Discussion
The blaKPC-2-positive ST11 type CRKP strains have emerged as a formidable clonal lineage in China, posing a threat in clinical settings [Citation23,Citation24]. Ceftazidime-avibactam, an effective antibiotic, has been clinically approved in China since 2019, with a predicted increase in resistance following extensive use [Citation25]. Resistance to ceftazidime-avibactam in CRKP strains has typically been correlated with various mutations in the blaKPC-2 gene [Citation9]. More than 60% of novel KPC gene subtypes have emerged since 2019, with mutations primarily occurring in four loops surrounding the active site core: the 105-loop (Leu102 to Ser106), Ω-loop (Arg164 to Asp179), 240-loop (Cys238 to Thr243) and 270-loop (Ala267 to Ser275) [Citation10]. Amino acid substitutions in the Ω-loop of the KPC-2, particularly at position 179, are the major contributors to resistance against ceftazidime-avibactam [Citation23,Citation26]. In this study, we described KPC-135, a novel KPC variant characterized by a deletion in the Ω-loop and a long-segment tandem repeat in the 270-loop, potentially causing Ω-loop disorder and further leading to a shallower allosteric binding site and increased solvent exposure [Citation27,Citation28]. As previously reported, this KPC variant exhibited increased ceftazidime affinity and decreased avibactam binding [Citation19,Citation29]. In addition, the reduced hydrolytic activity resulting from KPC-135 contributed to restored carbapenem susceptibility. The reason why ceftazidime was the only β-lactam that showed increased resistance against the blaKPC-135-positive strain could be that ceftazidime with lower drug permeation rate has special chemical structure and such KPC variants readily “capture” ceftazidime compared with KPC-2 enzyme [Citation27,Citation30]. Ceftazidime possesses bulky R1 substituents and small pyridine R2 substituents, which increased its intrinsic rigidity and affected the two-component regulatory system, contributing to the bacterial resistance [Citation27].
The blaKPC-135 gene resided in the IncFII/IncR-type plasmid pK. pneumoniae HSD-KPC, which exhibited improved stability and reduced transmissibility compared to similar plasmids reported worldwide [Citation31]. All deletions and insertions in pK. pneumoniae HSD-KPC occurred alongside one or two tandem IS26 copies, indicating the potential for IS26-mediated transposition that might lead to diversity in resistance and transmission [Citation32]. Unlike the Tn4401 transposon, the most prevalent blaKPC-containing mobile element, the blaKPC-135 gene was located in the IS26-truncated Tn6296 transposon [Citation33]. Tn6296 originated from the Tn2-like Tn6376 upstream of blaKPC and was followed by the integration of △ISKpn6 and Tn1721-like △TnAs1 downstream of blaKPC. The Tn6296/Tn6376 chimeras might represent non-Tn4401 transposons that evolved from Tn4401 through genetic recombination. These chimeras have gradually assumed a significant role among blaKPC-containing mobile elements, particularly blaKPC-2 variants in China [Citation11,Citation31,Citation34]. Furthermore, the genetic regulators of the Tn6296-associated blaKPC variant gene might exert an effect. PY served as a core promoter of the blaKPC gene, while the deletion of the PX and P1 promoters enhanced its expression in certain strains, frequently accompanied by the loss of the blaTEM-1 remnant encoding the active site [Citation35].
Regrettably, ST11-K47 K. pneumoniae HSD possessed both virulence and resistance, culminating in its successful persistence. On one hand, K. pneumoniae HSD carried an IncHI1B/FIB-type virulence plasmid encoding rmpA2, a regulators of capsular polysaccharide synthesis, widely documented globally [Citation36]. However, the strain K. pneumoniae HSD tested negative in the string test, indicating potential insensitivity in detecting the virulence of K. pneumoniae. On the other hand, K. pneumoniae HSD extensively expressed both type 1 and 3 fimbriae. The former mediated the formation of intracellular bacterial communities, evading host innate immune responses and facilitating colonization and intracellular survival. The latter mediated the biofilm formation on both abiotic and biotic surfaces, aiding K. pneumoniae in causing respiratory tract infections, as demonstrated in this study [Citation37,Citation38]. The elevated CNV of pgaA suggested that its expression might enhance adhesion [Citation39]. In addition, T6SS is implicated in the enhanced pathogenicity of K. pneumoniae, characterized by increased antibiotic resistance and biofilm-forming ability [Citation40].
Although ST11 HvKp with capsular serotype K47 is rarely reported, it is suggested as a serotype associated with high virulence according to a previous survey [Citation41]. Concerningly, a previous study reported that ST11-K47 HvKp lacked virulence genes, while the strain in our study possessed virulence determinants, enhancing its virulence. ST11-K47 strains represented a prevalent type of Hv-CRKP (ultravirulent) and CR-HvKP (supervirulent), proving to be more pathogenic than HvKP or CRKP strains and more challenging to treat. Characteristics in strain K. pneumoniae HSD, such as biofilm formation facilitating its spread and persistence on medical equipment, indicated that it represented an evolutionary development of Hv-CRKP from CRKP through the acquisition of a pLVPK-like virulence plasmid [Citation42].
Resently, new KPC variants have continuouslly emerged worldwide, typically associated with clinical use of ceftazidime-avibactam for 9–19 days [Citation11]. Owing to the lack of effective routine detection methods, strains producing KPC variants with inconspicuous resistant characteristic are often confused with those producing KPC-2 or ESBLs, leading to potential misjudgements in treatment [Citation11]. The blaKPC-135-positive ST11-K47 K. pneumoniae carried both resistance and virulence genes, undoubtedly presenting significant challenges in clinical management.
Supplemental Material
Download PNG Image (976.6 KB)Disclosure statement
No potential conflict of interest was reported by the author(s).
Data availability statement
The genomic information of K. pneumoniae HSD has been deposited at DDBJ/ENA/GenBank under the accession number JBBVMI000000000, with the nucleotide sequence of blaKPC-135 deposited separately under accession number OP205646.1.
Additional information
Funding
References
- Fupin H, Yan G, Demei Z, et al. CHINET surveillance of antimicrobial resistance among the bacterial isolates in 2021. Chin J Infection Chemother. 2022;22(5):521–530. doi:10.16718/j.1009-7708.2022.05.001
- Paniagua-García M, Bravo-Ferrer JM, Pérez-Galera S, et al. Attributable mortality of infections caused by carbapenem-resistant Enterobacterales: results from a prospective, multinational case-control-control matched cohorts study (EURECA). Clin Microbiol Infect. 2024;30(2):223–230. doi:10.1016/j.cmi.2023.11.008
- Yang W, Ding L, Han R, et al. Current status and trends of antimicrobial resistance among clinical isolates in China: a retrospective study of CHINET from 2018 to 2022. One Health Adv. 2023;1(1):8. doi:10.1186/s44280-023-00009-9
- Han R, Shi Q, Wu S, et al. Dissemination of Carbapenemases (KPC, NDM, OXA-48, IMP, and VIM) among Carbapenem-Resistant Enterobacteriaceae Isolated from adult and children patients in China. Front Cell Infect Microbiol. 2020;10:314. doi:10.3389/fcimb.2020.00314
- van Duin D, Bonomo RA. Ceftazidime/Avibactam and Ceftolozane/Tazobactam: second-generation beta-Lactam/beta-Lactamase Inhibitor Combinations. Clin Infect Dis. 2016;63(2):234–241. doi:10.1093/cid/ciw243
- Sharma R, Park TE, Moy S. Ceftazidime-Avibactam: a novel Cephalosporin/beta-Lactamase inhibitor combination for the treatment of resistant gram-negative organisms. Clin Ther. 2016;38(3):431–444. doi:10.1016/j.clinthera.2016.01.018
- Yin D, Wu S, Yang Y, et al. Results from the China Antimicrobial Surveillance Network (CHINET) in 2017 of the In Vitro Activities of Ceftazidime-Avibactam and Ceftolozane-Tazobactam against Clinical Isolates of Enterobacteriaceae and Pseudomonas aeruginosa. Antimicrob Agents Chemother. 2019;63(4). doi:10.1128/aac.02431-18
- Guo Y, Han R, Jiang B, et al. In Vitro Activity of New β-Lactam-β-Lactamase inhibitor combinations and comparators against clinical isolates of gram-negative Bacilli: results from the China Antimicrobial Surveillance Network (CHINET) in 2019. Microbiol Spectr. 2022;10(4):e0185422. doi:10.1128/spectrum.01854-22
- Wang Y, Wang J, Wang R, et al. Resistance to ceftazidime-avibactam and underlying mechanisms. J Glob Antimicrob Resist. 2020;22:18–27. doi:10.1016/j.jgar.2019.12.009
- Hobson CA, Bonacorsi S, Jacquier H, et al. KPC Beta-Lactamases are permissive to insertions and deletions conferring substrate spectrum modifications and resistance to Ceftazidime-Avibactam. Antimicrob Agents Chemother. 2020;64(12). doi:10.1128/aac.01175-20
- Ding L, Shen S, Chen J, et al. Klebsiella pneumoniae carbapenemase variants: the new threat to global public health. Clin Microbiol Rev. 2023;36(4):e0000823. doi:10.1128/cmr.00008-23
- Tian D, Liu X, Chen W, et al. Prevalence of hypervirulent and carbapenem-resistant Klebsiella pneumoniae under divergent evolutionary patterns. Emerg Microbes Infect. 2022;11(1):1936–1949. doi:10.1080/22221751.2022.2103454
- Tian D, Wang M, Zhou Y, et al. Genetic diversity and evolution of the virulence plasmids encoding aerobactin and salmochelin in Klebsiella pneumoniae. Virulence. 2021;12(1):1323–1333. doi:10.1080/21505594.2021.1924019
- Ping L, Mavridou DA, Emberly E, et al. Vital dye reaction and granule localization in periplasm of Escherichia coli. PLoS One. 2012;7(6):e38427. doi:10.1371/journal.pone.0038427
- Clinical and Laboratory Standards Institute. Performance standards for antimicrobial susceptibility testing. 33th ed. Wayne (PA): CLSI supplement M100. Clinical and Laboratory Standards Institute; 2023.
- The European Committee on Antimicrobial Susceptibility Testing. Breakpoint tables for interpretation of MICs and zone diameters. Version 14.0, 2024. https://www.eucast.org/clinical_breakpoints.
- U.S. Food and Drug Administration. Tigecycline-Injection products; 2023. available at https://www.fda.gov/drugs/development-resources/tigecycline-injection-products.
- Poirel L, Walsh TR, Cuvillier V, et al. Multiplex PCR for detection of acquired carbapenemase genes. Diagn Microbiol Infect Dis. 2011;70(1):119–123. doi:10.1016/j.diagmicrobio.2010.12.002
- Shi Q, Han R, Guo Y, et al. Multiple Novel Ceftazidime-Avibactam-Resistant Variants of blaKPC-2-Positive Klebsiella pneumoniae in two patients. Microbiol Spectr. 2022;10(3):e0171421. doi:10.1128/spectrum.01714-21
- Li X, Quan J, Ke H, et al. Emergence of a KPC variant conferring resistance to Ceftazidime-Avibactam in a Widespread ST11 Carbapenem-Resistant Klebsiella pneumoniae Clone in China. Front Microbiol. 2021;12:724272. doi:10.3389/fmicb.2021.724272
- Li X, Ke H, Wu W, et al. Molecular mechanisms driving the In Vivo Development of KPC-71-mediated resistance to Ceftazidime-Avibactam during Treatment of Carbapenem-Resistant Klebsiella pneumoniae Infections. mSphere. 2021;6(6):e0085921. doi:10.1128/mSphere.00859-21
- DelaFuente J, Rodriguez-Beltran J, San Millan A. Methods to study fitness and compensatory adaptation in plasmid-carrying bacteria. Methods Mol Biol. 2020;2075:371–382. doi:10.1007/978-1-4939-9877-7_26
- Shi Q, Yin D, Han R, et al. Emergence and recovery of Ceftazidime-avibactam resistance in blaKPC-33-Harboring Klebsiella pneumoniae sequence Type 11 isolates in China. Clin Infect Dis. 2020;71(Supplement_4):S436–s439. doi:10.1093/cid/ciaa1521
- Huang X, Shen S, Chang F, et al. Emergence of KPC-134, a KPC-2 variant associated with ceftazidime-avibactam resistance in a ST11 Klebsiella pneumoniae clinical strain. Microbiol Spectr. 2023;11(5):e0072523. doi:10.1128/spectrum.00725-23
- Dietl B, Martínez LM, Calbo E, et al. Update on the role of ceftazidime-avibactam in the management of carbapenemase-producing Enterobacterales. Future Microbiol. 2020;15:473–484. doi:10.2217/fmb-2020-0012
- Li D, Li K, Dong H, et al. Ceftazidime-Avibactam resistance in Klebsiella pneumoniae Sequence Type 11 Due to a Mutation in Plasmid-Borne blaKPC-2 to blaKPC-33, in Henan, China. Infect Drug Resist. 2021;14:1725–1731. doi:10.2147/idr.S306095
- Alsenani TA, Viviani SL, Kumar V, et al. Structural characterization of the D179N and D179Y Variants of KPC-2 β-Lactamase: Ω-loop destabilization as a mechanism of resistance to Ceftazidime-Avibactam. Antimicrob Agents Chemother. 2022;66(4):e0241421. doi:10.1128/aac.02414-21
- Galdadas I, Qu S, Oliveira ASF, et al. Allosteric communication in class A β-lactamases occurs via cooperative coupling of loop dynamics. Elife. 2021: 10. doi:10.7554/eLife.66567
- Winkler ML, Papp-Wallace KM, Bonomo RA. Activity of ceftazidime/avibactam against isogenic strains of Escherichia coli containing KPC and SHV beta-lactamases with single amino acid substitutions in the Omega-loop. J Antimicrob Chemother. 2015;70(8):2279–2286. doi:10.1093/jac/dkv094
- Barnes MD, Winkler ML, Taracila MA, et al. Klebsiella pneumoniae Carbapenemase-2 (KPC-2), Substitutions at Ambler Position Asp179, and resistance to Ceftazidime-Avibactam: unique antibiotic-resistant phenotypes emerge from β-Lactamase protein engineering. mBio. 2017;8(5):e00528–17. doi:10.1128/mBio.00528-17
- Chen L, Mathema B, Chavda KD, et al. Carbapenemase-producing Klebsiella pneumoniae: molecular and genetic decoding. Trends Microbiol. 2014;22(12):686–696. doi:10.1016/j.tim.2014.09.003
- Varani A, He S, Siguier P, et al. The IS6 family, a clinically important group of insertion sequences including IS26. Mob DNA. 2021;12(1):11. doi:10.1186/s13100-021-00239-x
- Zhang X, Li F, Cui S, et al. Prevalence and distribution characteristics of blaKPC-2 and blaNDM-1 Genes in Klebsiella pneumoniae. Infect Drug Resist. 2020;13:2901–2910. doi:10.2147/idr.S253631
- Shi L, Feng J, Zhan Z, et al. Comparative analysis of blaKPC-2- and rmtB-carrying IncFII-family pKPC-LK30/pHN7A8 hybrid plasmids from Klebsiella pneumoniae CG258 strains disseminated among multiple Chinese hospitals. Infect Drug Resist. 2018;11:1783–1793. doi:10.2147/idr.S171953
- Huang J, Hu X, Zhao Y, et al. Genetic factors associated with enhanced blaKPC Expression in Tn3/Tn4401 Chimeras. Antimicrob Agents Chemother. 2020;64(3). doi:10.1128/aac.01836-19
- Dong N, Yang X, Zhang R, et al. Tracking microevolution events among ST11 carbapenemase-producing hypervirulent Klebsiella pneumoniae outbreak strains. Emerg Microbes Infect. 2018;7(1):146. doi:10.1038/s41426-018-0146-6
- Zhu J, Wang T, Chen L, et al. Virulence factors in Hypervirulent Klebsiella pneumoniae. Front Microbiol. 2021;12:642484. doi:10.3389/fmicb.2021.642484
- Hornick DB, Allen BL, Horn MA, et al. Adherence to respiratory epithelia by recombinant Escherichia coli expressing Klebsiella pneumoniae type 3 fimbrial gene products. Infect Immun. 1992;60(4):1577–1588. doi:10.1128/iai.60.4.1577-1588.1992
- D'Apolito D, Arena F, Conte V, et al. Phenotypical and molecular assessment of the virulence potential of KPC-3-producing Klebsiella pneumoniae ST392 clinical isolates. Microbiol Res. 2020;240:126551. doi:10.1016/j.micres.2020.126551
- Mohamed NA, Alrawy MH, Makbol RM, et al. Type VI secretion system (T6SS) in Klebsiella pneumoniae, relation to antibiotic resistance and biofilm formation. Iran J Microbiol. 2023;15(5):601–608. doi:10.18502/ijm.v15i5.13865
- Yang Q, Jia X, Zhou M, et al. Emergence of ST11-K47 and ST11-K64 hypervirulent carbapenem-resistant Klebsiella pneumoniae in bacterial liver abscesses from China: a molecular, biological, and epidemiological study. Emerg Microbes Infect. 2020;9(1):320–331. doi:10.1080/22221751.2020.1721334
- Douradinha B. Should multidrug resistant Klebsiella pneumoniae strains displaying hypervirulent traits be reclassified as either ultravirulent or supervirulent? Microbiol Res. 2023;275:127446. doi:10.1016/j.micres.2023.127446