ABSTRACT
Carmoisine is a synthetic food additive which is commonly used in a wide range of food beverages. According to reports, excessive use of this coloring agent causes anxiogenic behavior in mice by inducing oxidative stress. The purpose of this study was to investigate the ameliorating effects of Vernonia Cinerea against carmoisine-induced brain injury and anxiogenic-like effects in mice. Results of behavioral studies revealed that Ethanol Extract of Vernonia Cinerea (EEVC) has anxiolytic activity. Furthermore, carmoisine administration resulted in a significant increase in neuro-chemicals such as glutamate and a depletion of Gamma-Amino Butyric Acid levels (p < 0.001) and antioxidant enzymes such as catalase, and superoxide dismutase levels (p < 0.05), confirming brain tissue damage, while EEVC administration (400 mg/kg p.o.) reversed these biochemical alterations significantly (p < 0.05) as compared to the normal control and carmoisine-treated groups. To a remarkable extent, the extract causes significant changes in neurochemicals. Furthermore, EEVC administration significantly increased the levels of various in vivo antioxidants. Furthermore, EEVC reversed hippocampus Cornu Ammonis 3 (CA3) area complications such as intense vocalization, cyanosis, and hemorrhage when compared to carmoisine groups. From the results, EEVC has shown significant anxiolytic and ameliorating potential against carmoisine-induced brain injury evidenced by a significant reversal of biochemical, neurochemical parameters along with significant improvement of antioxidant activities.
Introduction
Azo dyes have been used extensively in the cosmetics, leather, food, textile, paper, and pharmaceuticals. Carmoisine is one of the most important food additives among the all-azo dyes, and it is widely used in a variety of food beverages [Citation1,Citation2]. To increase the aesthetic content of food preparations, a number of artificial food colors are often incorporated. Some of the permitted organic synthetic dyes in synthetic food additives include sunset yellow, orange GGN, carmoisine, dazzling blue, tartrazine, erythrosine, ponceau 4 R, scarlet GN, allure red and quick red E. Because of their coloring properties, stability, low cost, and uniformity, artificial dyes are generally used. Long-term use of this synthetic food dyes often induces anemia, indigestion, and anomalies in offspring, allergic reactions such as asthma and urticaria, tumors and cancer paralysis, inflammatory lesions in the kidney, brain, spleen, and liver, and eye disorders in the majority of individuals, resulting in blindness, growth retardation, and mental retardation [Citation3,Citation4]. Between the biochemical pathways that are likely to be damaged by the oxidant effect, including cellular damage induced by the ingestion of these food dyes [Citation5].
Carmoisine exposure at higher doses reduces overall antioxidant capacity as well as biochemical parameters of dopamine and serotonin levels. It did, however, elevate the lipid peroxidation marker malonaldehyde (MDA), upregulate the mRNA expression of pro-apoptotic genes (Bax, Fas, casp3, and Fas-L), and lower the transcriptional level of the anti-apoptotic gene Bcl2. Furthermore, upregulating miR-140 and miR-34a expression decreased the expression of their target genes NRF-2 and SIRT-1, respectively. As a result, excessive oral intake of carmoisine causes brain apoptosis effect, and caution should be exercised while using it [Citation6].
It also causes changes in neurobehavioral patterns via oxidative stress. Furthermore, releasing reactive oxygen species from food dye causes the oxidative stress-induced detrimental effect on cellular events. The central nervous system is impaired by oxidative degradation in the brain. Studies performed in recent years suggest, oxidative stress can contribute to elevated anxiety levels and lower the antioxidant levels [Citation7]. However, further research is needed to reverse the neurobehavioral changes and oxidative stress due to food dyes in the brain. Furthermore, the majority of clinically available anti-anxiety medications are effective in anxiety patients. However, it is overburdened by side effects, slow onset of action, and poor patient compliance [Citation8,Citation9].
Herbal products are now thought to be safe and healthy for human consumption, having resolved the negative effects of synthetic dye [Citation10]. Novel antioxidants can provide an efficient and safe way for the human bodies to strengthen themselves by combating oxidants. Human brain cells are capable of fighting free radicals with a limited number of resources. Plant-derived antioxidants are more protective against oxidative stress reactions [Citation11,Citation12]. Vernonia cinerea plant is a part of the Asteraceae family, and the whole plant is often used to treat headaches, fever, dyspepsia, swelling, dysentery, fractures, and infection, as well as acting as an antioxidant, tranquilizer, and sedative [Citation13]. According to the earlier research reports, Vernonia cinerea’s pharmacological activities are associated with a variety of phyto-constituents such as phenolic resins, flavonoids, glycosides, and triterpenoids [Citation14].Vernonia Cinerea’s sedative properties are attributed to it being used in a number of traditional herbal remedies for insomnia and related ailments [Citation15]. This plant-treated rat has a depressive effect on the central nervous system. This neurological mechanism corroborates current study into the plant’s anti-anxiety properties [Citation16].
As a result of the aforementioned data, the current study was designed to establish the anxiety model for rodent and to use a potential novel extract of Vernonia cinerea against carmoisine-induced brain injury and anxiogenic like effect in mice by combating oxidative damage.
Methods
Materials
Carmoisine (Himedia Labs Ltd., Chennai) is a food dye that has been used as an anxiogenic agent. For lipid peroxidation activity, sodium dodecyl sulfate (SDS) (8.1%), thio barbituric acid (TBA) (0.8%), acetic acid (20%; pH 3.5), n-butanol, and Pyridine (Qualgens Fine Chemicals, Mumbai) were used. For Superoxide dismutase activity, carbonate buffer (100 mM, pH 10.2) and epinephrine (3 mM) (Maharashtra Himedia Labs Ltd) were used. Catalase activity is measured using potassium dihydrogen phosphate (KH2PO4), sodium hydrogen phosphate (Na2HPO4), 30% hydrogen peroxide (H2O2), and phosphate buffer (S.D. Fine Chemicals Ltd, Mumbai). For the determination of neurochemical levels, GABA, Ninhydrin reagent, Glutamate, Ethanol, and Silica gel (Sigma Aldrich, USA) were used. Methanol, Aluminum chloride, Potassium acetate, Quercetin, Folin-Ciocalteu reagent (Himedia Labs Ltd Chennai) was used for total phenols and flavonoids estimation.
Plant material and preparation of their extract
Vernonia cinerea was collected in Tirumala hills, Andhra Pradesh, from the Eastern Ghats in the Seshachalam range and at a location with the coordinates 14.3333°N 78.2500°E and taxonomic authentication No: TD 107 dated 16 November 2018 received from the Department of Botany, S.V University, Tirupati, India. This herb’s aerial portion was shade dried before being pulverized into a coarse powder. In the Soxhlet apparatus, 300 gm of plant pulverized materials is Soxhlet with 2.5 L of 90% ethanol. The solvent was extracted by distillation at low pressure, resulting in a green sticky residual formation. The extracted material achieved a final yield of 10% w/w [Citation17].
Determination of phenols and flavonoids in EEVC
Spectrophotometric quantification of total flavonoids and phenols were determined using the aluminum chloride colorimetric method and Folin-Ciocalteu’s reagent method, respectively [Citation18–20].
Animals
Twenty Swiss albino male mice (20–25 g) were used in the research and were placed in the dark and light cycles for 12 hours each. Mice were given free outpouring water and pellets of mice feed while maintaining a relative humidity of 55 ± 10% and a stable temperature (21 ± 2°C). All of the animals were kept in polypropylene cage for acclimatization period of about 10 days. The experimental protocols were reviewed and approved by the Institutional Animal Ethics Committee (IAEC), with the protocol number SVCP/IAEC/1-005/2018-19 dated 01/04/2019.
Pharmacological evaluation of EEVC
The Joint FAO/WHO Expert Committee on Food Additives (JECFA) established the acceptable daily intake of carmoisine as 0–4 mg/kg. The disturbance of neurochemicals and oxidative stress in brain cells occurred due to overexposure or repeated exposure to this food color. [Citation21 & Citation2]. Based on this review, carmoisine was administered to mice at doses equivalent to 5 times the ADI (20 mg/kg) in the current study. Based on previous research, we chose the plant extract EEVC 400 mg/kg p.o. and Diazepam 2 mg/kg p.o. [B] were used to treat the animals [Citation22].
In this research, 20 Albino mice (25–30 g) were used. The mice were categorized into four groups of five animals each (n = 5) at random basis. The groups are as follows: Group I was given Tween 20, (10 ml/kg p.o); Group II was given carmoisineat a dosage of 20 mg/kg p.o.; Group III was given carmoisine 20 mg/kg p.o. and Diazepam 2 mg/kg p.o.; Group IV was given carmoisine 20 mg/kg p.o. plus EEVC 400 mg/kg p.o. Carmoisine and drugs were administered for 30 days to the animals. At the end of the study the animals’ behavior were assessed using the Elevated maze test (EMT), Open field test (OFT), light/dark model test, loco-motor activity test and Rota-rod test by following standard procedures. Finally the animals were sacrificed and the whole brains were collected for preparation of tissue homogenate in order to estimate the biochemical, neurochemical, and antioxidant parameters. Part of the brain samples were used for histopathological analysis using hematoxylin and eosin staining.
Acute oral toxicity study
The acute oral toxicity assessment followed the guidelines laid out by the Organization for Economic Cooperation and Development. Six female rats weighing 130–160 g were divided into 2 groups. Each group consists of three animals: a control group and a test group. The control group received 10 ml/kg b.wt of distilled water as a vehicle, while the test groups received an oral dose of 2000 mg/kg of EEVC. All the experimental animals were observed for their mortality and clinical signs of toxicity (general behavior, respiratory patterns, cardiovascular signs, motor activities, reflexes, and changes in skin and fur texture) at 30 min, 1, 2, and 4 h and thereafter once a day for 14 days. Once a week, body weights were taken. On the 15th day, the overnight fasting rats were euthanized using a CO2 euthanasia chamber and all major internal organs, including the brain, heart, lung, liver, kidney, spleen, adrenals, and sex organs, were morphologically examined. The LD50 cutoff values of EEVC were obtained using the Globally Harmonized System of Classification and Labeling of Chemicals [Citation23].
Behavioral assessments
Elevated plus-maze
The equipment was lifted to a height of 25 cm above the floor. Each mouse was placed in the center of the apparatus, one by one, with its head facing toward an open arm, and the behavior was recorded for the next 5 minutes, primarily the time spent and the number of entries in each arm.
Open field exploratory test
The open-field experiment was used to evaluate rodent anxiety behavior. The apparatus is made up of 16 quadratic blocks with thin white lines running across the surface. The mice were placed in the center of the arena for 5 minutes whereas the researchers observed their ambulatory locomotion. The number of rearing peripheral and center crossings was counted.
Light/dark exploration task
Using an additional light source, the white section of the equipment was 1000 lux, and the black section was 50 lux. Mice were placed in the center of the light portion, facing the opening, and the animal was examined for the next 5 minutes for the passage between the dark and light (open/white) sections.
Calculate the percentage of time spent in the light portion.
Time spent = (Number of seconds spent in the light compartment divided by 300 seconds) X100
Loco motor performance test
Activity cage are made up of photoelectric cells that are linked together in a circuit with a counter. Each mouse was placed in the Activity cage for 5 minutes, and during that time the mice’s overall activity score was calculated.
Measurement of muscle coordination
For measuring animal motor coordination function, a metal rod was used to ride the mice on the Rota Rod apparatus for 300 seconds at a speed of 12 rpm. The fall-off time was measured at intervals of 30, 60, and 90 minutes.
Biochemical estimation
Brain homogenate preparation
Mice were sacrificed, and the hippocampus of their brains was gathered. The hippocampus of the brain was washed with ice-cold 0.9% NaCl and measured. 0.1 M Phosphate buffer was used to treat a 10% (w/v) homogenate of brain tissue (pH 7.4). The supernatants from this mixture were collected for biochemical analysis after samples were centrifuged at 12,000 g for 20 minutes at 4°C.
Superoxide dismutase and catalase level measurements
The obtained supernatants from brain tissue homogenate were used to measure the Superoxide dismutase and Catalase activity as per the standard procedure. The absorbance of reaction mixture and standard was measured at 480 nm for Superoxide dismutase and 240 nm for Catalase using spectrophotometer. The results were expressed as U/mg Protein [Citation24,Citation25].
Lipid peroxidation level measurement
Thio Barbituric acid activity was measured as per the standard protocols. In brief, 0.2 ml of brain homogenate was mixed with 1.5 ml of 20% acetic acid, 0.8% (W/V) of 1.5 ml of Thio Barbituric acid, and 0.2 ml of 8.1% (W/V) of sodium dodecyl sulfate in a test tube, vortexed vigorously, heated in a water bath for 1 h at 95°C, and then instantly cooled. The mixture was then vigorously shaken with 1 mL of water and 5 mL of a 15:1 V/V n-butanol/pyridine mixture. The supernatants were collected and the absorbance at 523 nm was measured after centrifuging the mixture for 10 minutes at 4000 rpm [Citation26].
Brain glutamate and gamma-amino butyric acid levels measurement
The levels of the brain neurochemicals such as glutamate and Gamma-Aminobutyric Acid are measured using standard methods and the results were expressed as mmol/mg tissue [Citation27].
Histological analysis
The brain tissue was embedded in paraffin after being preserved in a 10% neutral formaldehyde solution. For microscopic examination, 7 mm thick paraffin sections were prepared. Finally, tissues were dipped in water, and they were stained with hematoxylin and eosin at 60°C for 10 minutes. The dyed part was washed in tap water to remove the extra stain upon being upgraded with alcohol for dehydration purposes. To make the section slides final, they were washed with xylene and stored in DPX. The slides were examined under a Nikon 80 I at 400x and the histopathological changes were observed [Citation28].
Statistical analysis
Graph Pad Prism 5.0 Version analysis (Graph Pad Software, Inc., San Diego, California, USA) was used for the statistical. All data are provided as Mean Standard Error Mean (SEM), and measurements are made using one-way (Analysis of Variance) ANOVA, with Tukey’s test as a post hoc test. Statistical significance was expressed with p < 0.05, p < 0.01, and p < 0.001.
Results
Acute oral toxicity study
The control rats showed no signs of toxicity or treatment-related fatalities, while the EEVC-treated animals survived the study. The predominant behavioral signs of toxicity in the rats were restlessness and wiping their noses and mouths on the cage floor, which disappeared 24 hours after extract administration. Throughout the trial, there was no discernible difference in body weight between the treatment and control groups of rats. Furthermore, there were no obvious cross-pathological abnormalities in either the control or treated rats.
Spectrophotometric quantification of flavonoids and phenols in EEVC
Phenolic and flavonoid compounds are antioxidants that serve as free radical scavengers. The flavonoid content of the extract was 11.3 ± 0.12 mg/g in terms of standard Quercetin (the standard curve equation: y = 0.008x + 0.005, R2 = 0.996). and also demonstrated that the total phenols present in the EEVC is 21.4 ± 0.54 mg/g, as determined by the Folin Ciocalteu reagent in terms of Gallic acid equivalent (standard curve equation: y = 0.003x + 0.002, R2 = 0.998). The extract of EEVC with the highest concentration of flavonoid and phenolic compounds showed highest antioxidant potential.
Behavior assessments
Effects of EEVC on mouse behavior
As contrast to the control group, carmoisine-treated mice had a significant (p < 0.001) reduction in the number of central and peripheral crossings. The EEVC treatment dosage (400 mg/kg, p.o) significantly increased the level of peripheral, central crossing (p < 0.001), while diazepam (2 mg/kg, p.o) showed a highly significant effect on these parameters .
Table 1. Effect of EEVC on mouse anxiogenic behavior using open field and elevated plus maze test.
EMT data analysis showed that carnosine caused anxiety-like behavior, as shown by a significant reduction in the time spent in open arms (p < 0.001) as compared to the control group. EEVC-treated mice reversed carmoisine-induced modifications in EMT parameters. As compared to carnosine 20 mg/kg, p.o treated mice; EEVC (400 mg/kg) significantly (p < 0.001) increased the time spent in the open arm .
Effects of EEVC on mouse behavior inlight/ dark exploration task
Data analysis of light/dark exploration showed that carmoisine caused anxiety animals, as shown by a significant (p < 0.01) decrease in time spent in a light chamber and a significant (p < 0.001) increase in the number of crossings (p < 0.001) between the light and dark chambers. Simultaneously, the time spent in the dark chamber was significantly increased. The EEVC treatment dosage (400 mg/kg, po) and diazepam treated mice (2 mg/kg) led to a significantly (p < 0.001) reverse the aforementioned effects. But a non-significant effect on time spent in the light chamber was observed in diazepam treated group .
Table 2. Effect of EEVC on mouse behavior on light/dark exploration task, locomotor, and motor coordination test.
Effects of EEVC on loco-motor and motor coordination efficiency
As compared to the normal control group, carmoisine-treated mice exhibited a significant increase in the locomotor activity (p < 0.001). The EEVC treatment dose (400 mg/kg, p.o.) resulted in a significant (p < 0.001) reduction in spontaneous locomotor function. Carnosine treatment had no effect on falling time as compared to the normal control group. Our findings indicate that the fall in time from the Rota rod caused by EEVC (400 mg/kg, p.o) was not significant as compared to carnosine treated mice. Whereas diazepam treated mice (2 mg/kg) had a more significant impact in locomotor and fall off time (p < 0.001) when compared to the usual control group .
Biochemical estimation
Effects of EEVC on brain catalase, superoxide dismutase, and thio barbituric acid levels
From the results, Catalase and Superoxide dismutase levels were found to be depleted in the hippocampal area of carmoisine-treated mice, as well as a significant (p < 0.001) increase in hippocampal segment thio Barbituric acid values (). shows that EEVC (400 mg/kg, p.o.) and carmoisine treated mice hippocampal sections resulted in a significant (p < 0.001) decrease in Catalase, Superoxide dismutase, and thio Barbituric acid levels. Diazepam (2 mg/kg) significantly (p < 0.001) increases Catalase and Superoxide dismutase levels in the hippocampal section of carmoisine-treated animals, while decreasing thio Barbituric acid levels significantly (p < 0.001).
Figure 1. Effects EEVC on brain (A) Catalase levels; (B) Superoxide dismutase levels; (C) Thiobarbituric acids; (D) Glutamate levels; (E) GABA levels after 30 days of treatments. Values are expressed as Mean ± SEM. (n = 5); *p < 0.05, ** p < 0.01, ***p < 0.001 compared with normal control. #p < 0.05, ## p < 0.01, ###p < 0.001 compared with CR control. Statistical test employed was one way ANOVA followed by Tukey multiple comparison test.
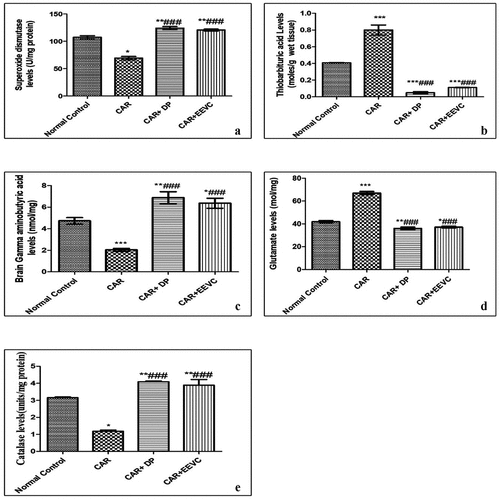
Figure 2. The impact of EEVC on mouse CA3 region of the brain’s hippocampus against the carmoisine induced anxiety.(A) Control – 1% tween 20 (10 ml/kg, p.o), The results showed that control mice had normal architecture cells in the CA3 region of the hippocampus; (B) CR (20 mg/kg, p.o), carmoisine treated groups had Severe vocalization(black arrows), Pkynosis (green arrow), Hemorrhage (Blue arrow), Autolytic cell (yellow arrow); (C) CR (20 mg/ kg, p.o))+ DP(1 mg/ kg, p.o), Treatment of diazepam along with carmosiine treated mice regeneration of neurone (Violet arrows), less perivascular edema (Green arrow), less vocalization (Black arrows); (D) CR (20 mg/kg, p.o)+ EEVC (400 mg/ kg, p.o). Treatment of EEVC along with carmoisne treated mice regeneration of neurone (Violet arrows), less perivascular edema (Green arrow), less vocalization (Black arrow).
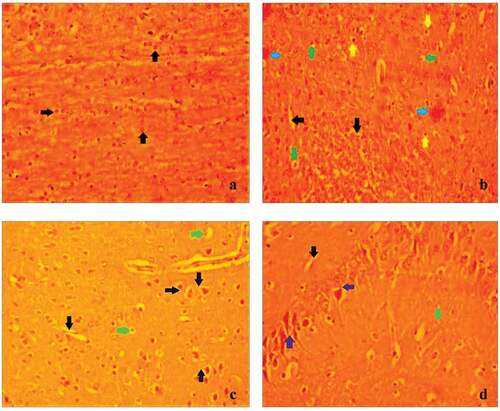
Effects of EEVC on mouse brain glutamate and gamma-amino butyric acid neurochemical level
From the results, a significant (p < 0.001) notable increase in glutamate levels and a decrease in Gamma-Amino butyric acid levels were seen after carmoisine administration. Glutamate and Gamma-Amino butyric acid levels in the carmoisine group are altered by the administration of EEVC (400 mg/kg, p.o.). show that diazepam (2 mg/kg) had a more notable impact on these alterations.
Discussions
Several studies have found that EEVC has significant antioxidant properties that protect against oxidant-induced diseases and neurological reactions caused by oxidative stress [Citation17,Citation29]. The phenolic compounds, which include flavonoids, are responsible for a broad range of biological activities such as antioxidant, antimutagenic, and anticancer properties [Citation30]. Many phenolic compounds, flavonoids, and steroids have been identified in Vernonia cinerea extract [Citation31]. These above data support the present study; UV spectrophotometric result shows that the total flavonoid and phenolic content in EEVC were found to be 11.3 ± 0.12 mg/g and 21.4 ± 0.54 mg/g, respectively. These correlations indicate that the extract contains neuroprotective and antioxidant phytoconstituents flavonoids and phenolic compounds.
According to the Globally Harmonized System of Classification and Labeling of Chemicals, there was no mortality or toxicity signs observed at 2000 mg/kg of EEVC, so it can be classified as category 5 and the LD50 value was greater than 2000 mg/kg, that provides a guideline for selecting the dose for pharmacological evaluation of this plant. The most widely used methods to evaluate the effects of anxiolytics on animal behavior parameters are the EMT and OFT [Citation32]. Carmoisine mediated anxiety behavior in the current study, as demonstrated by a significant reduction in the parameter in EMT, that is, time spent in open arm. These findings are consistent with previous research [Citation33,Citation34]. OFT carmoisine-treated rodents exhibit a decline in central and peripheral crossing, indicating the animal’s fear or anxiety. Similarly, EEVC-treated mice increased the number of crossings between the center and the periphery. The observed result suggests that the EEVC has an anxiolytic property against carmoisine-induced anxiety effects.
The light–dark box test is focused on rodents’ natural aversion to brightly lit environments. The test is helpful in predicting whether the studied animals had anxiolytic or anxiogenic activity. This result remain that the extract has anxiolytic-like action. Even after carmoisine injections, the animals tended to spend more time in the dark zone than in the light zones, confirming their aversion to brightly illuminated areas. When compared to carmoisine-treated mice, the EEVC (400 mg/kg) significantly increased time was spent in the light compartment of the light/dark test. As a result, it indicates that EEVC has a strong anxiolytic effect on laboratory animals.
The actophotometer is a common screening tool for assessing locomotor function. Anxiety is associated with elevated muscle tone, as well as other symptoms such as restlessness or becoming agitated, quickly fatigued, irritability, increased muscle stiffness, and sleep disruption. The significant decline in EEVC locomotor function may be attributed to the extract’s inhibitory effects on the Central Nervous System. The extract’s sedative impact may be responsible for the decrease of spontaneous motor activity [Citation33]. The EEVC 400 mg/kg treated mice greatly enhancing the efficacy of pentobarbital mediated narcosis with an improvement in sleeping time of laboratory animals was recorded and several scientific types of literature available the Vernonia cinerea plant possesses the sedative and CNS depressant effect [Citation35,Citation36].
The Rota rod performance of mice is a sensitive and commonly used method for assessing balance and coordination aspects of motor control [Citation37,Citation38]. Thus, muscle coordination and balance in the fore and hind limbs may be studied. This role necessitates normal cerebellar activity as well as muscle coordination [Citation39]. Mice with extreme motor coordination concerns will struggle to remain on the rotating rod. The extract had no effect on the duration of permanence on the bar in the Rota rod test, indicating the lack of defective motor skills. Furthermore, the extract’s inhibitory action could be elicited by central pathways rather than peripheral neuromuscular blockade [Citation40]. In comparison to EEVC, Diazepam greatly reduced this parameter, suggesting reduced the motor coordination. According to the literature, benzodiazepines, such as diazepam, serve as anxiolytics (at low levels) and anticonvulsants, while also having a myorelaxant reaction at higher doses [Citation41,Citation42]. This is unsurprising given that diazepam has a myorelaxant effect at the dosage used.
Accumulation of reactive oxygen species caused by stress can lead to the degradation of cell components, an inappropriate process for repairing Deoxyribo Nucleic Acid (DNA), and mitochondrial dysfunction that also can be important factors in the development of neurological disorders and aging [Citation43]. As a consequences, several kinds of research were currently being conducted in order to find a better therapeutic agent to protect the aging and neurological disorders from free radical disruption. In the present investigation, the EEVC extract reduced Lipid Peroxidation (LPO) whereas increasing antioxidant enzymes such as superoxide dismutase and catalase. The antioxidant action of Vernonia cinerea against oxidative stress has already been documented in the scientific literature [Citation44].
GABA is well known in the mammalian central nervous system as the key inhibitory neurotransmitter. It has been shown to play a crucial role in promoting neuronal regeneration, modulating synaptic transmission, preventing sleep loss, depression, and anxiety [Citation45]. Numerous research findings have shown that dysregulation of centrally functioning glutamate has been linked to symptoms of anxiety, post-traumatic stress disorder, obsessive-compulsive disorder (OCD), mania, depression, and psychotic [Citation46].
An imbalance in the neurochemicals GABA and Glutamate, can trigger neuropsychiatric and neurodegenerative conditions [Citation47]. Many medicinal plants have sedative and anxiolytic properties by increasing inhibitory neurotransmission or reducing excitatory neurotransmission [Citation48]. Carmoisine-treated mice received EEVC extract, which resulted in a significant increase in GABA and a decrease in glutamate levels. As a result, the current study found that the extract has a stronger GABA mimic effect against carmoisine-induced anxiety in animals.
The study found that control mice had the normal histological structure of the hippocampus’s CA3 area. In the CA3 region of the hippocampus, the carmoisine-treated group has seen a significant increase in neuronal death, extreme vacuolization, pyknosis, and bleeding. Though co-treatment of EEVC with carmoisine and diazepam with carmoisine substantially reduced neuronal cell damage in the CA3 region compared to the carmoisine-treated group, this indicates that EEVC can effectively make neuroprotective effects against the deleterious effects of carmoisine-mediated histological abnormalities by ameliorating neuronal loss in mice brain. EEVC along with carmoisine protected the CA3 area considerably less than diazepam along with carmoisine treated mice.
But apart from that, all groups had statistical significance. Many studies have shown that ethno medicinal plants containing flavonoids, phenolic, tannins, and saponins are effective in treating neurological disorders [Citation49]. Study results suggested our findings that the Vernonia cinerea is a possible free radical scavenger due to its high phenolic and flavonoid content [Citation50–52]. In the current research, phytochemical analyses of EEVC extract reported the existence of phenolic compounds, glycosides, flavonoids, and saponins. As a result of the existence of these phytoconstituents of the plant, this anxiolytic effect, as well as their free radical scavenging effect, can exist.
Conclusions
The synthetic food azo dye Carmoisine can produce oxidant stress and negatively impact biochemical markers in the brain by forming oxidants. According to the findings of the present study, EEVC possess significant anxiolytic and ameliorative potential against carmoisine-induced brain injury, as demonstrated by a significant reversal of biochemical, neurochemical parameters as well as a significant increase in antioxidant activities. Hence, it can be concluded that, the protective potential of EEVC may be due to attenuation of biochemical, neurochemical parameters and oxidative stress by combating oxidative stress through reversal in vivo antioxidants.
Disclosure statement
No potential conflict of interest was reported by the author(s).
Additional information
Funding
References
- Mansour HB, Corroler D, Barillier D, et al. Evaluation of genotoxicity and pro-oxidant effect of the azo dyes: acids yellow 17, violet 7 and Orange 52, and of their degradation products by Pseudomonas putida mt-2. Food Chem Toxic. 2007;45(9):1670–1677.
- Khiralla G, Salem S, El-Malky W. Effect of natural and synthetic food coloring agents on the balance of some hormones in rats. J Food Sci Nut. 2015;5(2):88–95.
- Ashida H, Hashimoto T, Tsuji S, et al. Synergistic effects of food colors on the toxicity of 3-amino-1, 4-dimethyl-5H-pyrido [4, 3-b] indole (Trp-P-1) in primary cultured rat hepatocytes. J Nut Sci Vitamin. 2000;46(3):130–136.
- Kroes R, Kozianowski G. Threshold of toxicological concern (TTC) in food safety assessment. Toxic Lett. 2002;127(1–3):43–46.
- Halliwell B. Antioxidants and human disease: a general introduction. Nut Rev. 2009;55(1):S44–S49.
- Hussein M, Mahmoud E, Abdo S. Mir-140 and Mir-34a as molecular markers for apoptotic brain in sunset yellow and carmoisine intoxicated mice. Zagazig Vet J. 2021;49(2):37–49.
- Kumar A, Garg R, Prakash AK. Effect of St. John’s Wort (Hypericum perforatum) treatment on restraint stress-induced behavioral and biochemical alteration in mice. BMC Complement Alter Med. 2010;10(1):18.
- Mesfin M, Asres K, Shibeshi W. Evaluation of anxiolytic activity of the essential oil of the aerial part ofFoeniculum vulgare Miller in mice. BMC Complement Alter Med. 2014;14(1):310.
- Sulakhiya K, Kumar P, Jangra A, et al. Honokiol abrogates lipopolysaccharide-induced depressive like behavior by impeding neuroinflammation and oxido-nitrosative stress in mice. Euro J Pharma. 2014;744:124–131.
- Zaidi SK, Hoda M, Tabrez S, et al. Protective effect of Solanum nigrum leaves extract on immobilization stress induced changes in rat’s brain. Evid Complement Alter Med E. 2014;912450.
- Viana M, Barbas C, Bonet B, et al. In vitroeffects of a flavonoid-rich extract on LDL oxidation. Atheros. 1996;123(1–2):83–91.
- Pinder RM, Sandler M. Alcohol, wine and mental health: focus on dementi and stroke. J Psychopharm. 2004;18(4):449–456.
- Dogra NK, Kumar S. A review on ethno-medicinal uses and pharmacology of Vernonia cinerea Less. Nat Prod Res. 2015;29(12):1102–1117.
- Goggi A, Malpathak N. Antioxidant activities of root, stem and leaves of vernonia cinerea (L) less. Free Rad Anti. 2017;7(2):178–183.
- MajallahTamatLatehanSinseh China. Pulau Pinang. Kumpulan Keempat: Pusat LatehanSinseh China; 1976
- Muir CK. Depressant action of an extract of Vernonia cinerea. Med J Malay. 1981;36(2):119–121.
- Pratheesh Kumar P, Kuttan G. Vernonia cinerea L. scavenges free radicals and regulates nitric oxide and proinflammatory cytokines profile in carrageenan induced paw edema model. Immuno Pharm Immun. 2009;31(1):94–102.
- Chang CC, Yang MH, Wen HM, et al. Estimation of total flavonoid content in propolis by two complementary colorimetric methods. J Food Drug. 2020;10:178–182.
- Gomes CA, Girao CT, Andrade JL, et al. Anticancer activity of phenolic acids of natural or synthetic origin: a structure-activity study. J Med Chemist. 2003;46(25):5395–5401.
- McDonald S, Prenzler PD, Antolovich M, et al. Phenolic content and antioxidant activity of Olive extracts. Food Chemist. 2001;73(1):73–84.
- Cemek M, Büyükokuroğlu ME, Sertkaya F, et al. Effects of food color additives on antioxidant functions and bio element contents of liver, kidney and brain tissues in rats. Food Nutr Res. 2014;2(10):686–691.
- Navaneethakrishnan S, Divya T, Jayaraman R, et al. Protective effect of vernonia cinerea against sunset yellow induced anxiogenic behaviour in mice model: counteract oxidative damage based approach. Nat Prod J. 2021;11:537–545.
- Organization for Economic Co-operation and Development. Guidance document on acute oral toxicity testing 423 [R]. Paris: OECD; 2001.
- Misra HP, Fridovich I. The oxidation of phenylhydrazine: superoxide and mechanism. Biochem. 1976;15(3):681–687.
- Catalase AH. In Methods of enzymatic analysis. New York: Verlag Chemie/Academic Press Inc; 1974. p. 673–684.
- Ohkawa H, Ohishi N, Yagi K. Assay for lipid peroxides in animal tissues by thiobarbituric acid reaction. Anal Biochemist. 1979;95(2):351–358.
- Srikumar BN, Ramkumar K, Raju TR, et al. Assay of acetylcholinesterase activity in the brain. Brain Behav. 2004;16:142–144.
- Kakkar V, Kaur IP. Evaluating potential of Curcumin loaded solid lipid nanoparticles in aluminium induced behavioural, biochemical and histopathological alterations in mice brain. Food Chemist Toxic. 2011;49(11):2906–2913.
- Meenakshi J, Swati R, Rajendra H. Investigations on anti-inflammatory and protective activity of Ethyl acetate fraction isolated from Vernonia Cinerea (L) in Spargue Dawley rats. J Pharm Phys. 2018;P6:92–98.
- Ketsuwan N, Leelarungrayub J, Kothan S, et al. Antioxidant compounds and activities of the stem, flower, and leaf extracts of the anti-smoking Thai medicinal plant: vernonia cinerea Less. Drug Des Dev Therap. 2017;11:383–391.
- Siddiqui Z, Rizvi S, Tabassum I. Effects of Ocimum sanctum and Camellia sinensis on stress-induced anxiety and depression in male albino Rattus norvegicus. Indian J Pharma. 2010;42(5):283–288.
- Chakraborti A, Gulati K, Banerjee BD, et al. Possible involvement of free radicals in the differential neurobehavioral responses to stress in male and female rats. Behav Brain Res. 2007;179(2):321–325.
- Masood A, Banerjee B, Vijayan V, et al. Modulation of stress-induced neurobehavioral changes by nitric oxide in rats. E J Pharm. 2003;458(1–2):135–139.
- Vogel HG. Drug discovery and evaluation: pharmacological assays.Springer Nature. London; 2002.
- Satyanathan V, Kumar AE, Babu MS, et al. Sedative-Hypnotic activity of whole plant extract of Vernonia cinerea (Linn) Less. J Res Pharma Pharm. 2012;1(2):169–171.
- Halliwell B. Antioxidants and human disease: a general introduction. Nut Rev. 1997;55(1):S44-9-S49–52.
- Hamm RJ, Pike BR, Odell DM, et al. The rota rod test: an evaluation of its effectiveness in assessing motor deficits following traumatic brain injury. J Neuro. 1994;11(2):187–196.
- Rogers DC, Campbell CA, Stretton JL, et al. Correlation between motor impairment and infarct volume after permanent and transient middle cerebral artery occlusion in the rat. Strok. 1997;28(10):2060–2066.
- Carter RJ, Lione LA, Humby T, et al. Characterization of progressive motor deficits in mice transgenic for the human Huntington’s disease mutation. J Neuros. 1999;19(8):3248–3257.
- Perez RM, Perez JA, Garcia LM, et al. Neuropharmacological activity of Solanumnigrum fruit. J Ethno. 1998;62(1):43–48.
- Onaivi ES, Maguire PA, Tsai NF, et al. Comparison of behavioral and central BDZ binding profile in three rat lines. Pharma Biochemist Behav. 1992;43(3):825–831.
- Wolffgramm J, Mikolaiczyk C, Coper H. Acute and subchronic benzodiazepine-barbiturate-interactions on behaviour and physiological responses of the mouse. Naunyn-Schmiedeberg Arch Pharma. 1994;349(3):279–286.
- Gandhi S, Abramov AY. Mechanism of oxidative stress in neurodegeneration. Ox Med Cell Long. 2012;2012:1–11.
- Kumar PP, Kuttan G. Vernonia cinereaL scavenges free radicals and regulates nitric oxide and proinflammatory cytokines profile in carrageenan induced paw edema model. ImmunopharmacolImmunotoxicol. 2009;31(1):94–102.
- Ngo DH, Vo TS. An updated review on pharmaceutical properties of gamma-amino butyric acid. Molecules. 2019;24(15):2678.
- Kraal AZ, Arvanitis AR, Jaeger AP, et al. Could dietary glutamate play a role in psychiatric distress. Neuro. 2020;79:13–19.
- Lydiard RB. The role of GABA in anxiety disorders. J Cl Psychiat. 2003;64:21–27.
- Al-Snafi AE, Talab TA, Majid WJ. Medicinal plants with central nervous activity. Overview IOSR J Pharm. 2019;9(3):52–102.
- Bhattacharya SK, Satyan KS. Experimental methods for evaluation of psychotropic agents in rodents: anti-anxiety agents. Indian J Ex Bio. 1997;35(6):565–575.
- Ahmad W, Zaharin S, Syafiqah N. Antioxidant activity of vernonia cinerea, peperomiapellucidaand the combination of vernonia cinerea and peperomiapellucida. J Acad. 2020;8(1):24–28.
- Alara OR, Abdurahman NH, Ukaegbu CI. Soxhlet extraction of phenolic compounds from Vernonia cinerea leaves and its antioxidant activity. J Applied Res Med Aroma. 2018;11:12–17.
- Alara OR, Abdurahman NH, Ukaegbu CI, et al. Vernonia cinerea leaves as the source of phenolic compounds, antioxidants, and anti-diabetic activity using microwave-assisted extraction technique. Crops Prod. 2018;122:533–544.