ABSTRACT
Hydroxychloroquine (HCQ) is a successful therapeutic anti-inflammatory drug frequently utilized in clinical settings for a number of disorders and diseases, however, it impact on obesity has not yet been fully established. Therefore, this study aimed to investigate the effectiveness and mechanism of action of HCQ on obesity in rats fed high fat diet (HFD). Adult male rats were feed a HFD for 18 weeks and concurrently treated with HCQ. The data showed that HCQ significantly reduced body weight gain, decreased blood glucose, HbA1c, and improved insulin level and insulin sensitivity. Furthermore, it normalized the serum lipid profile and D-dimer level. Comparable values of cardiac function parameters with the control group were observed The effects of HCQ was explained by striking amelioration of oxidative stress indicated by the lower levels of 4HNE and higher levels of GPx and GSH in the liver of HFD+HCQ than HFD fed rats. Furthermore, the inflammatory and the anti-inflammatory markers in serum of HFD+HCQ-treated rats were normalized to the control levels. In conclusion, the stabilization of redox balance and modulation of inflammatory response and their interdependence explain the ameliorative effect of HCQ on obesity and related metabolic disruption as well as organ functions.
Introduction
Obesity and habitual high caloric intake have emerged as worldwide health issues with predictions of exponential growth in the future; thus, they have attracted considerable public attention because of their associated pathological outcomes. Epidemiological evidence has confirmed that high-fat diet (HFD) leads to overweight and obesity caused by fat accumulation, and a positive correlation is observed between the amount of HFD intake and the level of obesity [Citation1].
Evidence from animal experiments and patients that consumed a HFD induces oxidative stress, suggesting the downregulation of the antioxidant defense system in individuals with obesity [Citation2]. Redox imbalance has been implicated in the pathogenesis of metabolic disorders, including the disruption of lipid and carbohydrate metabolism, which is associated with increased insulin resistance, obesity, and organ dysfunction [Citation3]. Inflammatory adipokines induce reactive oxygen species (ROS) generation, producing a shift in redox state known as oxidative stress. Considering that adipose tissue secretes adipokines, which produce ROS, adipose tissue is considered as a site for the generation of systemic oxidative stress [Citation4].
Obesity is associated with inflammation, and it is characterized by disturbance in the level of pro-inflammatory cytokines, including tumor necrosis factor-alpha (TNF-α) and interleukin-6 (IL-6) [Citation5]. Moreover, adipokines such as leptin, adiponectin, resistin, and visfatin, which are secreted from adipose tissue, show disrupted levels in obesity [Citation6]. Leptin synthesized and secreted by white adipose tissue regulates the appetite through leptin receptors primarily in the hypothalamic nuclei, and it is expressed in several organs and immune cells [Citation7]. Adiponectin is a cytokine that is released from adipose tissues; thus, it can be grouped in adipokines. It plays a number of molecular and cellular roles in physiological processes, primarily lipid metabolism, immune response, inflammation, and insulin sensitivity [Citation8]. Deregulation of these cytokines is implicated in obesity and diabetes-related inflammatory response [Citation9]. Leptin and adiponectin secretions are regulated in relation to the degree of adiposity. Plasma leptin concentration is elevated in individuals with obesity in proportion to the body mass index, whereas adiponectin secretion decreases in relation to the amount of adipose tissue [Citation10].
Hydroxychloroquine (HCQ) is a derivative of quinolone, which belongs to 4-aminoquinolines (heterocyclic aromatic compound). It has long been used as an effective antimalarial drug. HCQ is an economical and affordable monotherapy that can be combined with other drugs for the treatment of autoimmune diseases such as systemic lupus erythematosus [Citation11]. HCQ is used as prophylaxis in the primary thrombosis of patients who are positive for antiphospholipid antibody without systemic autoimmune disease [Citation12]. Despite the risk of the side effects of HCQ in some patients with COVID-19, which coexist with other complications, low dose of HCQ can decrease the mortality rate among critically ill patients with COVID-19 [Citation13]. HCQ may have antidiabetic potential, but information supporting this assumption is lacking [Citation14]. Although HCQ has long been used to treat autoimmune illnesses, its mode of action remains unclear [Citation15]. Therefore, this study aimed to examine the efficacy and mode of action of HCQ on obesity in rats fed a HFD.
Materials and methods
Animals and treatment
Male Wistar albino rats weighing 120–150 g were obtained from biological products and vaccination animal houses (VACSERA, Egypt). All the rats were kept at a constant temperature (25°C) and photoperiod (12 h of light/12 h of dark). They were given a commercial rat pellet meal and free access to water during the accommodation period for a week.
After acclimatization to our facilities, the rats were randomly assigned to one of the following groups (): control group in which only normal chow diet and tap water were provided only; HCQ-treated group in which rat daily received oral treatment with 40 mg/kg of HCQ [Citation16] for 18 months; HFD group in which rats were fed diet consisting 68% standard feed, 30% animal fat, and 2% cholesterol for 18 weeks; HFD + HCQ-treated group in which rats received a HFD and treated with HCQ for 18 weeks (sodium HCQ in water, 40 mg/kg/day) [Citation16].
Figure 1. Diagram of the experimental design. After a week of acclimatization, male albino rats weighing 120–150 g were fed a high-fat diet (HFD) with or without HCQ (40 mg/kg of body weight [BW]/day orally) for 16 weeks. All rats were analyzed after 18 weeks. Then, the blood and liver tissue were collected for biochemical evaluation.
![Figure 1. Diagram of the experimental design. After a week of acclimatization, male albino rats weighing 120–150 g were fed a high-fat diet (HFD) with or without HCQ (40 mg/kg of body weight [BW]/day orally) for 16 weeks. All rats were analyzed after 18 weeks. Then, the blood and liver tissue were collected for biochemical evaluation.](/cms/asset/78ce506c-9e29-43d9-a820-e38ad5edbb41/teba_a_2211832_f0001_oc.jpg)
During the dietary period of 18 weeks, the animals were weighed, and blood glucose levels were assessed.
After 18 weeks, rats were fasted overnight and anesthetized with ketamine/xylazine (10 mL/kg BW, IP). Blood was drawn by heart puncture into non-heparinized tubes and centrifuged at 3000 rpm for 15 min. After carefully separating the sera, samples were stored at−20°C for biochemical analysis.
Animals were dissected, and the liver was obtained. Liver samples were homogenized in Tris-HCl buffer using a tissue homogenizer (0.1 M, pH 7.4) followed by centrifugation, and the supernatant was kept at−20°C for further biochemical examination.
Biochemical determination
Glucose levels in serum and HbA1c levels in the blood were estimated using commercial kits provided by BioMed Diagnostics (White City, OR, USA) and Biosystems (Costa Brava, Barcelona, Spain), respectively. Serum insulin levels were estimated using an enzyme-linked immunosorbent assay (ELISA) kit purchased from RayBiotech (Norcross, GA, USA). Homeostatic model assessment (HOMA-IR) was performed as described previously [Citation17,Citation18].
Serum lipid fraction levels, including total lipids, total cholesterol (TC), triglycerides (TG), and high-density lipoprotein (HDL), were assessed using colorimetric test kits purchased from Spinreact (Girona, Spain). Friedewald’s formula was used to calculate the level of low-density lipoprotein cholesterol (LDL-C) and very low-density lipoprotein cholesterol (VLDL-C) [Citation19]. Creatine kinase myocardial band (CK-MB) and lactic dehydrogenase (LDH) activities in the serum were estimated in accordance with the standard methods using kits purchased from BioSystems (Barcelona, Spain). Serum troponin T level was determined using the sandwich enzyme immunoassay technique kit purchased from Kamiya Biomedical (Seattle, USA). Lipid peroxidation was determined by estimating the amount of 4-hydroxynonenal (4-HNE) according to the manufacturer’s instructions (FineTest, Wuhan, China). Glutathione (GSH) concentration and glutathione peroxidase (GPx) activities were estimated using kits supplied by Biodiagnostic (Giza, Egypt). Serum IL-6, IL-10, and TNF-α levels were assessed using ELISA kits purchased from BosterBio (California, USA) and ElisaGenie (London, UK). Leptin and adiponectin were measured in serum using an ELISA mouse leptin immunoassay kit (Catalog No: MOB00) and ELISA mouse Adiponectin/Acrp30 immunoassay kit (Catalog No: MRP300) obtained from R & D systems, MN, USA. Plasma D-dimer was determined using the Innovance D-dimer assay (Siemens, Marburg, Germany).
Statistical evaluation
GraphPad Prism 8.0 was used to perform statistical analysis of all the data. The findings are shown as the mean ± SEM (n = 5). One-way analysis of variance was used to analyze statistical comparisons, followed by Duncan’s multiple range tests. Percentages of changes in each group were calculated to compare the treated groups with control and HCQ groups. The level of statistical significance was set at P < 0.05.
Results
HCQ reduces body weight gain in HFD-fed rats
Consuming HFD for 18 months caused a significant increase in body weight (P < 0.001; ) compared with the control group. On the contrary, the administration of HCQ concurrently with HFD remarkably (P < 0.001) controlled the increase in body weight. Meanwhile, control and HCQ-treated rats gained comparable weight, which was significantly lower than the experimental group.
Figure 2. Effect of HCQ on body weight changes (a), and weekly glucose levels (b) of the studied groups. Cont.: control, HCQ: hydroxychloroquine, HFD: high-fat diet. ** and *** indicate statistical significance at P < 0.01 and P < 0.001, respectively, compared with the control group. ## and ### indicate statistical significance at P < 0.01 and P < 0.001, respectively, compared with the HCQ group. $$$ indicates statistical significance at P < 0.001 compared with the HFD group.
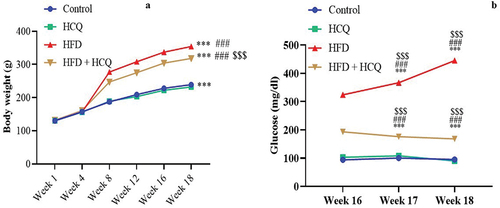
HCQ improves hyperglycemic and diabetic indexes
Blood glucose levels were measured at weeks 16–18 of the experiment and revealed a substantial (P < 0.001) increase in rats receiving HFD compared with control rats (). By contrast, the concurrent administration of HCQ to HFD-treated rats remarkably (P < 0.001) hampered the development of hyperglycemia until the completion of the experiment. Blood glucose levels in the control group and HCQ-treated animals were in the normal range.
The assessment of diabetic parameters and indices at the end of the study revealed that HFD-fed rats had significantly (P < 0.001) high HbA1c and insulin levels. In addition, homeostatic model assessment of insulin resistance (HOMA-IR) significantly (P < 0.001) increases, whereas homeostatic model assessment of β-cell function (HOMA-β) and homeostatic model assessment of insulin sensitivity (HOMA-IS) decrease. On the contrary, the HCQ therapy markedly prevented these alterations compared with HFD-treated rats. The control group and the animals treated with HCQ had diabetes parameter values that were comparable to one another within the normal range ().
Table 1. Effect of HCQ on glucose levels, glycosylated hemoglobin, and insulin sensitivity in HFD-fed rats.
HCQ ameliorates changes in the lipid profile and D-dimer levels in HFD-fed rats
Rats fed with a HFD had significantly (P < 0.001) higher levels of total lipids, TC, TGs, LDL, and VLDL and lower levels of HDL in their serum than the control group (). Compared with rats fed a HFD, the levels of lipid parameters were significantly (P < 0.001) improved when HFD-fed animals were given HCQ. The rats in the control group and those that had been given HCQ showed values that were comparable to those of the usual values.
Figure 3. Effect of HCQ on the levels of cholesterol (a), triglyceride (b), low-density lipoprotein (LDL-C) (c), very low-density lipoprotein (VLDL-C) (d), high-density lipoprotein (HDL-C) (e), and D-dimer (f) in serum of rats in different groups. Cont.: control, HCQ: hydroxychloroquine, HFD: high-fat diet. Values are expressed as means ± SEM (n = 5). (*, **, and *** indicate statistical significance at P < 0.05, P < 0.01, and P < 0.001, respectively, compared with the control group. # and ### indicate statistical significance at P < 0.05 and P < 0.001, respectively, compared with the HCQ group. $$$ indicates statistical significance at P < 0.001 compared with the HFD group).
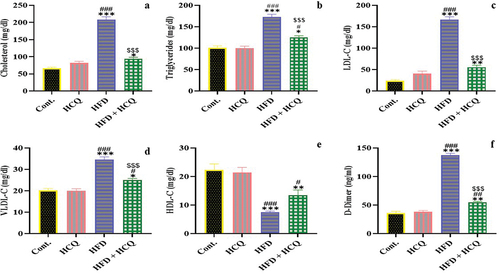
D-dimer concentration in serum was assessed to evaluate liver function under the current experimental setup (). Compared with rats fed a standard diet, animals maintained on HFD for 18 months displayed significantly (P < 0.001) higher serum levels of D-dimer. This increase was significantly (P < 0.001) improved by the administration of HCQ compared with HFD-fed rats. D-dimer levels in serum remained unchanged in control and HCQ-treated animals.
HCQ ameliorates heart function in HFD-fed rats
Metabolic disorder can affect heart function; thus, the troponin content as well as creatine kinase myocardial band (CK-MB) and lactate dehydrogenase (LDH) activity in the serum of the studied groups were assessed (). The data revealed that HFD-fed rats displayed a significant (P < 0.001) increase in cardiac function parameters, including the level of troponin-T and the activity of CK-MB and LDH compared with control rats, which were remarkably (P < 0.001) prevented by HCQ treatment. Meanwhile, an insignificant difference in heart function indicators was observed between the rats in the control group and those treated with HCQ ().
Figure 4. Effect of HCQ on the serum level of troponin-T (a), as well as the activities of creatine kinase myocardial band (CK-MB) (b) and lactate dehydrogenase (LDH) (c) in different groups. Cont.: control, HCQ: hydroxychloroquine, HFD: high-fat diet. Values are expressed as means ± SEM (n = 5). (*, **, and *** indicate statistical significance at P < 0.05, P < 0.01, and P < 0.001, respectively, compared with the control group. # and ### indicate statistical significance at P < 0.05 and P < 0.001, respectively, compared with the HCQ group. $$$ indicates statistical significance at P < 0.001 compared with the HFD group).
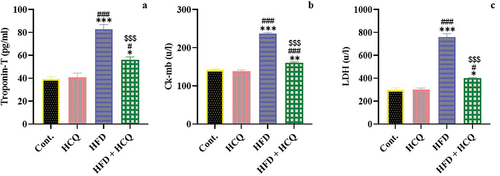
HCQ ameliorates oxidative stress in HFD-fed rats
The liver is the central organ for several metabolic and oxidative activities; therefore, the level of oxidative stress in the liver was assessed under the experimental condition (). Daily feeding a HFD for 18 weeks significantly (P < 0.001) increased the 4-HNE level and severely (P < 0.001) decreased the GSH content and GPx activity in the liver. Treatment with HCQ concurrently with HFD supplementation caused a significant (P < 0.001) improvement in oxidative stress indicators. On its own, HCQ did not affect the redox balance in the liver when administered daily for 18 weeks, but it showed values that were comparable to those of the control group ().
Figure 5. Effect of HCQ on the oxidative stress marker 4-hydroxynonenal (4-HNE) (a), glutathione (GSH) content (b), and glutathione peroxidase (GPx) activity (c) in the liver of rats in different groups. Cont.: control, HCQ: hydroxychloroquine, HFD: high-fat diet. Values are expressed as means ± SEM (n = 5). (** and *** indicate statistical significance at P < 0.01 and P < 0.001, respectively, compared with the control group. ## and ### indicate statistical significance at P < 0.01 and P < 0.001, respectively, compared with the HCQ group. $$$ indicates statistical significance at P < 0.001 compared with the HFD group).
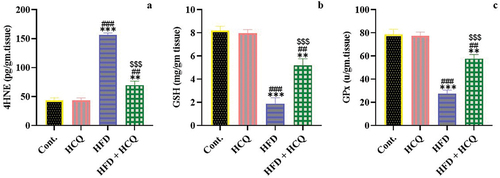
HCQ improves the inflammatory response in HFD-fed rats
The effect of HCQ on the link among inflammation, obesity, and HFD was studied by estimating pro-inflammatory TNF-α and IL-6 and anti-inflammatory IL-10 cytokines as well as adipokines (leptin and adiponectin; ). The present data show that HFD-fed rats had a significant (P < 0.001) increase in TNF-α, IL-6, and leptin levels, whereas IL-10 and adiponectin levels were significantly (P < 0.001) decreased. This effect was remarkably (P < 0.001) alleviated when HCQ was administered to rats that were maintained on HFD. Meanwhile, the control group and HCQ-treated rats showed comparable values.
Figure 6. Effect of HCQ on the serum level of tumor necrosis factor alpha (TNF-α) (a), interleukin-6 (IL-6) (b), and leptin (c); interleukin-10 (IL-10) (d) and adiponectin (e) of rats in different groups. Cont.: control, HCQ: hydroxychloroquine, HFD: high-fat diet. Values are expressed as means ± SEM (n = 5). (*, **, and *** indicate statistical significance at P < 0.05, P < 0.01, and P < 0.001, respectively, compared with the control group. #, ##, and ### indicate statistical significance at P < 0.05, P < 0.01, and P < 0.001, respectively, compared with the HCQ group. $$$ indicates statistical significance at P < 0.001 compared with the HFD group).
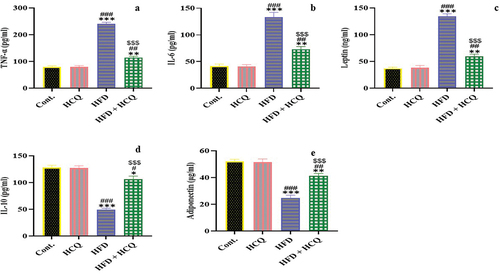
Discussion
Overweight and obesity are major health issues affecting several populations in modernized and developing countries because individuals with obesity frequently have organ dysfunction caused by the development of diabetes, oxidative stress, and inflammation. Weight loss is the first-line treatment of obesity before bariatric surgery. HCQ is a potent therapy for autoimmune diseases with several pharmacological effects [Citation11]. Therefore, the ameliorative effect of HCQ on obesity and its complications were studied. In the current study, HCQ improved body weight gain, hyperglycemia, and lipid profile as well as maintained the heart and liver function in HFD-fed rats. An effect is attributed to preserving the homeostatic level of redox balance and the anti-inflammatory properties of HCQ.
Rats fed a HFD for 18 weeks displayed a marked increase in body weight, severe hyperglycemia, and increased HbA1c, suggesting the development of diabetes. This effect is associated with decreased insulin levels in serum, indicating the development of insulin resistance and β-cell dysfunction, as indicated by the high HOMA-IR and low HOMA-β values in obese rats. Concurrent treatment of HFD-treated rats with HCQ remarkably ameliorate these effects, thereby supporting earlier studies that HCQ improved insulin resistance in obese rats by controlling lipid metabolism [Citation16]. Furthermore, the current data of homeostatic model assessment for insulin resistance suggest appropriate function of β-cell. Therefore, HCQ can induce protection against obesity-mediated T2 diabetes and maintain hepatic and pancreatic functions. These findings are consistent with recent preliminary data for the antidiabetic potential of HCQ; however, more clinical trials and mechanistic research are required to ascertain its benefits [Citation14].
The liver plays an essential role in maintaining systemic glucose and lipid levels during feeding and fasting, which were coordinated by highly tuned Kreb’s cycle via insulin and glucagon balance [Citation20]. Hepatic lipid and carbohydrate biosynthesis increases in T2 diabetes, which contributes to hyperglycemia and hypertriacylglycerolemia [Citation21]. The consumption of HFD caused a significant increase in serum lipid fractions, indicating disrupted lipid metabolism, which is a main indicator of insulin resistance in the liver and other organs with the development of fatty liver. The anti-lipidemic effect of HCQ is supported by a significant normalization of the blood lipid profile under the present condition, indicating normal hepatic functions. The HCQ+HFD group showed decreased TC, TG, LDL-C and VLDL-C levels as well as increased HDL level, suggesting protection against lipid toxicity. These findings indicate that HCQ may regulate lipid metabolic disruption, which might be beneficial for hepatic and cardiovascular functions.
The protection of liver function during the development of obesity was achieved by HCQ treatment as indicated by the amelioration of D-dimer levels in the serum of HFD-fed rats. D-dimer is a prognostic marker that is indicative of liver dysfunction [Citation22] and pancreatitis, as the D-dimer level was related to dyslipidemia and TG/HDL-C ratio [Citation23]. Patients with obesity have increased plasma concentrations of all pro-thrombotic agents [Citation24]. Thus, treatment with HCQ might have a hepatoprotective effect and might improve the coagulative process in patients with obesity.
Obesity and cardiac dysfunction frequently coexist [Citation25]. In the present study, rats fed a HFD had significantly higher serum levels of troponin-T as well as CK-MB and LDH activities than control rats, indicating obesity-related cardiac dysfunction and confirming the adverse effect of obesity on heart function. These findings support recent research results, that is, HFD caused obesity and heart anomalies such as cardiac remodeling, contractile dysfunction, ultrastructural disarray, apoptosis, and inflammation [Citation26]. Even short-term HFD can result in physiological alterations that could affect cardiac health [Citation27]. In the present study, heart function was significantly improved in the HFD+HCQ group, which is manifested by a significant decrease in markers of heart function when compared with obese rats. Recently, HCQ mediated an improvement in heart performance, probably via enhancing myosin motor function in dilated cardiomyopathy of transgenic D94A mice [Citation28]. Thus, HCQ markedly preserved heart function in obese rats.
Obesity, diabetes, and other metabolic syndrome are redox diseases [Citation3]. Oxidative stress is a major pathophysiological process that characterizes several liver disorders, including metabolism and proliferation. It is also a major cause of liver injury caused by obesity. The liver is the main organ for lipid metabolism and fatty acid oxidation in the body. Thus, the effect of HCQ on HFD-induced oxidative stress in the liver was assessed. Feeding a HFD for 18 months caused a significant increase in 4-HNE, which is a marker of lipid peroxidation, and induced a severe decrease in GPx activity and GSH content in the liver of obese rats. Recently, HFD influences oxidative stress in the liver via peroxisomal and mitochondrial β-oxidation of free fatty acids [Citation29] and overconsumption of oxygen [Citation4]. Furthermore, people with obesity exhibit increased expression of pro-oxidant genes of the oxidative mechanism [Citation30], expressing their pathophysiological role in obesity. The current findings demonstrated that HCQ prevented the change in redox balance because antioxidant and lipid peroxidation levels were preserved in rats treated with the combination of HFD and HCQ compared with the control group. The protective effect of HCQ might be facilitated by controlling fatty acid β-oxidation activity, thereby regulating the production of ROS in cells, including hepatocytes and cardiomyocytes. Moreover, HCQ maintained a normal mitochondrial antioxidant system in activated T cells [Citation15]. Oxidative stress has a direct effect on metabolism, including the activity of the tricarboxylic acid cycle and electron transport chain enzymes [Citation31]. Considering that HCQ can alter proton fluxes in many subcellular organelles, these drugs can change the type of ROS present and the ability of ROS to damage cells and subcellular organelles [Citation32]. Nevertheless, the mechanism by which HCQ changes ROS reactivity and the state of redox biomarkers warrants further investigation in overweight and obese individuals.
Numerous studies have revealed a link among obesity, chronic inflammation, organ pathology, and immunological activation [Citation33,Citation34]. Organ dysfunction results from inflammation in people with obesity or T2 diabetes [Citation35]. Obesity-related inflammation is an important risk for many diseases, including T2 diabetes [Citation36]. Rats fed a HFD for 18 months exhibited severe inflammation with a marked shift of redox balance toward oxidative stress. In addition, an increase in pro-inflammatory mediators (TNF-a, IL-6, and leptin) and marked decrease in anti-inflammatory cytokines (IL-10 and adiponectin) were observed. These findings are consistent with previous results, that is, HFD increased IL-6, which activates hepatic synthesis and C-reactive protein secretion and enhances fibrinogen production [Citation37]. In the current study, HCQ prevented the elevation of inflammatory cytokines. Meanwhile, an increase in anti-inflammatory cytokine level indicated an anti-inflammatory effect in HFD-fed rats. These findings are consistent with previous study results, that is, HCQ reduced the hepatic level of macrophage-specific genes and pro-inflammatory genes of IL-1β, TNF-α, and monocyte chemoattractant protein-1 in obese mice [Citation16,Citation38]. These findings indicate that the improvement of oxidative stress, production of inflammatory adipokines, and decrease in fat accumulation result from HCQ intervention.
The improvement in adiponectin levels in HCQ-treated obese rats has a fundamental and beneficial effect. The ability of adiponectin to control obesity consequences is attributed to several mechanisms [Citation8]. It can suppress the induction of TNF-α and enhance the level of anti-inflammatory IL-10. Moreover, adiponectin decreases the amount of adipose tissue TG and improves insulin signaling and sensitivity. It also activates β-oxidation through enhancing peroxisome proliferator-activated receptor alpha (PPAR-α) receptor phosphorylation and stimulating AMP-activated protein kinase (AMPK) cascade [Citation8]. Thus, these findings indicate that the effect of HCQ on obesity is mediated by adiponectin. In patients with systemic lupus erythematosus, treatment with HCQ controls adipokines [Citation39]. In general, obesity is characterized by hyperleptinemia and resistance to weight loss [Citation40]. Thus, we suggest that HCQ might normalize leptin levels, suppress leptin resistance, and/or restore the balance between leptin and adiponectin in rats treated with HFD+HCQ. These data indicate that HCQ intervention in obese rats improved oxidative stress status, declined production of inflammatory adipokines and reduced fat storage.
Conclusions
The ameliorative effect of HCQ on obesity and associated metabolic disturbance as well as organ functions is explained by the stabilization of redox balance, modulation of inflammatory response, and their interaction.
Ethics approval and consent to participate
The current investigation was performed in accordance with the guidelines of the national research council for care and use of laboratory animals and under the supervision of the animal ethics committee of Mansoura University, Egypt (Sci-Z-M-2021-39).
Availability of data and materials
The data that support the findings of this study are available on request from the corresponding author.
Acknowledgments
Facilities provided by Mansoura University are greatly acknowledged.
Disclosure statement
The authors declare that they have no conflict of interest.
References
- Wang L, Wang H, Zhang B, et al. Elevated fat intake increases body weight and the risk of overweight and obesity among Chinese Adults: 1991–2015 Trends. Nutrients. 2020;12(11):12(11. DOI:10.3390/nu12113272
- Lasker S, Rahman MM, Parvez F, et al. High-fat diet-induced metabolic syndrome and oxidative stress in obese rats are ameliorated by yogurt supplementation. Sci Rep. 2019;9(1):1–15. DOI:10.1038/s41598-019-56538-0
- Korac B, Kalezic A, Pekovic-Vaughan V, et al. Redox changes in obesity, metabolic syndrome, and diabetes. Redox Biol. 2021;42:101887.
- Fernández-Sánchez A, Madrigal-Santillán E, Bautista M, et al. Inflammation, oxidative stress, and obesity. Int J Mol Sci. 2011;12(5):3117–3132. DOI:10.3390/ijms12053117
- Islam MA, Khandker SS, Kotyla PJ, et al. Immunomodulatory effects of diet and nutrients in Systemic Lupus Erythematosus (SLE): a Systematic Review. Front Immunol. 2020;11:1477.
- Versini M, Jeandel P-Y, Rosenthal E, et al. Obesity in autoimmune diseases: not a passive bystander. Autoimmun Rev. 2014;13(9):981–1000. DOI:10.1016/j.autrev.2014.07.001
- Fujita Y. Leptin and autoimmune disease. Nihon Rinsho Meneki Gakkai Kaishi. 2017;40(3):155–159.
- Khoramipour K, Chamari K, Hekmatikar AA, et al. Adiponectin: structure, physiological functions, role in diseases, and effects of nutrition. Nutrients. 2021;13(4):1180. DOI:10.3390/nu13041180
- Kwon H, Pessin JE. Adipokines mediate inflammation and insulin resistance. Front Endocrinol. 2013;4:71.
- Carbone F, La Rocca C, Matarese G. Immunological functions of leptin and adiponectin. Biochimie. 2012;94(10):2082–2088.
- Martinez GP, Zabaleta ME, Di Giulio C, et al. The role of chloroquine and hydroxychloroquine in immune regulation and diseases. Curr Pharm Des. 2020;26(35):4467–4485. DOI:10.2174/1381612826666200707132920
- Erkan D, Unlu O, Sciascia S, et al. Hydroxychloroquine in the primary thrombosis prophylaxis of antiphospholipid antibody positive patients without systemic autoimmune disease. Lupus. 2018;27(3):399–406. DOI:10.1177/0961203317724219
- Yu B, Li C, Chen P, et al. Low dose of hydroxychloroquine reduces fatality of critically ill patients with COVID-19. Sci China Life Sci. 2020;63(10):1515–1521. DOI:10.1007/s11427-020-1732-2
- Wondafrash DZ, Desalegn TZ, Yimer EM, et al. Potential effect of hydroxychloroquine in diabetes mellitus: a systematic review on preclinical and clinical trial studies. J Diabetes Res. 2020;2020:5214751.
- Kim ML, Hardy MY, Edgington-Mitchell LE, et al. Hydroxychloroquine inhibits the mitochondrial antioxidant system in activated T cells. iScience. 2021;24(12):103509. DOI:10.1016/j.isci.2021.103509
- Qiao X, Zhou Z-C, Niu R, et al. Hydroxychloroquine improves obesity-associated insulin resistance and hepatic steatosis by regulating lipid metabolism. Front Pharmacol. 2019;10:855.
- Matthews DR, Hosker JP, Rudenski AS, et al. Homeostasis model assessment: insulin resistance and β-cell function from fasting plasma glucose and insulin concentrations in man. Diabetologia. 1985;28(7):412–419. DOI:10.1007/BF00280883
- Ko J, Skudder-Hill L, Cho J, et al. The relationship between abdominal fat phenotypes and insulin resistance in non-obese individuals after acute pancreatitis. Nutrients. 2020;12(9):12(9. DOI:10.3390/nu12092883
- Friedewald WT, Levy RI, Fredrickson DS. Estimation of the concentration of low-density lipoprotein cholesterol in plasma, without use of the preparative ultracentrifuge. Clin Chem. 1972;18(6):499–502.
- Trefts E, Gannon M, Wasserman DH. The liver. Curr Biol. 2017;27(21):R1147–r1151.
- Jones JG. Hepatic glucose and lipid metabolism. Diabetologia. 2016;59(6):1098–1103.
- Li Y, Qi X, Li H, et al. D-dimer level for predicting the in-hospital mortality in liver cirrhosis: a retrospective study. Exp Ther Med. 2017;13(1):285–289. DOI:10.3892/etm.2016.3930
- Jia X, Zhang X, Sun D, et al. Triglyceride to HDL-C ratio is associated with plasma D-dimer levels in different types of pancreatitis. Sci Rep. 2022;12(1):12952. DOI:10.1038/s41598-022-17421-7
- De Pergola G, Pannacciulli N. Coagulation and fibrinolysis abnormalities in obesity. J Endocrinol Invest. 2002;25(10):899–904.
- Mouton AJ, Li X, Hall ME, et al. Obesity, hypertension, and cardiac dysfunction: novel roles of immunometabolism in macrophage activation and inflammation. Circ Res. 2020;126(6):789–806. DOI:10.1161/CIRCRESAHA.119.312321
- Gong Y, Li G, Tao J, et al. Double knockout of Akt2 and AMPK accentuates high fat diet-induced cardiac anomalies through a Cgas-STING-mediated mechanism. Biochim Biophys Acta Mol Basis Dis. 2020;1866(10):165855. DOI:10.1016/j.bbadis.2020.165855
- Hynynen H, Mutikainen M, Naumenko N, et al. Short high-fat diet interferes with the physiological maturation of the late adolescent mouse heart. Physiol Rep. 2020;8(13):e14474. DOI:10.14814/phy2.14474
- Kanashiro-Takeuchi RM, Kazmierczak K, Liang J, et al. Hydroxychloroquine mitigates dilated cardiomyopathy phenotype in transgenic D94A Mice. Int J Mol Sci. 2022;23(24):23(24. DOI:10.3390/ijms232415589
- Tan BL, Norhaizan ME. Effect of high-fat diets on oxidative stress, cellular inflammatory response and cognitive function. Nutrients. 2019;11(11):11(11.
- Baig S, Parvaresh Rizi E, Chia C, et al. Genes Involved in oxidative stress pathways are differentially expressed in circulating mononuclear cells derived from obese insulin-resistant and lean insulin-sensitive individuals following a single mixed-meal challenge. Front Endocrinol. 2019;10:256.
- Masschelin PM, Cox AR, Chernis N, et al. The impact of oxidative stress on adipose tissue energy balance. Front Physiol. 2019;10:1638.
- Klouda CB, Stone WL. Oxidative stress, proton fluxes, and chloroquine/hydroxychloroquine treatment for COVID-19. Antioxidants (Basel). 2020;9(9):9(9.
- Yu Y, Liu Y, An W, et al. STING-mediated inflammation in Kupffer cells contributes to progression of nonalcoholic steatohepatitis. J Clin Invest. 2019;129(2):546–555. DOI:10.1172/JCI121842
- Liu K, Zhao E, Ilyas G, et al. Impaired macrophage autophagy increases the immune response in obese mice by promoting proinflammatory macrophage polarization. Autophagy. 2015;11(2):271–284. DOI:10.1080/15548627.2015.1009787
- Raggi F, Rossi C, Faita F, et al. P2X7 receptor and heart function in a mouse model of systemic inflammation due to high fat diet. 2022;15:2425–2439.
- Wu H, Ballantyne CM. Metabolic inflammation and insulin resistance in obesity. Circ Res. 2020;126(11):1549–1564.
- Khateeb S, Albalawi A, Alkhedaide A. Diosgenin modulates oxidative stress and inflammation in high-fat diet-induced obesity in mice. Diabetes Metab Syndr Obes. 2022;15:1589–1596.
- Wong SK. Repurposing new use for old drug chloroquine against metabolic syndrome: a review on animal and human evidence. Int J Med Sci. 2021;18(12):2673–2688.
- Wakiya R, Ueeda K, Shimada H, et al. Supplemental hydroxychloroquine therapy regulates adipokines in patients with systemic lupus erythematosus with stable disease. Clin Rheumatol. 2022;41(11):3345–3353. DOI:10.1007/s10067-022-06282-0
- Obradovic M, Sudar-Milovanovic E, Soskic S, et al. Leptin and obesity: role and clinical implication. Front Endocrinol. 2021;12:585887.