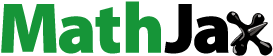
ABSTRACT
This study sought to assess the health impact of feeding adult healthy rats with either 7% (w/w) animal-derived fat (subcutaneous rump fat or peri-kidney fat) from domestic buffalo or vegetable oil (corn oil or olive oil) for 8 weeks on bioactivity; lipid profile; hepatic and cardiac histological changes. Twenty-four male albino rats were randomly categorized into four dietary groups (six rats/group): 1) diet contains 7% (w/w) corn oil; 2) diet contains 7% (w/w) olive oil; 3) diet contains 7% (w/w) subcutaneous rump fats and 4) diet contains 7% (w/w) Peri-kidney fats. Animal fats intake significantly increased serum total lipids (TL), triacylglycerol (TG), low-density lipoprotein cholesterol (LDLC), cardiac troponin-I (CTn-I) concentration as well as alanine aminotransferase (ALT), aspartate aminotransferase (AST), gamma-glutamyl transpeptidase (GGT), creatine kinase MB (CK-MB) and lactate dehydrogenase (LDH) activities compared to vegetable oils intake. In histopathological research, liver and heart sections excised from rats consumed animal-derived fats showed several signs of extensive damage, including inflammation as well as fibrosis. Compared to animal-derived fats, vegetable oils consumption markedly increased serum high-density lipoprotein cholesterol (HDLC) concentration and exhibited a potential attenuation effect on liver and heart enzymes activities and a potential role in the reduction of TG in the bloodstream of rats as well as revealed normal liver and heart histopathological architectures. In conclusion, this study suggests that, replacing animal fats, especially peri-kidney fat with vegetable oils, particularly olive oil in diets, liver and heart can be protected.
Introduction
The majorities of individuals are worried about their general health, and frequently want to cut dietary calories and choose nutritious and healthier meals [Citation1,Citation2]. Animal and vegetable dietary lipids have gained more interest as a vital source of important elements, due to the increased demands of the consumers toward healthier diets [Citation3]. The ingestion of fats with specific fatty acids (FA) can have either a negative or a protective health impact [Citation4]. Excessive consumption of harmful fats may contribute to the development of obesity, diabetes, heart problems, chronic renal disease, liver disease, metabolic syndrome and several cancer kinds [Citation5,Citation6]. Therefore, there is increasing attention in FA types and composition as well as the impacts of lipids on the overall health of humans [Citation7,Citation8].
The nutritional quality of meat, especially in relation to their FA composition, has gained a greater concern [Citation9,Citation10]. So, demand to produce lean animal meat with improved FA composition containing less saturated fatty acids (SFA) and more unsaturated fatty acids (USFA) has grown [Citation11]. Despite the fact that the meat nutritional properties are largely related to its fat content and FA composition, an association between consumption of fat from animal sources and coronary heart disease (CHD), diabetes and obesity was established due to high concentration of cholesterol, SFA and low concentration of polyunsaturated fatty acids (PUFA) [Citation12,Citation13]. In Egypt, Bubalus bubalis, the Egyptian buffalo, is regarded as the main source of milk and red meat and is important to the agricultural economy and food security [Citation14].
Foods from plant are good sources of vegetable proteins, antioxidants and important FAs as well as phytosterols that affect plasma cholesterol concentrations [Citation15]. Corn oil is a light yellow vegetable oil rich in PUFAs and phytosterols. Corn oil is considered as one of the most important edible oils in most industries of foods and mostly used for cooking due to its good composition of FAs and the low price [Citation16]. It contains a mixture of both SFAs and USFAs and is distinguishable from other lipid sources due to its relatively high amounts of USFAs, especially linoleic acid (LA) (53–55%), oleic acid (OA) (28–30%) and low concentrations of SFAs (15%) [Citation17].
Olive oil is one of the healthiest sources of fat in human nutrition because of the high proportions of monounsaturated fatty acids (MUFA) principally OA (55–83%) [Citation18]. It contains a number of essential ingredients, including squalene, polyphenols, and tocopherol, which can contribute to its beneficial properties [Citation19,Citation20].
This study intended to evaluate the growth performance, lipid profile and other biochemical and histopathological alterations in adult healthy rats that consumed dietary lipids from animal or vegetable sources.
Materials and methods
Animals
The current study used adult 24 male healthy albino rats with an initial body weight of 80–90 g purchased from the Egyptian Institute for Vaccine production and Serology, Helwan, Egypt. They were kept in groups of four per cage. The cages contain wood-chip bedding, renewed daily in a suitable animal house at room temperature (22 ± 2°C) under a 12-h light dark cycle and 40%–60% relative humidity. Rats were permitted an adequate normal laboratory rodent diet and were given water ad libitum for a period of 1 week before the experiment for adaptation. The body weight of rats was recorded weekly.
Ethical consideration
All rats within this study were maintained in the animal care facilities based on ‘Research Ethics Committee’ Mansoura University, Mansoura, Egypt, in accordance with the Institutional Animal Ethics Committee (IAEC) of Mansoura University (Code Number: Sci-Z-Ph-2021–75).
Diet preparation
Postmortem samples of animal-derived fat were taken from the carcass of a female domestic buffalo (Bubalus bubalis) bred for normal food consumption. Subcutaneous rump fats were obtained from the rump region of a female domestic buffalo (Bubalus bubalis). Subcutaneous rump fat that is present in between muscle and skin was collected from the middle gluteal area 10 cm caudal to the sacrum. Peri-kidney fats were obtained from the region around the kidney of the same domestic buffalo.
Corn oil and olive oil samples were obtained commercially from a local market (Afia International Company, Egypt) in high purity. Each experimental diet was prepared by mixing either melted animal fat or vegetable oil with normal commercial rodent pellet to contain 7% (w/w) of melted animal fats or vegetable oils [Citation21]. The 7% diets were freshly prepared by mixing 7 g olive oil, corn oil, melted kidney fat or melted rump fat to 93 g rat pallet manually, and were then left at room temperature overnight to absorb vegetable oils or animal fats until they become homogeneous in dough-like consistency.
Animals grouping
male Albino rats were equally categorized into four groups (six rats/each) as follows:
Corn oil group, rats consumed a diet supplemented with 7% fresh corn oil for 8 weeks.
Olive oil group, rats received a diet supplemented with 7% fresh olive oil for 8 weeks.
Subcutaneous rump fat group, rats supplemented with a diet containing 7% animal rump fat for 8 weeks.
Peri-kidney fat group, rats consumed a diet containing 7% animal Peri-kidney fat for 8 weeks.
Blood and tissue sample collection
At the end of the experimental period (8 weeks), rats were fasted overnight. Thereafter, rats were anesthetized by ketamine xylazine mixture, then were sacrificed. Immediately after collecting blood by cardiac puncture, animals were dissected, hepatic and cardiac tissues from each rat was removed, washed with a saline solution (0.9%) and weighed. A known weight (0.2 g) of each was homogenized in ice-cold Tris buffer (pH 7.3) using a high-speed homogenizer (DI18 basic, IKA, Germany) surrounded with an ice jacket to give a 10% w/v homogenate, and then kept frozen at −200°C until being analyzed. The remaining portions of liver and heart were fixed in 10% neutral formalin, then dehydrated in an ascending series of ethanol, then cleared with xylene and embedded in paraffin wax. The prepared tissue blocks were sectioned using a microtome on glass slides (5 µ) and stained with Hematoxylin and Eosin then examined for histopathological alterations using a light microscope [Citation22].
Investigated parameters
Estimations of albumin, bilirubin, TLs, total cholesterol (TC), HDLC, TG concentrations, AST and ALT activities were performed using Kits purchased from Biodiagnostic Company, Giza, Egypt. Activity of LDH was estimated using kits purchased from BioMed diagnostic company, Badr City, Egypt. Activity of GGT was estimated according to the manufacturer’s instructions of GGT ELISA Kit Catalog No. NK1120FY100 of Creative Biolabs Company, USA. Serum CK-MB activity was estimated according to the manufacturer’s instructions of Rat CK-MB ELISA Kit Catalog No. MBS2515061 of MyBiosource Company, USA. Serum cardiac troponin I (CTn-I) concentration was estimated according to the manufacturer’s instructions of Rat CTn-I ELISA Kit Catalog No. CSB-E08594r of CUSABIO Company, China. The value of very low-density lipoprotein cholesterol (vLDLC) was calculated according to the following equation: vLDLC = TG/5. Concentration of LDLC was calculated using the following equation: LDLC=TG/5. The value of the atherogenic index (Al) was calculated using the following equation:
.
Statistical analysis
The results of this study were expressed as means ± SEM. The significance of the experimental data was performed by one-way analysis of variance, then followed by the least significant difference (LSD) test, using GraphPad prism 9.0 software. The data were considered statistically significant at P < 0.05 [Citation23].
Results
Body and organs weight change
As indicated in , administration of corn oil, olive oil, rump fat and peri-kidney fat to normal rats for 8 weeks showed non-significant differences in body weight gain, absolute or relative weights of liver and heart between all treated groups.
Table 1. Body weight, absolute and relative liver and heart weight changes in the different rat groups.
Changes in serum TL, TC, TG concentration
As shown in , serum TC concentration was significantly increased (p < 0.05) in peri-kidney fats enriched diet receiving group when compared with other groups. In addition, a remarkable increase (p < 0.05) in serum TL and TG concentrations was observed in animal fat enriched diet receiving groups compared to vegetable oil enriched diet receiving groups.
Figure 1. Effect of vegetable and animal fat consumption on; (i): Serum TC (mg/dl), (ii): Serum TL (mg/dl), (ii): Serum TG (mg/dl). (a) significantly different with corn oil group.
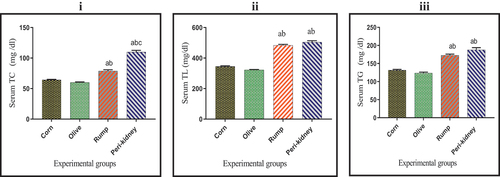
Changes in liver TC and TL content
As shown in , the liver TC content was significantly increased (p < 0.05) in peri-kidney fat enriched diet receiving group with respect to all other groups. A significant increase (p < 0.05) was recorded in liver TL content in animal fat enriched diet receiving groups with respect to vegetable oil enriched diet receiving groups.
Figure 2. Effect of vegetable and animal fat consumption on; (i): Liver TC (mg/g), (ii): Liver TL (mg/g). (a) significantly different with corn oil group, p < 0.05. (b) significantly different with olive oil group, p < 0.05. (c) significantly different with rump fat group.
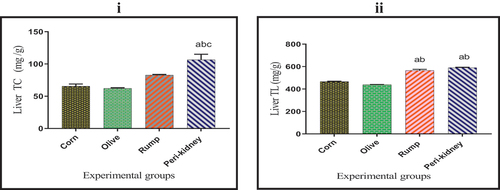
Changes in serum HDLC, LDLC and vLDLC concentration
The obtained results in show a significant increase (p < 0.05) in serum HDLC concentration in vegetable oil enriched diet receiving groups compared with animal fat enriched diet receiving groups. In the meantime, a marked decrease (p < 0.05) was observed in serum LDL-C and vLDLC concentrations as well as AI in vegetable oil enriched diet receiving groups when compared with animal fat enriched diet receiving groups. A significant decrease (p < 0.05) was recorded in serum LDLC concentration and AI in the rump fat enriched diet receiving group compared to the peri-kidney fat enriched diet receiving group.
Figure 3. Effect of vegetable and animal fat consumption on; (i): Serum HDLC (mg/dl), (ii): Serum LDLC (mg/dl), (iii): Serum vLDLC (mg/dl), (iv): Atherogenic index (ai).(a) significantly different with corn oil group, p < 0.05. (b) significantly different with olive oil group, p < 0.05. (c) significantly different with rump fat group, p < 0.05. Values are expressed as means ± SEM; (n =6) for each group.
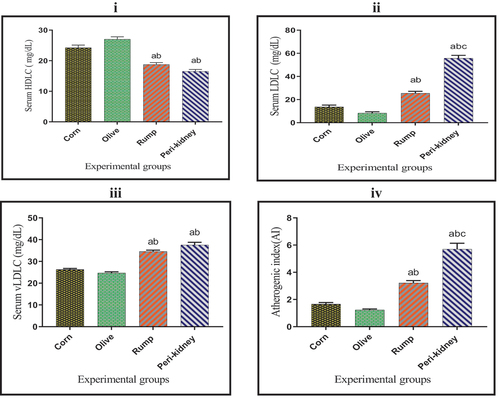
Changes in serum CK-MB, LDH and CTn-I values
The results in reveal a significant increase in serum CK-MB and LDH activities in animal fat enriched diet receiving groups compared to vegetable oil enriched diet receiving groups. In addition, a significant decrease was observed in serum CTn-I value in olive oil group relative to other groups. Otherwise, serum CTn-I value was significantly increased in the peri-kidney fat group compared to other groups. Serum CTn-I value in rump fat group was significantly increased compared to the corn oil group.
Figure 4. Effect of vegetable and animal fat consumption on; (i): Serum CK MB (pg/ml), (ii): Serum LDH (u/l), (ii): Serum CTn-I (pg/ml). (a) significantly different with corn oil group, p< 0.05. (b) significantly different with olive oil group, p < 0.05. (c) significantly different with rump fat group, p < 0.05. Values are expressed as means± SEM; (n =6) for each group.
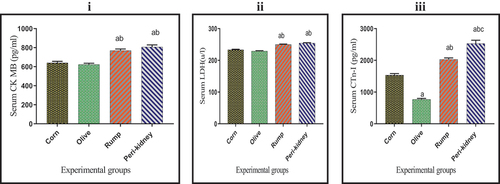
Effect of fat consumption on serum and liver ALT, AST, and GGT values
Results in show that, serum ALT, AST, GGT as well as liver ALT and AST activities were significantly increased (p < 0.05) in peri-kidney fat rich diet receiving group compared to other groups. Animal fat enriched diet receiving groups recorded a marked increase (p < 0.05) in serum, liver ALT and GGT activities compared to vegetable oil enriched diet receiving groups.
Figure 5. Effect of vegetable and animal fat consumption on; (i): Serum ALT (mg/dl), (ii): Serum AST (mg/dl), (ii): Serum GGT (mg/dl). (iv): Liver ALT (mg/g), (v): Liver AST (mg/g), (vi): Liver GGT (mg/g). (a) significantly different with corn oil group, p< 0.05. (b) significantly different with olive oil group, p < 0.05. (c) significantly different with rump fat group, p < 0.05. Values are expressed as means± SEM; (n =6) for each group.
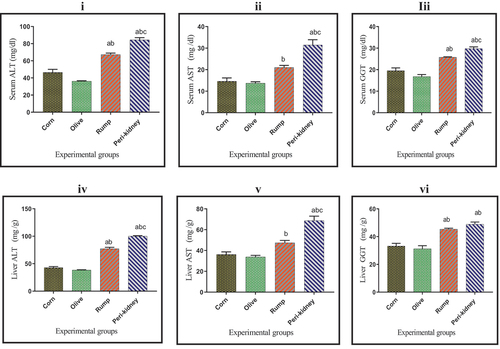
Effect of fat consumption on serum bilirubin and albumin concentrations
As indicated in , a significant increase (p < 0.05) was recorded in serum bilirubin concentration in animal fat enriched diet receiving groups compared to vegetable oil enriched diet receiving groups. Meanwhile, serum albumin concentration was significantly decreased (p < 0.05) in animal fat enriched diet receiving groups with respect to vegetable oil enriched diet receiving groups.
Figure 6. Effect of vegetable and animal fat consumption on; (i): Serum bilirubin (mg/dl) (ii): Serum albumin (mg/dl). (a) significantly different with corn oil group, p< 0.05. (b) significantly different with olive oil group, p < 0.05. (c) significantly different with rump fat group, p < 0.05. Values are expressed as means± SEM; (n =6) for each group.
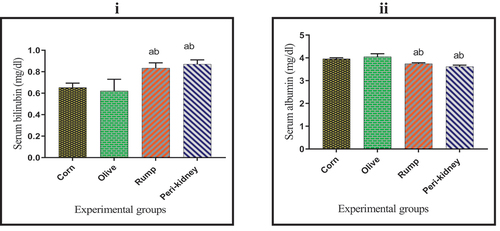
Effect of fat consumption on liver histopathology
Histopathological observations of liver sections excised from the corn oil enriched diet receiving group showed quite normal liver with crescent apoptotic cells and slight inflammatory manner (). Liver sections from rats fed on olive oil revealed normal histopathological architecture (fig. 7c&d). However, liver tissue dissected from rump fat fed rats () revealed sub-chronic changes, as marked dilatation in blood sinusoids, portal and central veins accompanied by perivascular necrosis and inflammatory infiltration. Hepatocytes were suffering from micro- and macro-vesicular fat accumulation with nuclear apoptotic polymorphism. Liver sections from the peri-kidney fat enriched diet receiving group depict dilatation in central vein and blood sinusoids with focal hemorrhages here and there in the tissue. In hepatocytes with different mitotic figures, early and late apoptotic nuclear features were observed ().
Figure 7. a&b: Photomicrographs of liver section from corn oil fed rats showing hepatic cords (hc), hepatocytes (h), crescent-shaped nucleus (yellow arrow), central vein (cv), monocytes infiltrated (green arrow), blood sinusoids (bs), Kupffer cells (k), bile duct (bd). c&d: liver sections from olive oil fed rats showing hepatic cords (hc), hepatocytes (h), central vein (cv), blood sinusoids (bs), Kupffer cells (k), bile duct (bd). e&f: liver section from rump fat fed rats revealing biliary hyperplasia (bd), macro-vesicular hepatocyte (f), pyknotic nuclei (red arrow), Kupffer cells (k), central vein (cv), blood sinusoids (bs), zonal necrosis (curved arrow), leucocytic infiltration (green arrow), hepatocytes with apoptotic nuclei (red arrow), crescent-shaped nucleus (yellow arrow). g&h: peri-kidney-fat fed rats depicts hepatic cords (hc), dilated central vein (cv), focal hemorrhage here and there in the tissue (blue arrow), inflammatory cells invasion (green arrow), vacuolated hepatocyte (v), pyknotic (red arrow), karyorrhexis (arrowhead). (X100 in right panel, X400 in left panel, X1000 in insets of F&H photomicrographs, H&E).
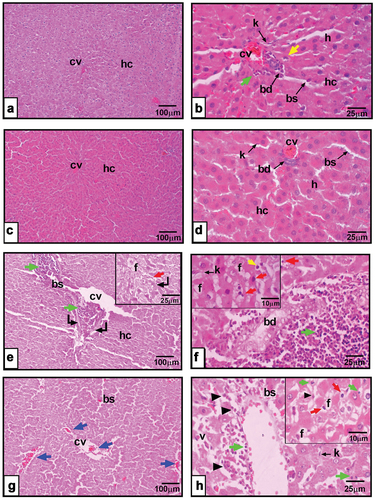
Effect of fat consumption on cardiac histopathology
Microscopic observations of olive oil fed animals revealed intact anastomosing cardiac myofibers with eosinophilic sarcoplasm, single oval centrally located nuclei of cardiomyocytes, and normal amount of interstitial connective tissue with abundant fibroblasts (). Meanwhile, feeding rats on a corn oil-rich diet revealed minor abnormalities such as a little separation in myofibers with homogenous highly acidophilic sarcoplasm (). Otherwise, cardiomyopathies are more frequently found in rump-fat fed rats. Loss of cellular detail is apparent in the areas of pallor multifocal necrosis of cardiomyocyte together with gradual replacement by fibroplasia and myofiber vacuolation. Hyaline cardiomyocyte hyper-eosinophilia of pre-acute injury could be noticed (). Moreover, animals fed on peri-kidney fat rich diet depicted distorted cardiac architecture in the form of remarkably separated myofibers, fibrosis, congested and dilated thick-walled blood vessels, extravasation and pyknotic nuclei were noted ().
Figure 8. a&b: Photomicrographs of cardiac sections from corn oil fed rats showing slight separation (s), fibrosis of the myofibers (*), cardiomyocytes (black arrow), fibroblasts (blue arrow). c&d: cardiac sections from olive oil fed rats revealing branched myofibers, cardiomyocytes with centrally located oval nuclei (black arrow), fibroblasts lining the interstitial tissue (blue arrow). e&f: heart sections from rump fat fed rats depicting widely separated hyalinized myofibers(s), homogenous highly acidophilic sarcoplasm (green arrow), focal to multifocal necrosis (curved arrow), fibroplasia (*), pyknotic cardiomyocytes (red arrow). g&h: heart sections represent peri-kidney-fat fed rats showing disarrayed separated myofibers (s), pyknotic nuclei (red arrow), dilated congested blood vessels (yellow arrow), thick wall and extravasation (ex), inflammatory infiltration (orange arrows). (X100 in right panel, X400 in left panel, H&E).
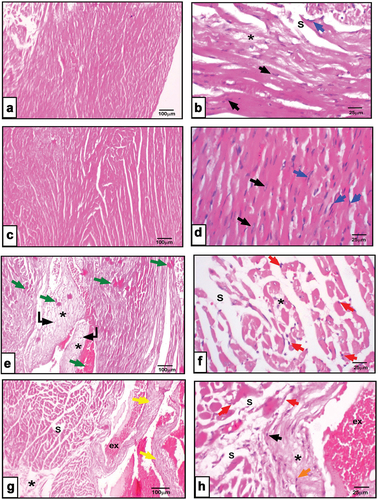
Discussion
The current study has investigated the effects of fats from animal or vegetable sources on lipid profile, liver and heart changes in healthy rats. Administration of corn oil; olive oil; peri-kidney fat and rump fat to rats did not exhibit any significant change in the body weight gain, absolute and relative liver and heart weights between all treated groups, these results run parallel with previous findings of Eka-Pratiwi et al. [Citation24] who found non-significant difference in body weight gain of rats consumed coconut oil or corn oil for 28 days. Similarly, these results are in accordance with Matsuo et al. [Citation25] who observed that rats fed vegetable oils or animal fats showed the same body weight gain after 2 months. These results might be mainly due to the similar level of flavor, juiciness, and general palatability, daily feed intake (FI) and feed efficiency of the rats when consumed vegetable oil or animal fat diets.
The present study found that, dietary animal fats contributed actively in HDLC depression and elevated TG, TC, VLDLC and LDLC concentrations in serum and liver of rats. These findings run parallel with previous results among several animal models [Citation13,Citation26]. Regarding, the effect of animal fats mainly peri-kidney fat rich in SFA and cholesterol on serum lipid profile, the present results agree with Mensink [Citation27] who reported that, SFA caused a significant elevation in serum TC, TG, TL, LDLC, AI and decreased HDLC concentrations. These results may be due to the low PUFA and high SFA proportions in animal fats mainly peri-kidney fat [Citation13,Citation28].
On the other side, plant-derived fat enriched diet receiving groups significantly reduced serum TC, TG and LDLC concentration as well as AI compared to animal-derived fat enriched diet receiving groups. These findings are in agreement with Dauqan et al. [Citation29] who found that, vegetable oil consumption decreased serum TC concentration in rats for 2 months, these results may be based on the fact that vegetable oil rich in vital constituents such as USFAs and phytosterols that have hypolipidemic effects.
Olive oil contains up to 70–80% MUFA, mostly in the form of OA and several antioxidant components, including phenolic compounds that could partially account for the observed beneficial effects [Citation30]. The valuable effects of olive oil in decreasing serum TC, TG and LDLC may be related to increased LDLC catabolic rate and decreased LDL oxidation in addition to inhibition of cholesterol transport-related proteins expression and lipogenic enzyme activity such as fatty acid synthase (FAS) [Citation31,Citation32]. Intensive olive oil intake is associated with increased PUFAs such as α-linolenic acid (ALA), eicosapentaenoic (EPA), docosahexaenoic (DHA) and arachidonic (AA) acids, which are considered as the main components of biological membranes, and play important roles in physiological processes [Citation33]. Oleoyl-ethanolamide (OEA) form is an OA-derivative, acts as an endogenous ligand of the peroxisome proliferator-activated receptor alpha (PPARα) nuclear receptor, and can be used as a potential therapeutic agent for the treatment of chronic disease such as obesity by regulating dietary fat intake and energy homeostasis [Citation34,Citation35]. Furthermore, OA activates silent information regulator 1, sirtuin 1 (SIRT1) which has a major role in stopping inflammation and oxidative stress related to liver and heart disorders. The direct substrate of SIRT1 is the transcriptional co-activator peroxisome proliferator-activated receptor γ co-activator 1-α (PGC1α), which becomes deacetylated and hyperactive after OA treatment. This substrate increases genes associated with FA oxidation via SIRT1-PGC1α-related mechanism [Citation36]. An additional novel mechanism suggested for OA to ameliorate atherogenicity and hepatocellular lipotoxicity is the suppression of the reticulum stress pathway and pyroptosis [Citation37].
In the current study, serum and liver TC, LDLC and TG values were significantly decreased in rats that consumed corn oil diet compared to rats that received animal fat diets. These results are consistent with Mandimika et al. [Citation38] who found that, rats fed corn oil recorded significant decrease in serum TC, TG and LDLC concentrations with respect to control group. These findings may be attributed to its relatively high concentration of PUFA, especially LA and other vital components such as phytosterols that can inhibit cholesterol absorption in the gastrointestinal tract (GIT) [Citation39]. Furthermore, the protective effects of corn oil may be related to the inhibition of hepatic expression of genes responsible for lipid metabolisms such as carnitine palmitoyltransferase I (CPT1-α), which is considered to be a rate limiting gene in FA β- oxidation; resulting in increased cholesterol catabolism and decreased lipid synthesis [Citation40–42].
In the present study, animal fat enriched diets provoked disturbances in liver and heart function tests. These findings are in accordance with dos Santos Lacerda et al. [Citation43] who found a significant increase in liver enzyme activities in rats consumed enriched SFA diet with respect to control group. Panchal et al. [Citation44] found that, the diets rich in SFA and/or cholesterol resulted in increased fat deposition, inflammation and fibrosis as well as increased liver enzyme activities in experimental rats. The marked increase in liver and heart enzymes in the blood of animal fat enriched diet receiving groups may be due to the accumulation of lipids causing cellular damage and cytotoxicity [Citation41]. Marques et al. [Citation45] observed that diets rich in SFA decreased serum albumin concentration. This could be associated with abnormalities in the renal system as a result of dyslipidemia [Citation46,Citation47].
Histopathological observations of liver sections excised from rats consumed vegetable oils revealed normal liver architecture. However, liver tissue dissected from rump fat fed animals depict dilatation in central vein and blood sinusoids with focal hemorrhages here and there in the tissue. Hepatocytes with different mitotic figures and early and late apoptotic nuclear features were observed in liver sections excised from rats fed prei-kidney fat. These observations agreed with previous studies that recorded hepatic alterations in rats fed animal fat diet represented in elevated amounts of hepatic stellate cells, hepatocellular fat deposition and abnormal fat vacuoles [Citation48,Citation49]. These abnormal fat vacuoles are markers of deterioration in lipid metabolism, and their occurrence may suppose liver aberration [Citation50].
Microscopic observations of cardiac samples excised from olive oil fed animals revealed intact anastomosing cardiac myofibers with eosinophilic sarcoplasm, single oval centrally located nuclei of cardiomyocytes and normal amount of interstitial connective tissue with abundant fibroblasts. Meanwhile, feeding rats on a corn oil-rich diet revealed minor abnormalities such as a little separation in myofibers with homogenous highly acidophilic sarcoplasm. Otherwise, animal fed on rump-fat rich diet depicted distorted cardiac architecture in the form of remarkably separated myofibers, fibrosis, congested and dilated thick-walled blood vessels, extravasation and pyknotic nuclei were noted. Moreover, cardiomyopathies are more frequently found in peri-kidney fat fed rats. Loss of cellular detail is apparent in the areas of pallor multifocal necrosis of cardiomyocyte together with gradual replacement by fibroplasia and myofiber vacuolation. Hyaline cardiomyocyte hyper-eosinophilia of pre-acute injury could be noticed. These results run in parallel with Abdel-Halim et al. [Citation51] and Rus et al. [Citation52] who reported that, vegetable oils (flaxseed, corn and olive oils) have cardioprotective impacts on the amelioration of acute myocardial infarction.
Conclusion
This work has shown that, dietary intake of vegetable oils, mainly olive oil, exerted biochemical markers and histological observations better than the animal fats mainly peri-kidney fat. The advantageous effects of vegetable oils represented in the line of excess serum HDLC and albumin concentrations as well as the positive effect of reducing liver and heart disorders as evidenced by decreased serum TG, TC and LDLC concentrations as well as liver and heart enzyme activities. As a result of this, in order to protect the liver and the heart, the study suggests consumption of olive oil enriched diets.
Disclosure statement
No potential conflict of interest was reported by the authors.
References
- Jayedi A, Shab-Bidar S. Fish consumption and the risk of chronic disease: an umbrella review of meta-analyses of prospective cohort studies. Adv Nutr. 2020;11(5):1123–1133. doi: 10.1093/advances/nmaa029
- Feldman D, Huggins S, Norwitz NG. Short-term hyper-caloric high-fat feeding on a ketogenic diet can lower low-density lipoprotein cholesterol: the cholesterol drop experiment. Curr Opin Endocrinol Diabetes Obes. 2022;29(5):434–439. doi: 10.1097/MED.0000000000000762
- Durmuş M. Fish oil for human health: omega-3 fatty acid profiles of marine seafood species. Food Sci Technol. 2019;39(2):454–461. doi: 10.1590/fst.21318
- Bogie JFJ, Haidar M, Kooij G, et al. Fatty acid metabolism in the progression and resolution of CNS disorders. Adv Drug Deliv Rev. 2020;159:198–213. doi: 10.1016/j.addr.2020.01.004
- Kawecka A, Radkowska I, Sikora J. Concentrations of selected bioactive components in traditional cheeses made from goat’s, cow’s and sheep’s milk. J Elem. 2020;25:431–442. doi: 10.5601/jelem.2019.24.3.1907
- Kendrick M. Assessing cardiovascular disease: looking beyond cholesterol. Curr Opin Endocrinol Diabetes Obes. 2022;29(5):427–433. doi: 10.1097/MED.0000000000000761
- Cerone M, Smith TK. A brief journey into the history of and future sources and uses of fatty acids. Front Nutr. 2021;8:1–15. doi: 10.3389/fnut.2021.570401
- Schuldt L, Von Brandenstein K, Jacobs C, et al. Oleic acid-related anti-inflammatory effects in force-stressed PdL fibroblasts are mediated by H3 lysine acetylation associated with altered IL10 expression. Epigenetics. 2022;17(13):1892–1904. doi: 10.1080/15592294.2022.2090654
- Razmaitė V, Šiukščius A, Šveistienė R, et al. Relationships between fat and cholesterol contents and fatty acid composition in different meat-producing animal species. Acta Veterinaria-Beograd. 2020;70(3):1–12. doi: 10.2478/acve-2020-0028
- Wang B, Tian G, Zhang Q. Vegetable oil or animal fat oil, which is more conducive to cardiovascular health among the elderly in China? Curr Probl Cardiol. 2023;48(2):101485. doi: 10.1016/j.cpcardiol.2022.101485
- Schumacher M, DelCurto-Wyffels H, Thomson J, et al. Fat deposition and fat effects on meat quality—A review. Animals. 2022;12(12):1550–1566. doi: 10.3390/ani12121550
- Dinh TTN, To KV, Schilling MW. Fatty acid composition of meat animals as flavor precursors. Meat Muscle Biol. 2021;5(1):1–16. doi: 10.22175/mmb.12251
- Martínez E, Pardo JE, Rabadán A, et al. Effects of animal fat replacement by emulsified melon and pumpkin seed oils in deer burgers. Foods. 2023;12(6):1279–1292. doi: 10.3390/foods12061279
- Merdan SM, El-Zarei MF, Ghazy AA, et al. Genetic differentiation between Egyptian buffalo populations using microsatellite markers. J Of Animal, Poultry & Fish Production; Suez Canal University. 2019;8(1):21–28.
- Zhang B, Zeng M, Wang Y, et al. Oleic acid alleviates lps-induced acute kidney injury by restraining inflammation and oxidative stress via the Ras/MAPKs/PPAR-γ signaling pathway. Phytomedicine. 2022;94:1–12. doi: 10.1016/j.phymed.2021.153818
- Navas PB, Carrasquero-Durán A. Chemical and sensory characterization of corn oil flavoured by citrus. Braz J Food Technol. 2020;23:1–11. doi: 10.1590/1981-6723.05419
- Kostik V, Memeti S, Bauer B. Fatty acid composition of edible oils and fats. J Hyg Eng Des. 2013;4:112–116.
- Hamid R, Hag Husein H, Bäumler R. Characteristics of some wild olive phenotypes (oleaster) selected from the western mountains of Syria. Sustainability. 2022 18;14(9):5151–5164. doi: 10.3390/su14095151
- Farràs M, Almanza-Aguilera E, Hernáez Á, et al. Beneficial effects of olive oil and Mediterranean diet on cancer physio-pathology and incidence. Semin Cancer Biol. 2021;73:178–195. doi: 10.1016/j.semcancer.2020.11.011
- Santa-María C, López-Enríquez S, Montserrat-de la Paz S, et al. Update on anti-inflammatory molecular mechanisms induced by oleic acid. Nutrients. 2023;15(1):224–239. doi: 10.3390/nu15010224
- Nogoy KMC, Kim HJ, Lee DH, et al. Oleic acid in Angus and Hanwoo (Korean native cattle) fat reduced the fatty acid synthase activity in rat adipose tissues. J Anim Sci Technol. 2021;63(2):380–393. doi: 10.5187/jast.2021.e4
- Bancroft JD, Gamble M . Theory and practice of histological techniques. Elsevier Health Sci. 2008;6:433–473.
- Snedecor CW, Cochran WC. Statistical methods. 7th ed ed. Ames, Iowa: Iowa state univ press; 1980. p. 593.
- Eka-Pratiwi I, Soetjiningsih S, Mantik-Astawa I, et al. Virgin coconut oil compared to corn oil in world health organization formula on glutathione, TNF-α, and body weight gain in severe malnourished Wistar rat. Bali Medical J. 2016;5(3):453–456. doi: 10.15562/bmj.v5i3.321
- Matsuo T, Takeuchi H, Suzuki H, et al. Body fat accumulation is greater in rats fed a beef tallow diet than in rats fed a safflower or soybean oil diet. Asia Pac J Clin Nutr. 2002;11(4):302–308. doi: 10.1046/j.1440-6047.2002.00299.x
- Lee Y, Kim BR, Kang GH, et al. The effects of PPAR agonists on atherosclerosis and nonalcoholic fatty liver disease in ApoE−/−FXR−/− Mice. Endocrinol Metab. 2021;36(6):1243–1253. doi: 10.3803/EnM.2021.1100
- Mensink RP. Effects of saturated fatty acids on serum lipids and lipoproteins: a systematic review and regression analysis. Geneva, World Health Organization. 2016 .
- Garcia JP, Cézar JS, Lemos BS, et al. Effects of high fat diet on kidney lipid content and the Na, K-ATPase activity. Braz J Pharm Sci. 2018;54(1):1–13. doi: 10.1590/s2175-97902018000117165
- Dauqan E, Sani HA, Abdullah A, et al. Effect of different vegetable oils (red palm olein, palm olein, corn oil and coconut oil) on lipid profile in rat. Food Nutr Sci. 2011;2(4):253–258. doi: 10.4236/fns.2011.24036
- Xu J, Feng ZP, Peng HY, et al. Omega-3 polyunsaturated fatty acids alleviate adenine-induced chronic renal failure via regulating ROS production and TGF-β/SMAD pathway. Eur Rev For Med Pharmacol Sci. 2018;22:5024–5032.
- Tutunchi H, Asghari-Jafarabadi M, Hoojegani S, et al. General and abdominal obesity is related to socioeconomic status and food choices: a cross-sectional study. Nutr Food Sci. 2020a;50(1):61–73. doi: 10.1108/NFS-02-2019-0056
- Tutunchi H, Ostadrahimi A, Saghafi-Asl M. The effects of diets enriched in monounsaturated oleic acid on the management and prevention of obesity: a systematic review of human intervention studies. Adv Nutr. 2020b;11(4):864–877. doi: 10.1093/advances/nmaa013
- Nogoy KMC, Kim HJ, Lee Y, et al. High dietary oleic acid in olive oil-supplemented diet enhanced omega-3 fatty acid in blood plasma of rats. Food Sci Nutr. 2020;8(7):3617–3625. doi: 10.1002/fsn3.1644
- Yang ZH, Nill K, Takechi-Haraya Y, et al. Differential Effect of dietary supplementation with a soybean oil enriched in oleic acid versus linoleic acid on plasma lipids and atherosclerosis in LDLR-Deficient mice. Int J Mol Sci. 2022;23:8385. doi: 10.3390/ijms23158385
- Tutunchi H, Ostadrahimi A, Saghafi-Asl M, et al. Expression of NF-κB, IL-6, and IL-10 genes, body composition, and hepatic fibrosis in obese patients with NAFLD—Combined effects of oleoylethanolamide supplementation and calorie restriction: a triple-blind randomized controlled clinical trial. J Cell Physiol. 2021;236(1):417–426. doi: 10.1002/jcp.29870
- Yang Y, Liu Y, Wang Y, et al. Regulation of SIRT1 and its roles in inflammation. Front Immunol. 2022;13:1–16. doi: 10.3389/fimmu.2022.831168
- Zeng X, Zhu M, Liu X, et al. Correction to: Oleic acid ameliorates palmitic acid induced hepatocellular lipotoxicity by inhibition of er stress and pyroptosis. Nutr Metab. 2020;17(1):1–18. doi: 10.1186/s12986-020-00438-y
- Mandimika T, Paturi G, De Guzman C, et al. Effects of dietary broccoli fiber and corn oil on serum lipids, fecal bile acid excretion and hepatic gene expression in rats. Food Chem. 2012;131(4):1272–1278. doi: 10.1016/j.foodchem.2011.09.117
- Nicholls SJ, Lincoff AM, Garcia M, et al. Effect of high-dose omega-3 fatty acids vs corn oil on major adverse cardiovascular events in patients at high cardiovascular risk the strength randomized clinical trial. JAMA. 2020;324(22):2268–2280. doi: 10.1001/jama.2020.22258
- Rideout T, Harding S, Jones P, et al. Guar gum and similar soluble fibers in the regulation of cholesterol metabolism: current understandings and future research priorities. Vasc Health Risk Manag. 2008;4(5):1023–1033. doi: 10.2147/VHRM.S3512
- Yao M, Zhou P, Qin YY, et al. Mitochondrial carnitine palmitoyltransferase-II dysfunction: a possible novel mechanism for nonalcoholic fatty liver disease in hepatocarcinogenesis. World J Gastroenterol. 2023;29(12):1765–1778. doi: 10.3748/wjg.v29.i12.1765
- Trabjerg MS, Andersen DC, Huntjens P, et al. Inhibition of carnitine palmitoyl-transferase 1 is a potential target in a mouse model of Parkinson’s disease. Npj Parkinsons Dis. 2023;6(1):1–26. doi: 10.1038/s41531-023-00450-y
- dos Santos Lacerda D, de Almeida MG, Teixeira C, et al. Biochemical and physiological parameters in rats fed with high-fat diet: the protective effect of chronic treatment with purple grape juice (Bordo Variety). Beverages. 2018;4(4):100–112. doi: 10.3390/beverages4040100
- Panchal SK, Poudyal H, Iyer A, et al. High-carbohydrate, high-fat diet-induced metabolic syndrome and cardiovascular remodeling in rats. J Cardiovasc Pharmacol. 2011;57(5):611–624. doi: 10.1097/FJC.0b013e3181feb90a
- Marques C, Meireles M, Norberto S, et al. High fat diet induced obesity rat model: a comparison between Wistar and Sprague-Dawley rat. Adipocyte. 2011;5(1):11–21. doi: 10.1080/21623945.2015.1061723
- Al-Hayder MN, Al-Mayyahi RS, Hraishawi RM. The effects of high-fat diet on kidney and lung histopathology in experimental rats. Open J Sci Technol. 2020;3(1):40–45. doi: 10.31580/ojst.v3i1.1357
- Dalal MM Potential role of gum Arabic on calcium homeostasis in adenine-induced chronic renal failure in rats. M. Sc. Thesis, Faculty of Science, Mansoura University, Mansoura, Egypt; 2019.
- Welch-White V, Dawkins N, Graham T, et al. The impact of high fat diets on physiological changes in euthyroid and thyroid altered rats. Lipids Health Dis. 2013;12(1):100–108. doi: 10.1186/1476-511X-12-100
- Nour MS, Sakara ZA, Hasanin NA, et al. Histological and immunohistochemical study of the effect of liraglutide in experimental model of non-alcoholic fatty liver disease. Egypt J Basic Appl Sci. 2023;10(1):342–357. doi: 10.1080/2314808X.2023.2204258
- Moreira RK. Hepatic stellate cells and liver fibrosis. Arch Pathol Lab Med. 2007;131(11):1728–1734. doi: 10.5858/2007-131-1728-HSCALF
- Abdel-Halim NH, Ghalwash M, Sarhan MMH, et al. Effect of flaxseed and corn oils on acute myocardial infarction in high fat diet fed rat. Mansoura Med J. 2016;45(1&2):47–62. doi: 10.21608/mjmu.2016.123883
- Rus A, Molina F, M J M-R, et al. Effects of olive oil consumption on cardiovascular risk factors in patients with fibromyalgia. Nutrients. 2020;12(4):918–930. doi: 10.3390/nu12040918