ABSTRACT
The fundamental concept of diabetes research is the restoration of normal glycemic status. Nowadays, nanoemulsion medication delivery technologies provide effective approaches to the second type of diabetes mellitus care. This study aimed to investigate the potential of metformin nanoemulsion (MTF-NE) in treating streptozotocin (STZ)-induced diabetic retinopathy in rats. Histopathological examinations, including hematoxylin and eosin, anti-F-actin, anti-CD57, and anti-S100 immunostains, were performed after 8 weeks. Many alterations in the STZ group as slight disorganization of retinal layers, loss of some photoreceptor processes, and focal widening of intercellular spaces between small darkly stained nuclear cells in inner and outer nuclear layers, focal areas of disruption of outer plexiform layer, pyknotic nuclei of ganglion cells, and dilated congested blood capillaries with vascular leakage in the ganglion cell layer, decreased F-actin, increased CD75 and S100 immunohistochemical expression. Rats treated with metformin nanoemulsion have substantial improvements in retinal organization, histopathology, and immunohistochemical markers compared to those treated with metformin. The study suggests that metformin nanoemulsion holds promise for alleviating diabetic retinopathy in rats on a histological and immunohistochemical basis.
Introduction
Diabetic retinopathy (DR), a common complication of diabetes, has been identified as a major cause of visual loss. The first stage of DR involves non-proliferative diabetic retinopathy, which is distinguished by frequent microaneurysms and retinal hemorrhages. The second stage, proliferative diabetic retinopathy, is brought about by increasing capillary non-perfusion, which leads to neovascularization. An increase in the vascular endothelial growth factor (VEGF) causes neovascularization and elevated vascular permeability in the retina [Citation1]. Diabetes is becoming more common each year, with estimated 451 million individuals suffering from the condition in 2017 with an anticipated rise of 693 million people by 2045 [Citation2]. Most diabetic patients with type −1 eventually acquire DR, and 50–80% of diabetes patients with type-2 will develop DR after 20–25 years of diabetes diagnosis [Citation3].
Streptozotocin was used because it is considered to be the best-known animal model for the evaluation of retinal complications in diabetes, and this agreed with a prior investigation that reported that STZ induces various biochemical and histological alterations that very closely mirror the first grades of diabetic retinopathy that occurs in people [Citation4]. STZ is a dangerous glucose analog that aggregates in pancreatic cells via uptake by glucose transporter, according to previous research. STZ breaks down into the glucose component and methyl nitrosourea moiety. The latter is a potent alkylating agent that causes numerous DNA strand breaks and fragmentation, resulting in cell damage [Citation5].
Anti-VEGF therapy and laser photocoagulation are presently considered standard-of-care procedures. While anti-VEGF medication may decrease vascular permeability and halt the course of DR, trials have demonstrated that it isn’t effective for all individuals, and that some patients' reaction to the therapy wanes over time [Citation6].
Metformin is indeed a biguanide-class medicine that is created and utilized as an oral hypoglycemic treatment to treat diabetes patients with type-2 across the world [Citation7]. Metformin lowers the pace at which blood glucose levels increase in muscle tissue, in addition to inhibiting hepatic gluconeogenesis as well as glycogenolysis, reducing glucose absorption from the stomach, and increasing peripheral tissue insulin sensitivity [Citation8]. MTF has been reported to stimulate insulin release by pancreatic tissue beta cells [Citation9].
A recent study indicated that metformin reduced the diabetic-induced retinopathy in terms of gliosis and apoptosis, and its mechanism may be due to the reduction of hyperglycemia and oxidative stress suppression [Citation10]. Another work reported that treating induced diabetic rats with (500 mg/kg/day) metformin for four weeks could partially correct the diabetic alterations but not to a healthy control level. So, metformin is not entirely effective on its own [Citation11].
Polymer nanoparticles possess sizes ranging from 1 to 1000 nanometers in diameter [Citation12]. Nanoparticle medicines utilized as biodegradable polymers are currently undergoing substantial research for an array of uses [Citation13]. Nanoparticles provide various benefits, such as increased therapeutic efficacy, prolonged and better drug release, fewer side effects, enhanced stability, and less drug breakdown [Citation14].
A recent literature showed that nano-metformin has a significantly higher anti-diabetic efficacy than metformin by altering the insulin signaling system and apoptotic gene expression [Citation15].
The goal of the study was to investigate the possible therapeutic impact of a nanoemulsion of metformin (MTF-NE) on retinal injury in experimentally diabetic rats versus metformin. This is an interesting and important work in the discipline of nanotherapy for relieving retinal disease associated with diabetes.
Materials and methods
Metformin® & Streptozotocin were bought from Sigma-Aldrich, whereas oleic acid and Tween 80 were obtained from Sigma-USA.
Metformin nanoemulsion formulation
Oleic acid is a fatty acid that occurs naturally and can be acquired from sources that are both animal- and plant-based. An ultrasonic homogenizer was used to create an oleic acid nanoemulsion. The oil phase, aqueous phase, and nonionic surfactants are all components of nanoemulsion. Under vortex for 5 minutes, the oil phase (5% v/v) was introduced into an aqueous phase containing 1 g of metformin with hydrophilic surfactant (Tween 80–1.68% v/v). The coarse emulsion was then homogenized using an ultrasonic homogenizer (BioLogics Ultrasonic Homogenizer Model 150 VT; BioLogics Inc., Manassas, VA, USA) for 10 minutes at 50% pulsed power and 30% pulsar rate to produce nanoemulsion [Citation16]. The metformin nanoemulsified particles were sterilized by passing them through a 0.22 m syringe filter and then tested for physical stability over time.
Nanoemulsion characterization
Metformin nanoemulsion droplets mean particle size, zeta potential, plus polydispersity indexes (PdI) were determined using a Zetasizer® Nano ZS from Malvern PCS Instruments in the UK. The measurement was carried out at 25°C with a scattering angle of 160.9°, a material refractive index of 1.40, and a viscosity (cP) of 0.8872 cPoise. In this case, the dispersant dielectric constant and zeta run were 78.5 and 16, respectively. The estimation sample was diluted 100 to 200 times before measurement, with 10 L of the sample added to 1 mL of ultrapure water [Citation17].
Experimental design and study groups
Adult male rats (Rattus norvegicus) weighing around 100 g +20 g were procured from the Medical Ain Shams Research Institute in Cairo, Egypt, and were kept under normal conditions throughout our study. To avoid diabetic coma during the experiment, all rats had restricted access to food and water. To overcome the drug-induced hypoglycemia, mice were given a 5% glucose solution to drink overnight. Diabetes is characterized by a random blood glucose level of greater than 300 mg/dl on three consecutive days after a 72-hour STZ injection. After 12 weeks, histological alterations indicated the presence of diabetic nephropathy. Treatment started four weeks following the STZ injection, and the animals were split across four groups of ten rats each:
CONT group: these rats received no treatment.
STZ group: Rats were fasted before receiving a single intraperitoneal dose of freshly produced STZ solution (60 mg/kg) mixed in cold citrate buffer (0.9%) [Citation18].
STZ+MTF group: diabetic animals received a daily dose of metformin (18 mg/200 g b.wt.) [Citation15].
STZ+ MTF-NE group consisted of diabetic rats that received daily intraperitoneal injections of metformin nanoemulsion (18 mg/kg b.wt.) [Citation15].
After a period of eight weeks of treatment, the animals were put under isoflurane anesthesia and died in order to perform histological experiments.
Histopathological and immunohistochemical study
Rats were euthanized and after that retinae were removed for histological analysis. The excised organs were then fixed in Bouin’s fixative for about 24 hours, rinsed in 70% alcohol then dehydrated, cleaned in xylene, and impregnated in parablast for blocking. Serial slices of 5 m thickness were produced and stained by hematoxylin and eosin [Citation19].
For immunohistochemistry staining, retinal sections (5 µm) were deparaffinized and rehydrated with falling alcohol concentrations. The retinal tissues were treated with 3% hydrogen peroxide (H2O2) to eliminate natural peroxidase-like activity. These portions were treated for 30 minutes in a boiling water bath with citrate buffer (0.01 M, pH 6.0). The sections were treated overnight at room temperature with primary antibodies: anti-F-actin (Abcam, ab1801, at 5 g/ml), pure mouse anti-human CD57 (dilution 1:30, clone HNK-1, BD Bioscience, San Jose, CA, USA), and polyclonal rabbit anti-S100 (dilution 1:400, DAKO, Glostrup, Denmark). After rinsing with phosphate-buffered saline, the streptavidin complex was applied, and the reactions were visible using diamine-benzidine (DAB) chromogen.
Morphometric analysis
Six non-overlapping high-power fields at a magnification of 400 were morphometrically assessed from each group of immunohistochemically stained sections of F-actin, CD57, and S100 using the Image J analyzer computer system (NIH, Maryland, USA). The percent of positively stained immune cells in retina was calculated. Each group’s data were quantitatively displayed as mean ± SD and statistically assessed.
Statistical analysis
The data are shown as the mean± standard deviation (SD). The statistical study was done using the statistical software ‘Prism version 7’. The analysis of variance (one-way ANOVA) was performed to compare different groups, followed by the Tukey test. Statistical significance was established when the P-value was less than 0.05 [Citation20].
Results
Nanoemulsion characterization
The average size of the metformin nanoemulsion was 291 nm, as illustrated in . The monodisperse droplet population can be detected by a reduced PDI value (close to zero), while a PDI value nearer to one (one) indicates a droplet population of varying sizes. Despite the fact that droplet size increased somewhat after this test, its PDI score remained at roughly 0.220, indicating that the droplet population was uniform in both formulations. Metformin nanoemulsion has a zeta potential of −24.9 . The projected zeta potential is adequate to produce a repellent barrier between metformin nanodroplets and stably scattered colloidal systems.
Histopathological observations
Examination of hematoxylin and eosin-stained sections of the CONT group shows a normal histological structure of well-organized retinal layers formed of three cellular layers and two synaptic layers. Cellular layers include ganglion cell layer (GCL), inner nuclear layer (INL), which has big nuclei, and the outer nuclear layer (ONL), which has densely packed dark nuclei. Synaptic layers are inner plexiform layer (IPL) along with the outer plexiform layer (OPL), which forms a loose reticular layer. Photoreceptor layer (PRL) of rods and cones cells and nerve fiber layer (NFL) were indicated .
Figure 2. A photomicrograph of the cross section of the rat retina in the experimental groups (hematoxylin and eosin stain). A: CONT group showing normal histological structure of well-organized retinal layers arranged from outward to inward as photoreceptor layer (PRL), outer nuclear layer (ONL) of nuclear cells (red arrow), outer plexiform layer (OPL), inner nuclear layer (INL) of nuclear cell (red arrow), inner plexiform layer (IPL), ganglion cell layer (GCL) of ganglion cells with large vesicular nuclei (yellow arrow), and nerve fiber layer (NFL). B: STZ group showing slight disorganization of retinal layers, loss of some photoreceptor processes (arrow head), focal widening of intercellular spaces (S) between small dark-stained nuclear cells (black arrows) in ONL and INL appearing as empty spaces between the nuclei, focal areas of disruption of OPL can be observed (blue arrow), pyknotic nuclei of ganglion cells (yellow arrow), and dilated congested blood capillary with vascular leakage (star) in GCL are observed. C: the STZ+MTF group exhibits slightly improved retinal pathology. Small empty spaces (S) between the nuclei of the INL with some shrunken pyknotic nucleui (black arrow), the thinning of the OPL, and some shrunken pyknotic nuclei of the ganglion cell in the GCL (red arrow) are present. D: STZ+ MTF-NE group showing marked improvement of the retinal pathology. Small empty spaces (S) between the nuclei of the INL and some dilated blood capillaries (stars) in the GCL are observed (scale bar = 50µm).
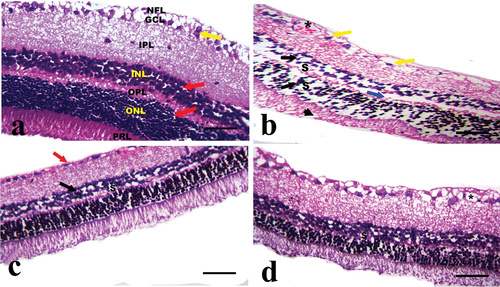
The retinae of diabetic animals (STZ group) displayed slight disorganization of retinal layers, loss of some photoreceptor processes, and focal widening of intercellular spaces between small darkly stained nuclear cells in the inner and outer nuclear layers, focal areas of disruption of the outer plexiform layer, ganglion cells’ pyknotic nuclei, and dilated congested blood capillaries with vascular leakage in the GCL .
The STZ+MTF group shows moderate improvement in retinal pathology. Small empty spaces between the nuclei of the inner nuclear layer with some shrunken pyknotic nuclei, thinning of the outer plexiform layer, and some shrunken pyknotic nuclei of ganglion cell in ganglion cell layer can be observed . The STZ+ MTF-NE group showed marked improvement in retinal pathology. Small empty spaces between the nuclei of INL and some dilated blood capillaries in ganglion cell layer can be observed .
Histoscoring of the thickness of the retina in H&E-stained sections showed a significant decrease in STZ and improvement of the retina’s thickness in all treated groups, with the STZ+ MTF-NE group showing a marked improvement in the retinal thickness compared to STZ+MTF and .
Figure 3. Morphometric analysis of retinal sections in the study groups. a) significance against the CONT group; b) significance against the STZ group; and c) significance of STZ+MTF-NE against the STZ+MTF group, p ≤ 0.05. A: STZ significantly reduced retinal thickness (µm), while treatment groups improved significantly. Additionally, the STZ+MTF-NE group showed a significant increase in thickness compared to the STZ+MTF group. B: the percentage of F-actin-positive cells decreased in the STZ group but increased significantly in the treatment groups. Furthermore, the STZ + MTF-NE group was much larger than STZ + MTF. C: the percentage of CD57-positive cells was considerably higher in the STZ group and lower in the treated groups. Furthermore, the STZ + MTF-NE group was much smaller than STZ + MTF. D: the area percent of S-100 positive cells was dramatically raised in STZ and improved in the treated groups, while it showed a significant drop in the treated groups. Furthermore, the STZ + MTF-NE group was significantly decreased than STZ+MTF.
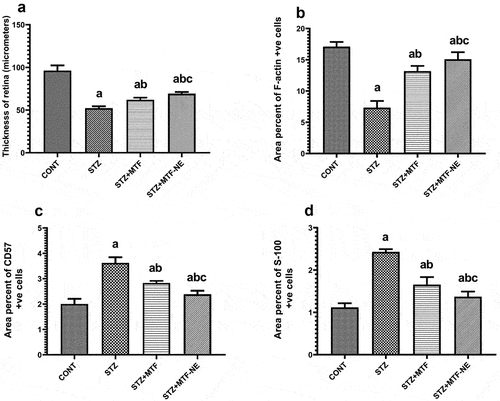
Table 1. Morphometric analyses of retinae sections in the study groups.
Immunohistochemistry
The demonstration of F-actin in the rat retina of the CONT group showed marked positive immunoreactions in PRL, INL, and GCL . While its demonstration in the STZ group shows mild positive expression in PRL, INL, and GCL . But after STZ+MTF and STZ+ MTF-NE treatment, they showed moderate positive immunoexpression in PRL, INL, and GCL . Morphometric estimation of the area percent of F-actin-positive cells showed a significant decrease in the STZ group and an improvement in their values in the treated groups. The STZ+ MTF-NE group showed a marked improvement in the area percent of this immune marker than STZ+MTF and .
Figure 4. A photomicrograph of the cross section of the rat retina in the experimental groups (anti-F-actin immunostain). A: CONT group showing marked positive expression (green arrow), especially in the photoreceptor layer (PRL), inner nuclear layer (INL), and ganglion cell layer (GCL). B: STZ group showing mild positive expression (green arrow), especially in PRL, INL, and GCL. C: STZ+MTF group showing mild to moderate positive expression (green arrow), especially in PRL, INL, and GCL. D: STZ+ MTF-NE group showing moderate to marked F-actin positive expression (green arrow), especially in PRL, INL, and GCL. (Scale bar = 50µm).
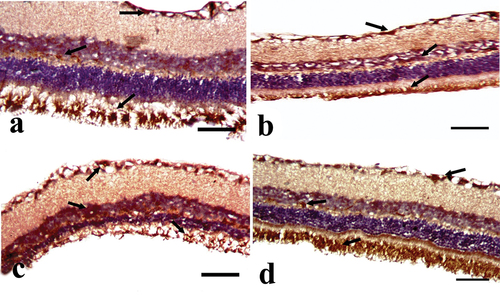
The detection of CD57 in the retinae of the CONT group showed a mild positive immunoreaction in the NFL and GCL . While its demonstration in the STZ group shows strong positive expression in the NFL and GCL . But after STZ+MTF and STZ+ MTF-NE treatment, they show moderate positive immunoexpression in the NFL and GCL . Morphometric estimation of the area percent of CD57-positive cells showed a significant increase in the STZ group and an improvement in their values in the treated groups. The STZ+ MTF-NE group showed a marked improvement in the area percent of this immune marker than STZ+MTF and .
Figure 5. A photomicrograph of the cross section of the rat retina in the experimental groups (anti-CD57 immunostained). A: the CONT group demonstrates modest positive expression (black arrow), particularly in the nerve fiber layer (NFL) and ganglion cell layer (GCL). B: the STZ group demonstrates strong positive expression (black arrow), particularly in the NFL and GCL. C: the STZ+MTF group demonstrates considerable positive expression (black arrow), particularly in the NFL and GCL. D: the STZ+ MTF-NE group has moderate CD57 positive expression (black arrow), particularly in the NFL and GCL. (Scale bar: 50µm).
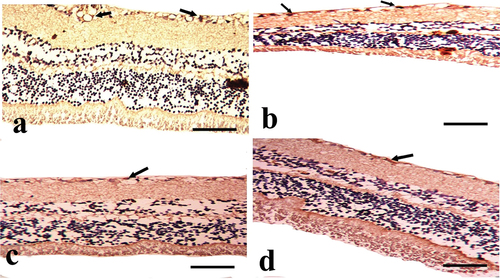
S100 detection in the retinae of the CONT group reveals a minor positive immunoreaction in the NFL and GCL . While its presentation in the STZ group demonstrates high positive expression in NFL, GCL, and INL . However, after STZ+MTF and STZ+ MTF-NE therapy, they exhibit moderate positive immunoexpression in NFL and GCL . The morphometric estimation of the area percent of CD57-positive cells shows a considerable increase in the STZ group and improvement in the treated groups. The STZ+ MTF-NE group showed a marked improvement in the area percent of this immunological marker than STZ+MTF and .
Figure 6. A photomicrograph of the cross section of the rat retina in the experimental groups (anti-S100 immunostain). A: CONT group showing mild positive expression (black arrow), especially in the nerve fiber layer (NFL) and ganglion cell layer (GCL). B: STZ group showing marked positive expression (black arrow), especially in NFL, GCL, and inner nuclear layer. C: the STZ+MTF group shows moderate positive expression (black arrow), especially in the NFL and GCL. D: STZ+ MTF-NE group showing moderate S-100 positive expression (black arrow), especially in NFL and GCL. (Scale bar = 50µm).
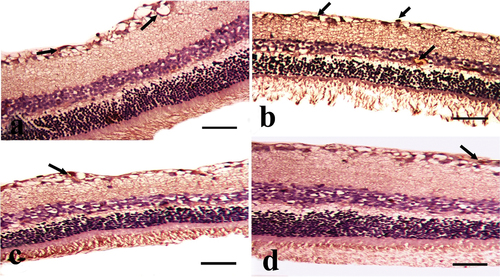
Discussion
The current study found slight disorganization of retinal layers, loss of some photoreceptor processes, and focal widening of intercellular spaces between small darkly stained nuclear cells in inner nuclear layer and outer nuclear layer, as well as focal areas of disruption of outer plexiform layer, pyknotic nuclei of ganglion cells, and dilated congested blood capillaries with vascular leakage in ganglion cell layer in diabetic animals (STZ group).
The underlying mechanism of diabetic retinopathy seems quite complex. Leukocyte adhesion causes inflammatory damage to endothelial cells, apoptosis, and the shedding of pericytes in addition to endothelial cells in the early phases. Damage to the inner blood-retinal barrier is caused by decreased endothelial tight junction protein and thickened basement membrane. This damages the retinal nerve’s glial inner layer and blood vessel functions, increasing blood vessel permeability and leakage of retinal tissue [Citation21,Citation22]. One of the body’s tissues that uses the most oxygen and energy is the retina [Citation23]. Rapid energy consumption causes an excess of reactive oxygen species (ROS), resulting in a confined, and highly oxidative microenvironment [Citation24]. Unreduced ROS damage retinal cells; this causes a pathogenic context of the eye. ROS are produced in the disorders, resulting in retinal degeneration [Citation25].
Furthermore, inflammation is a frequent cause of retinal degeneration [Citation26]. Ocular ganglion cells are injured, and their numbers are decreasing. The retina weakens and thins, the patient’s capacity to detect light is compromised, and the patient has varying degrees of vision loss [Citation27]. In addition to oxidative stress, inflammation, and various damaging processes, including as aberrant metabolism and neovascular development abnormalities, affect the diseased intraocular environment [Citation28].
Endothelial dysfunction is indeed a well-known pathogenic characteristic of DR. Multiple interacting variables, including as advanced glycosylation end products, pro-inflammatory cytokines, growth factors, and oxidative stress, promote endothelial injury. Furthermore, diabetics’ retinal microvasculopathy and neurodegeneration cause the blood-retinal barrier (BRB) to break down, resulting in edema in the retinal structures, acellular capillaries, and neo-vessel development [Citation1].
The current histology results were in line with the outcomes of prior studies that discovered a significant drop in INL and ONL thickness after 12 weeks of diabetic state. This decrease can be attributable to increased death of retinal neurons [Citation29,Citation30]. The appearance of empty spaces may be attributed to glial cells phagocytosing apoptotic retinal neurons [Citation31]. Furthermore, the reduction in the total number of ganglion cells coincided with a previous research [Citation32]. This can be explained by ganglion cell apoptosis, but to a smaller extent than in the other retinal layers [Citation33]. Furthermore, the inner retina’s blood vessel count increased, which can be explained by pathological neovascularization in advanced DR [Citation34].
The current histopathological results in terms of retinal thickness were consistent with the previous investigators [Citation35,Citation36] who observed thinner retinas, degraded cells (RPE, ONL, INL, and GCL), and dilated congested new blood vessels. This finding was explained as in diabetic retinopathy, mitochondrial dysfunction, endoplasmic reticulum stress, and consequent collapse of cellular homeostasis participate in retinal cell death [Citation37].
The three primary protein filaments that comprise the cytoskeleton are microtubules, intermediate filaments, and actin filaments (F-actin) [Citation38]. They are in charge of regulating cell structure, placing cellular organelles and conveying intracellular proteins. F-actin and MTs collaborate closely in both function and structural structure [Citation39]. It has previously been demonstrated that f-actin polymerization contributes to pericyte contraction in vitro [Citation40]. In the ongoing research, F-actin was shown to be mildly positive in PRL, INL, and GCL of the STZ group. This could be related to retinal cell degeneration, as DR is a vascular condition associated with endothelial dysfunction, which includes endothelial cell and pericyte loss, neovascularization, thickening of the capillary basement membrane and leaking in the BRB [Citation41].
A previous study also established retinal neuron loss as a key contributor in DR [Citation42]. In addition, during the preliminary phases of DR, neurodegeneration and immune-mediated inflammation impact retinal ganglionic cells [Citation43]. Pericytes serve as command and control centers for retinal vascular homeostasis, including the establishment of the BRB [Citation44]. In experimental DR, pericyte loss may result in microaneurysm development, which occurs before endothelial cell death [Citation45].
A prior study discovered that pericyte apoptosis is greater in diabetic retinal samples than in non-diabetic retinas [Citation46]. Several previous studies discovered that in human diabetic retinas, more neurons within retinae in ganglion cell layer die via apoptosis than in non-diabetic retinas [Citation47,Citation48].
Previous research has shown that endothelial cell death occurs in experimental DR [Citation49] and human diabetic retinas [Citation50]. Ganglions in diabetic people's retinal tissues have been discovered to demonstrate proapoptotic proteins Bax, Fas, and caspase-3, indicating that retina cells are susceptible to DR [Citation51]. Furthermore, DR is connected with the death of retinal neuronal cells [Citation52], retinal pigment epithelium malfunction [Citation53] and retinal thinning [Citation52], all of which contribute to DR.
Immunohistochemistry results demonstrated that antibodies against S-100 protein for perivascular glial cells and CD-57 antigen for Müller cells showed high positive expression in nerve fiber layer and ganglion cell layer. This finding aligned with an older study finding of enhanced immunoreactivity to S-100 protein, corresponding to perivascularly situated glial cells in diabetic patients’ retinas. Furthermore, the material invading the lumen of some retinal capillaries in vascular occlusion locations was immunoreactive to CD-57, indicating that this material is ingrowth of Müller cells of retina [Citation54].
S100B serves as a calcium-binding protein found primarily within the nervous system astrocytes. Its levels in biological fluids have been recognized as a valid sign of continuous brain injury, and more recently, accumulating evidence pointed to S100B as an injury-associated molecular pattern molecule that, at high quantities, initiates tissue responses to damage [Citation55].
The retina contains immune-like cells known as microglia, astrocytes, as well as Müller cells. Astrocytes along with Müller cells are involved in the establishment of the blood retinal barrier (BRB) [Citation56] whilst activated microglia can phagocytoze cellular debris, acting similarly to macrophages [Citation57]. Neuronal stress and cell death activate glial cells, which occurs early in DR. Acute gliosis is beneficial to the stressed retina because glia and microglia remove debris and cytotoxins, phagocytize apoptotic cells, and secrete neurotrophic factors. However, because activated glial cells emit inflammatory cytokines, cytotoxic chemicals, as well as vascular growth factors, chronic gliosis in the DR is harmful to the neurons and blood vessels of the retina, leading to microvascular malfunction and neurodegeneration [Citation27].
In the present experiment, metformin treatment improved retinal pathology moderately, but nanodrug therapy improved retinal pathology significantly in the STZ+ MTF-NE group. The present morphometric estimation of the area% of F-actin, CD57, and S100 positive cells demonstrated that their levels improved mostly to normal in control group following MTF, and MTF-NE treatment. Traditional treatments for retinal degeneration or injury include surgery and pharmaceuticals, such as eye elimination, anti-inflammatory medication, and immunosuppressive medications [Citation58].
Regarding the healing capacity of Metformin in the current study, MTF is extensively used as a therapy for diabetes mellitus of type-2 because to its favorable benefits, high safety, and low cost. MTF exerts its anti-diabetic effect by decreasing hepatic gluconeogenesis and increasing insulin sensitivity to regulate abnormally high blood glucose levels and reduce associated diabetic complications, as well as having strong anti-inflammatory, anti-oxidative, anti-apoptotic, and anti-cancer properties [Citation59].
In the current work, MTF therapy enhanced retinal thickness in DR rats compared to control rats. This result was in line with the result of a previous investigator, who found that MTF reduced diabetes-induced retinopathy in terms of gliosis and apoptosis, and that its mechanism may be linked to hyperglycemia reduction and oxidative stress suppression [Citation58].
A recent study reported four weeks of metformin therapy at a dosage of 500 mg/kg/day might partially correct diabetes alterations but not to a healthy level. As a result, the MTF is not completely effective [Citation11].
Because nanoparticles provide a number of advantages, including increased effectiveness in therapy, continued and improved drug absorption, decreased toxic effects, improved stability, and reduced drug breakdown, the MTF-nanoemulsion treatment produced better results in retinal abnormalities and immunohistochemical markers [Citation14].
Hassan et al. [Citation60] found that nano-metformin was more efficient in reducing inflammation, fibrosis, and apoptosis in diabetic nephropathy rats than normal metformin. In addition to its potential to reduce amyloid deposits, nano-metformin may contribute to improving nephropathy. This study supported our data in role of nano-metformin in improving retinopathy
According to Albeltagy et al. [Citation15], incorporating metformin into the oil phase of a nanoemulsion reduces blood sugar levels significantly. Additionally, Abbasian et al. [Citation7] found that nanoparticle assembly of metformin has a high drug release profile and enhances absorption in the gastrointestinal tract, especially at lower doses. Furthermore, nanoparticles had significantly larger glucose-lowering effects than pure metformin, which contradicted Kumar’s work [Citation61].
Conclusion
This study found that metformin nanoparticle therapy can ameliorate diabetic retinopathy at both the histology and immunohistochemical levels. It is advised that additional molecular and genetic research be conducted on nano-therapy of diabetes complications, particularly diabetic retinopathy, with metformin nanoparticles in order to understand the pathways and mechanisms that led to the positive results in this study.
Abbreviation
BRB: blood retinal barrier; DR: Diabetic retinopathy; F-actin: actin filaments; MTF: metformin; MTF-NE: metformin nanoemulsion; MTs: microtubules; PdI: polydispersity index; ROS: reactive oxygen species; SD: standard deviation; STZ: streptozotocin; VEGF: vascular endothelial growth factor;
Availability of data and materials
All datasets analyzed and described during the present study are available from the corresponding author upon reasonable request.
Author contribution
Sherif R. is the investigator who performed the animal experiments, data analyses and paper writing. B.N. and A.S. performed histopathological analyses, and discussion of results. M. A., D.S. & A.S. contributed to the completion of the experimental and laboratory analysis.
Ethics approval
The study has followed the guidelines of Institutional Animal Care and Use Committees at Helwan University (HU-IACUC/Z/SR0104–41).
Acknowledgments
The authors would like to express their gratitude to the Zoology Department at Faculty of Science, Helwan University in Cairo, Egypt.
Disclosure statement
No potential conflict of interest was reported by the author(s).
References
- Sorour OA, Mehta N, Baumal CR, et al. Morphological changes in intraretinal microvascular abnormalities after anti-VEGF therapy visualized on optical coherence tomography angiography. Eye Vis (Lond). 2020;7(1):29. doi: 10.1186/s40662-020-00195-2
- Cho NH, Shaw JE, Karuranga S, et al. IDF diabetes atlas: Global estimates of diabetes prevalence for 2017 and projections for 2045. Diabet Res Clin Pract. 2018;138:271–281. doi: 10.1016/j.diabres.2018.02.023
- Curtis TM, Gardiner TA, Stitt AW. Microvascular lesions of diabetic retinopathy: clues towards understanding pathogenesis? Eye (Lond). 2009;23(7):1496–1508. doi: 10.1038/eye.2009.108
- Jiang T, Chang Q, Cai J, et al. Protective effects of melatonin on retinal inflammation and oxidative stress in experimental diabetic retinopathy. Oxid Med Cell Longev. 2016;2016:3528274. doi: 10.1155/2016/3528274
- Luippold G, Bedenik J, Voigt A, et al. Short- and longterm glycemic control of streptozotocin-induced diabetic rats using different insulin preparations. PLOS ONE. 2016;11(6):e0156346. doi: 10.1371/journal.pone.0156346
- Titchenell PM, Antonetti DA. Using the past to inform the future: anti-VEGF therapy as a road map to develop novel therapies for diabetic retinopathy. Diabetes. 2013;62(6):1808–1815. doi: 10.2337/db12-1744
- Abbasian M, Bighlari P, Mahmoodzadeh F, et al. A de novo formulation of metformin using chitosan-based nanomicelles for potential diabetes therapy. J Appl Polym Sci. 2019;136(41):48037. doi: 10.1002/app.48037
- Bodmer M, Meier C, Krahenbuhl S, et al. Metformin, sulfonylureas, or other antidiabetes drugs and the risk of lactic acidosis or hypoglycemia: a nested case-control analysis. Diabetes Care. 2008;31(11):2086–2091. doi: 10.2337/dc08-1171
- Ilahi I, Asghar A, Ali S, et al. Beneficial effects of Pentanema vestitum Linn. whole plant on the glucose and other biochemical parameters of alloxan induced diabetic rabbits. ISRN Pharmacol. 2012;2012:478023. doi: 10.5402/2012/478023
- Hassan NH, Maern S, Saleh D. Does metformin protect against diabetic retinopathy in albino rats? An immunohistochemical study. Cytol & Histol Rep. 2019;2(02):110.
- Ibrahim IY, Ali FF, Abdel-Hakeem EA, et al. Pathophysiological mechanisms of type 2 diabetes mellitus and the effects of metformin treatment in adult male albino rats. Minia J Med Res. 2023;34(1):209–214. doi: 10.21608/mjmr.2023.184444.1271
- Freichels H, Danhier F, Préat V, et al. Fluorescent labeling of degradable poly(lactide-co-glycolide) for cellular nanoparticles tracking in living cells. Int J Artif Organs. 2011;34(2):152–160. doi: 10.5301/ijao.2011.6420
- Jia L. Nanoparticle formulation increases oral bioavailability of poorly soluble drugs: Approaches experimental evidences and theory. Curr Nanosci. 2005;1(3):237–243. doi: 10.2174/157341305774642939
- Liu KC, Yeo Y. Extracellular stability of nanoparticulate drug carriers. Arch Pharm Res. 2014;37(1):16–23. doi: 10.1007/s12272-013-0286-0
- Albeltagy RS, Hussein MM, Alazzouni AS, et al. Anti-diabetic effects of metformin nanoemulsion and cell-based therapy on the insulin signaling pathway (IRS1/AKT) and apoptotic related genes in type 2 diabetic rat model. Egypt Acad J Of Biol Sci C, Physiol And Mol Biol. 2021;13(1):159–172. doi: 10.21608/eajbsc.2021.178255
- Lin C-Y, Chen L-W. Comparison of fuel properties and emission characteristics of two- and three-phase emulsions prepared by ultrasonically vibrating and mechanically homogenizing emulsification methods. Fuel. 2008;87(10–11):2154–2161. doi: 10.1016/j.fuel.2007.12.017
- Rodrigues FVS, Diniz LS, Sousa RMG, et al. PREPARATION and CHARACTERIZATION of NANOEMULSION CONTAINING a NATURAL NAPHTHOQUINONE. Quím Nova. 2018;41:756–761. doi: 10.21577/0100-4042.20170247
- Abdel Aziz MT, Wassef MAA, Ahmed HH, et al. The role of bone marrow derived-mesenchymal stem cells in attenuation of kidney function in rats with diabetic nephropathy. Diabetol Metab Syndr. 2014;6(1):34. doi: 10.1186/1758-5996-6-34
- Bancroft JD, Layton C. The hematoxylins and eosin. Bancroft’s theory and practice of histological techniques. Elsevier; 2019. p. 126–138. doi: 10.1016/B978-0-7020-6864-5.00010-4
- Armitage P, Berry G, Matthews JNS. Statistical methods in medical research. Fourth ed. 2008. p. 760–783. doi: 10.1002/9780470773666.refs
- Chibber R, Ben-Mahmud BM, Chibber S, et al. Leukocytes in diabetic retinopathy. Curr Diabetes Rev. 2007;3:3–14. doi: 10.2174/157339907779802139
- Tang J, Kern TS. Inflammation in diabetic retinopathy. Prog Retin Eye Res. 2011;30:343–358. doi: 10.1016/j.preteyeres.2011.05.002
- Yu D-Y, Cringle SJ. Retinal degeneration and local oxygen metabolism. Exp Eye Res. 2005;80(6):745–751. doi: 10.1016/j.exer.2005.01.018
- Masuda T, Shimazawa M, Hara H. Retinal diseases associated with oxidative stress and the effects of a free radical scavenger (Edaravone). Oxid Med Cell Longev. 2017;2017:9208489. doi: 10.1155/2017/9208489
- Nishimura Y, Hara H, Kondo M, et al. Oxidative stress in retinal diseases. Oxid Med Cell Longev. 2017;2017:4076518. doi: 10.1155/2017/4076518
- Rathnasamy G, Foulds WS, Ling E-A, et al. Retinal microglia - a key player in healthy and diseased retina. Prog Neurobiol. 2019;173:18–40. doi: 10.1016/j.pneurobio.2018.05.006
- Cuenca N, Fernández-Sánchez L, Campello L, et al. Cellular responses following retinal injuries and therapeutic approaches for neurodegenerative diseases. Prog Retin Eye Res. 2014;43:17–75. doi: 10.1016/j.preteyeres.2014.07.001
- Campochiaro PA, Akhlaq A. Sustained suppression of VEGF for treatment of retinal/choroidal vascular diseases. Prog Retin Eye Res. 2021;83:100921. doi: 10.1016/j.preteyeres.2020.100921
- Martin PM, Roon P, Van Ells TK, et al. Death of retinal neurons in streptozotocin-induced diabetic mice. Invest Ophthalmol Vis Sci. 2004;45(9):3330–3336. doi: 10.1167/iovs.04-0247
- Sadek E, Abd-El Fattah L, El Maadawi Z, et al. Simvastatin ameliorates vascular endothelial growth factor overexpression in a rat model of diabetic retinopathy: a histological and immunohistochemical study. Kasr Al Ainy Med J. 2017;23(1):24. doi: 10.4103/2356-8097.207186
- Marc RE, Jones BW, Watt CB, et al. Extreme retinal remodeling triggered by light damage: implications for age related macular degeneration. Mol Vis. 2008;14:782–806.
- Foureaux G, Nogueira BS, Coutinho DCO, et al. Activation of endogenous angiotensin converting enzyme 2 prevents early injuries induced by hyperglycemia in rat retina. Braz J Med Biol Res. 2015;48(12):1109–1114. doi: 10.1590/1414-431X20154583
- Huang H, Gandhi JK, Zhong X, et al. TNFα is required for late brb breakdown in diabetic retinopathy, and its inhibition prevents leukostasis and protects vessels and neurons from apoptosis. Invest Ophthalmol Vis Sci. 2011;52(3):1336–1344. doi: 10.1167/iovs.10-5768
- Moran EP, Wang Z, Chen J, et al. Neurovascular cross talk in diabetic retinopathy: Pathophysiological roles and therapeutic implications. Am J Physiol Heart Circ Physiol. 2016;311(3):H738–749. doi: 10.1152/ajpheart.00005.2016
- El-Halim He A, Helal OK, Salem NE, et al. The possible therapeutic effect of mesenchymal stem cells and their exosomes on experimentally induced diabetic retinopathy in rats: Histological and immunohistochemical study. Egypt J Histol. 2020;43:390–411. doi: 10.21608/ejh.2019.18175.1185
- Yang Q-H, Zhang Y, Jiang J, et al. Protective effects of a novel drug RC28-E blocking both VEGF and FGF2 on early diabetic rat retina. Int J Ophthalmol. 2018;11(6):935–944. doi: 10.18240/ijo.2018.06.07
- Roy S, Trudeau K, Roy S, et al. Mitochondrial dysfunction and endoplasmic reticulum stress in diabetic retinopathy: mechanistic insights into high glucose-induced retinal cell death. Curr Clin Pharmacol. 2013;8(4):278–284. doi: 10.2174/1574884711308040003
- Darnell JE, Lodish HF, Baltimore D. Molecular cell biology. 2nd ed. (NY): Scientific American Books : Distributed by W.H. Freeman; 1990.
- Zhou F-Q, Waterman-Storer CM, Cohan CS. Focal loss of actin bundles causes microtubule redistribution and growth cone turning. J Cell Bio. 2002;157(5):839–849. doi: 10.1083/jcb.200112014
- Lee S, Zeiger A, Maloney JM, et al. Pericyte actomyosin-mediated contraction at the cell–material interface can modulate the microvascular niche. J Phys Condens Matter. 2010;22(19):194115. doi: 10.1088/0953-8984/22/19/194115
- Hammes H-P, Feng Y, Pfister F, et al. Diabetic retinopathy: targeting vasoregression. Diabetes. 2011;60(1):9–16. doi: 10.2337/db10-0454
- Cai X, McGinnis JF. Diabetic retinopathy: Animal models, therapies, and perspectives. J Diabetes Res. 2016;2016:3789217. doi: 10.1155/2016/3789217
- Asnaghi V, Gerhardinger C, Hoehn T, et al. A role for the polyol pathway in the early neuroretinal apoptosis and glial changes induced by diabetes in the rat. Diabetes. 2003;52(2):506–511. doi: 10.2337/diabetes.52.2.506
- Park DY, Lee J, Kim J, et al. Plastic roles of pericytes in the blood–retinal barrier. Nat Commun. 2017;8(1):15296. doi: 10.1038/ncomms15296
- Pfister F, Feng Y, Vom Hagen F, et al. Pericyte migration: a novel mechanism of pericyte loss in experimental diabetic retinopathy. Diabetes. 2008;57(9):2495–2502. doi: 10.2337/db08-0325
- Romeo G, Liu W-H, Asnaghi V, et al. Activation of nuclear factor-κb induced by diabetes and high glucose regulates a proapoptotic program in retinal pericytes. Diabetes. 2002;51(7):2241–2248. doi: 10.2337/diabetes.51.7.2241
- Oshitari T, Yamamoto S, Hata N, et al. Mitochondria- and caspase-dependent cell death pathway involved in neuronal degeneration in diabetic retinopathy. Br J Ophthalmol. 2008;92:552–556. doi: 10.1136/bjo.2007.132308
- Oshitari T, Yamamoto S, Roy S. Increased expression of c-Fos, c-Jun and c-Jun N-terminal kinase associated with neuronal cell death in retinas of diabetic patients. Curr Eye Res. 2014;39(5):527–531. doi: 10.3109/02713683.2013.833248
- Joussen AM, Doehmen S, Le ML, et al. TNF-alpha mediated apoptosis plays an important role in the development of early diabetic retinopathy and long-term histopathological alterations. Mol Vis. 2009;15:1418–1428.
- Al-Shabrawey M, Ahmad S, Megyerdi S, et al. Caspase-14: a novel caspase in the retina with a potential role in diabetic retinopathy. Mol Vis. 2012;18:1895–1906.
- Abu-El-Asrar AM, Dralands L, Missotten L, et al. Expression of apoptosis markers in the retinas of human subjects with diabetes. Invest Ophthalmol Vis Sci. 2004;45(8):2760–2766. doi: 10.1167/iovs.03-1392
- Barber AJ. Diabetic retinopathy: recent advances towards understanding neurodegeneration and vision loss. Sci China Life Sci. 2015;58(6):541–549. doi: 10.1007/s11427-015-4856-x
- Wang HJ, Jin YX, Shen W, et al. Low dose streptozotocin (STZ) combined with high energy intake can effectively induce type 2 diabetes through altering the related gene expression. Asia Pac J Clin Nutr. 2007;16 Suppl 1:412–417.
- Bek T. Immunohistochemical characterization of retinal glial cell changes in areas of vascular occlusion secondary to diabetic retinopathy. Acta Ophthalmol Scand. 1997;75:388–392. doi: 10.1111/j.1600-0420.1997.tb00395.x
- Michetti F, Clementi ME, Di Liddo R, et al. The S100Bprotein: a multifaceted pathogenic factor more than a biomarker. Int J Mol Sci. 2023;24(11):9605. doi: 10.3390/ijms24119605
- Vecino E, Rodriguez FD, Ruzafa N, et al. Glia-neuron interactions in the mammalian retina. Prog Retin Eye Res. 2016;51:1–40. doi: 10.1016/j.preteyeres.2015.06.003
- Jin N, Gao L, Fan X, et al. Friend or Foe? Resident microglia vs bone marrow-derived microglia and their roles in the retinal degeneration. Mol Neurobiol. 2017;54(6):4094–4112. doi: 10.1007/s12035-016-9960-9
- Kaur G, Singh NK. The role of inflammation in retinal neurodegeneration and degenerative diseases. Int J Mol Sci. 2021;23:386. doi: 10.3390/ijms23010386
- LaMoia TE, Shulman GI. Cellular and molecular mechanisms of metformin action. Endocr Rev. 2021;42(1):77–96. doi: 10.1210/endrev/bnaa023
- Hassan BN, Said AA, Fathalla AS. Ameliorative effect of metformin nano emulsion against induced diabetic nephropathy in rat model: microanatomy study. Adv In Basic And Appl Sci. 2023;1(1):55–62. doi: 10.21608/abas.2023.195731.1010
- Kumar S, Bhanjana G, Verma RK, et al. Metformin-loaded alginate nanoparticles as an effective antidiabetic agent for controlled drug release. J Pharm Pharmacol. 2017;69(2):143–150. doi: 10.1111/jphp.12672