ABSTRACT
To highlight how gene drives could be useful for control of agricultural insect pests, we selected species that are pests of animals (New World screwworm), plants (spotted wing Drosophila, diamondback moth, Bemisia tabaci whitefly), or stored grains (red flour beetle). With the exception of whitefly, routine methods for delivering DNA to the germline and selecting for genetically modified insects have been developed. The traditional approach in agriculture has been to suppress insect pest populations using insecticides and other farming practices. Similarly, we suggest the main use of gene drives in agriculture will be for population suppression through targeting essential genes. We provide examples of gene drives that target specific genes including female-essential genes. Further, we discuss issues related to containment in the laboratory and eventual field testing of strains harboring a Cas9-mediated gene drive system.
Introduction
Insect pests of crops, livestock, and agricultural products have long been a threat to food production. Global losses due to arthropod crop pests (mostly insects and mites) are estimated to total $470 billion annually (Culliney Citation2014). Similarly, insects are major economic pests of livestock. For example, in the United States the horn fly is estimated to cause losses of $1 billion annually for the cattle industry (Kunz et al. Citation1991). Historically, efforts to control these pests and reduce losses have relied on extensive physical labor, changes in agricultural practices or the application of chemicals that kill the offending insects. However, chemical controls are fraught with their own challenges. Some of the most potent pesticides were later discovered to hurt benign and beneficial insects and vertebrates. Meanwhile, target pests develop resistance over time, requiring ever-increasing doses of poison until the pesticide loses all effectiveness. Thus, new pesticides must be continuously developed which effectively control the target insect, while limiting the impact on non-target species (Casida and Quistad Citation1998).
Using knowledge of pest genetics to control pests, either by reducing pest populations or limiting their ability to do damage, is a particularly attractive idea that has historically been limited by technological feasibility. The sterile insect technique (SIT), which involves radiation-induced chromosome breakage, has been successfully used in a small number of species to produce adult males that can be released in the field to mate with wild females that then produce inviable embryos (Klassen and Curtis Citation2005). To substantially reduce a pest population, this technique requires the continual release of large numbers of irradiated males. Thus SIT is particularly effective if the pest population is low. A number of more complex genetic approaches for manipulating pests were described and explored theoretically and empirically, starting in the 1940s (Klassen and Curtis Citation2005). For human disease vectors, an alternative approach to eradication is to replace the population with insects that cannot transmit disease as they carry a gene refractory to transmitting a human pathogen. Curtis suggested the concept of linking the refractory gene to a genetic element (translocation) that could drive the desired gene into a wild population (Curtis Citation1968). Genetic drive elements with super-Mendelian inheritance (more than 50% of the offspring inherit the genetic element) abound in nature, ranging from sequences capable of replicating themselves in a genome (e.g. transposons, aka ‘jumping genes’) to systems that kill any offspring that fail to inherit the element (e.g. Medea; Beeman, Friesen, and Denell Citation1992). However, adapting natural gene drives has proven to be technically difficult as the mechanisms are not generally well understood or easily adapted. Nevertheless, artificial drives based on the principles of natural elements have been developed such as synthetic Medea (Chen et al. Citation2007). Artificial drives based on the CRISPR/Cas9 gene-editing system seem the simplest and most effective to build. Briefly, Cas9 is an RNA-guided nuclease discovered in bacteria to function as part of an adaptive immune system (Bhaya, Davison, and Barrangou Citation2011). Short RNA sequences guide Cas9 to a target, where Cas9 introduces a double-stranded break. Given appropriate guides, Cas9 is also capable of introducing double-stranded breaks in eukaryotic genomes, including those of insects and vertebrates, where the breaks can be repaired randomly (non-homologous end-joining, NHEJ) or based off a template (homology-directed repair) (Jinek et al. Citation2012; Mali, Esvelt, and Church Citation2013). The latter mechanism can be exploited to introduce the coding sequence for Cas9 and guides into the genomic site targeted by those guides. This results in a self-replicating insertion that could continuously mutate a target gene every generation or carry an introduced gene into the population (Esvelt et al. Citation2014). More details for the design of Cas9 gene drives are discussed elsewhere in this volume (Min, Smidler, and Esvelt Citation2017).The purpose of this paper is to discuss the potential use of Cas9-mediated gene drive systems for control of several agricultural insect pests.
Gene drive systems and agricultural insect pests
One of the simplest goals for gene-based pest control would be population suppression, i.e. decreasing the number of insects in an area, thereby reducing the damage done to crops or livestock. One way a Cas9-based gene drive could be used for population suppression is to target a gene that is essential for survival in the field but unimportant in a rearing facility (e.g. a gene essential for vision). Alternatively, a Cas9-based gene drive could be placed within a gene necessary for female development, survival, or fecundity. In theory, releasing a few insects carrying a very efficient drive could be sufficient to suppress a pest population after many generations. In practice, growers would likely desire a faster response, which could be achieved by releasing more insects carrying the gene drive. For example, a ratio of 1:10, modified:wild-type insects could lead to pest suppression in 10–20 generations. However, rearing a large number of insects carrying a gene drive that targets an essential gene for females would be difficult in practice, since the drive would be expected to suppress the captive population. The simplest way to overcome this limitation would be to manually cross the males of the drive strain back to wild type (non-drive) virgin female insects each generation, but this would also be very labor intensive. It may be possible to obtain sufficient virgin females through mechanical sorting of males and females at an early stage of development. Sorting could be based on differences in size between the sexes (Carvalho et al. Citation2014) or sex-specific expression of a fluorescent marker gene (Catteruccia, Benton, and Crisanti Citation2005).
We suggest that an attractive alternative is to control Cas9 activity such that it can be suppressed in the insect rearing facility, but would be active in the field. There are a variety of conditional expression systems that can be used to switch Cas9 production on or off. The most effective systems involve the use of a chemical added to the insect food which blocks gene activation. For example, the tetracycline transactivator (tTA)/tetO conditional expression system (Gossen and Bujard Citation1992), which uses tetracycline in the insect diet to suppress gene expression, is currently used for suppression of female-lethal genes in engineered strains designed for genetic control programs (Koukidou and Alphey Citation2014; Scott Citation2014), as will be discussed below. The protein, tTA, binds a very specific genetic sequence, tetO, and can activate genes found down stream of tetO sequences. However, in the presence of tetracycline, tTA does not bind to DNA. An example of the design of a conditional Cas9 gene drive system is shown in .
Figure 1. A conditional Cas9-mediated gene drive. The tetracycline transactivator (tTA) is expressed in the germline using the vasa gene promoter. In the absence of tetracycline in the diet, tTA will bind to tetO and activate expression of Cas9. Expression of a gRNA complementary to an essential gene is controlled using a U6 gene promoter. Following Cas9 digestion of the essential gene, homology-directed repair will lead to insertion of the multi-gene cassette bracketed by left (LHA) and right (RHA) homology arms. A GFP marker gene would facilitate initial identification of genetically modified insects. Insulator elements (I) prevent tTA bound to tetO from enhancing its expression from the vasa gene promoter, which could reduce fitness. Additional containment measures should be used for drive evaluation such as physical barriers and splitting the drive by not including the vasa-Cas9 or U6-gRNA gene in the construct shown.
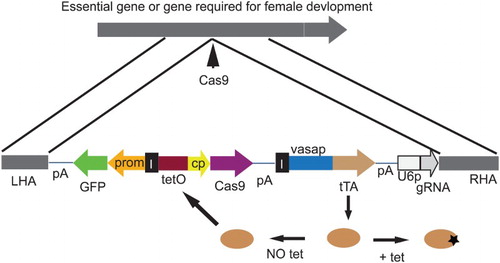
It should be noted that conditional expression systems, like tTA/tetO, would not prevent accidental release of modified insects (Akbari et al. Citation2015), a particular concern for gene drives, since the drive would be active when the insects feed on a diet that lacks tetracycline (i.e. most food options found in the wild). However, if tTA was replaced with reverse tTA (rtTA) (Urlinger et al. Citation2000), the system would only drive on diet that contains tetracycline, as rtTA will only bind DNA in the presence of tetracycline. This could provide a means for containment while evaluating gene drive systems, in addition to physical barriers and other strategies (e.g. split drive with Cas9 and gRNA components separated) (Akbari et al. Citation2015). In addition to the proposed containment strategies for evaluation of gene drives, we also support the parallel development of reversal drives with recoded target genes (Esvelt et al. Citation2014). As discussed elsewhere in this volume (Burt et al.), there are several issues that would need to be addressed through studies in containment (e.g. influence of genetic background) prior to consideration of a field release. Further, we have focused only on using Cas9-mediated gene drives for population suppression. They could also be used to drive anti-pathogen genes into an insect population that carries diseases. While there may be cases where this would be more useful than population suppression, primary damage from pest insects is typically the greater concern for agriculture. The purpose of this paper is to examine the potential for using gene drives for population suppression of selected agricultural pests.
The new world screwworm: a devastating obligate parasite of livestock
The New World screwworm fly, Cochliomyia hominivorax is a serious pest of warm-blooded animals (Krafsur Citation1998; Alexander Citation2006). Females lay their eggs in open wounds or a natural orifice. The hatched larvae then feed on the animal’s living tissue. Animals with severe screwworm infestations may die if untreated. While most cases are less severe, they can still have an economic impact (Vargas-Teran, Hofmann, and Tweddle Citation2005), as the animal suffers weight loss, and carcasses and hides are damaged. Screwworm populations are limited in their range by cold seasonal temperatures: the insect cannot survive freezing temperatures and cannot overwinter successfully under temperate conditions. The pre-eradication range was between 35 and −35 latitudes in the Western Hemisphere.
Edward Knipling realized that if large numbers of sterile males could repeatedly be released into wild populations, it would eventually eliminate population reproduction and lead to eradication (Knipling Citation1960, Citation1955). This genetic control method is now generally referred to as the SIT. The program initiated by Knipling and his colleague, Raymond Bushland, began with releases of sterilized insects in Florida in the late 1950s. Subsequently, the SIT approach was used to eradicate screwworm from all of the USA (Mastrangelo and Welch Citation2012). However, Texas farmers faced an ongoing threat of invasion of screwworm from Mexico. To alleviate this threat, SIT was used to eradicate the fly from that country in a joint program with the Mexican government. Subsequently, the program was extended to eradicate screwworm from all of Central America (Vargas-Teran, Hofmann, and Tweddle Citation2005). Currently, to prevent re-infestation from South America, sterilized flies are being released in a ‘buffer zone’ in Eastern Panama and along the border with Colombia (Scott et al. Citation2017). The screwworm mass rearing facility is in Pacora, Panama, and is run by the U.S.-Panamanian Commission for the Eradication and Prevention of Screwworms (Comisión Panamá–Estados Unidos para la Erradicación y Prevención del Gusano Barrenador del Ganado or COPEG). About 15 million flies are reared each week. The annual producer benefit from eradication of screwworm is estimated to be $1.3 billion annually (Vargas-Teran, Hofmann, and Tweddle Citation2005). Due to the success of the screwworm SIT program, SIT has since been used for control of several species of tropical fruit flies (e.g. Mediterranean fruit fly), olive fly, and some Lepidopteran species (e.g. codling moth) (Klassen and Curtis Citation2005). So why was the screwworm SIT program so successful? Are there any lessons for future control programs based on Cas9 gene drive systems?
SIT is often said to be a ‘numbers game’ because success depends on the released sterile males outnumbering the wild-type males, preferably by at least 10:1 (Klassen and Curtis Citation2005). Fortunately, the screwworm fly is naturally present at low densities (Knipling Citation1955). In addition, economical mass rearing methods were developed (Melvin and Bushland Citation1936; Chaudhury and Skoda Citation2007), sterilization by radiation had only a small negative effect on male fitness (Crystal Citation1979), and there were no significant mating barriers across the eradication zone (Lachance et al. Citation1982). More recently, efficient methods were developed for distributing chilled sterile flies by air using modern GPS-guided navigation (Tween and Rendon Citation2007; Scott et al. Citation2017). Other factors that contributed to the success of the program that we think are also applicable to future gene drive programs include:
The technology was never seen as a ‘silver bullet’ that by itself would eradicate the pest population. Within the eradication zone a large monitoring network of veterinarians and others was established, and any infested animals were identified and promptly treated (Mastrangelo and Welch Citation2012).
As the eradication zone spanned several countries, agreements between neighboring countries were essential, as insects will cross national boundaries (Wyss Citation2000).
The eradication program was effectively managed by a team that did not have other responsibilities.
From the beginning, the screwworm program sought to engage the public and address any concerns regarding the release of radiation-sterilized flies.
Given the success of using SIT to eradicate screwworm, should we consider developing gene drive systems for agricultural pest population suppression? Screwworm remains a problem throughout most of tropical South America and on some Caribbean islands, notably Cuba and Jamaica (Scott et al. Citation2017). It may not be necessary to use a gene drive system for eradication and/or suppression of populations on islands and in regions in South America west of the Andes. In these locations, a more efficient SIT approach could be successful. The efficiency of SIT can be increased several fold by releasing only sterile males (Rendon et al. Citation2004). However, to date this has not been possible in the screwworm program. Recently, the corresponding author’s laboratory, along with USDA-ARS collaborators, have succeeded in creating transgenic ‘male-only’ screwworm strains that carry a conditional female-lethal gene that is suppressed by addition of tetracycline to the larval and adult diets (Concha et al. Citation2016). The best of these strains are now being evaluated under semi mass rearing conditions. Field trials of sterilized male flies have also been planned, but will require regulatory approval.
An efficient SIT program may not be able to eradicate or suppress screwworm populations east of the Andes, which presents a considerable geographic area with ideal habitat for screwworm. With no appreciable geographic barriers, there would be little to stop wild-type flies from invading an area being targeted by aerial releases of sterile screwworm flies. In contrast, the eradication of screwworm from North and Central America benefited from a narrowing of the eradication zone as the program moved progressively southward from Texas to Panama. In South America, a Cas9 gene drive might prove more useful; flies carrying an efficient gene drive system may only have to be released once throughout the region to potentially serve as an effective means for population suppression. Consequently, prior to release, approval should be sought from all countries that have endemic screwworm flies, regardless of whether they see actual releases, since the drive effect will spread beyond the original release zones over the course of multiple generations. In contrast, for the Screwworm Eradication Program, it was only necessary to obtain agreements with countries over which sterile flies were to be released (Wyss Citation2000).
What gene should be targeted for a Cas9 gene drive in screwworm? Modeling suggests that it would be advantageous to target genes that are essential for females but not males, or required for germ-cell development or reproduction in one sex (Burt Citation2003; Deredec, Burt, and Godfray Citation2008). One possibility would be to target the transformer (tra) gene, which is essential for female development in screwworm, as in most Diptera (Li et al. Citation2013). An unknown Y-linked male-determining gene switches off tra in males (). In females, tra switches the doublesex and fruitless genes into their ‘female-mode’, which leads to female development and behavior (Scott, Pimsler, and Tarone Citation2014). In the absence of tra activity, XX females develop as phenotypic males. We don’t know if XX males are fertile, but in the Australian sheep blowfly, a close relative of screwworm, the Y chromosome does not appear to encode any factors essential for male fertility (Concha and Scott Citation2009). Thus it is possible that transformed XX screwworm males will be fertile. If so and the XX males are competitive, a gene drive that would transform all females to males could be very effective for suppression of the insect pest population. One potential problem with this approach is that tra may play an essential role in the female germline. If so, it would be desirable to limit Cas9 expression, and thus gene drive, to the male germline, assuming a suitable gene promoter can be found.
Figure 2. A comparison of the genetic mechanisms that determine sex in Drosophila melanogaster, Lucilia cuprina and Tribolium casteneum. In female D. melanogaster embryos, a high dose of X signal elements (XSE) leads to activation of the master gene Sex lethal (Sxl). Sxl both autoregulates its expression and switches transformer (tra) RNA splicing into the female mode. Only the female form of tra mRNA codes for functional protein. TRA combines with TRA-2 to switch doublesex (dsx) and fruitless (fru) into the female modes of splicing. DSX and FRU control the expression of many genes that influence sexual development and behavior. The bottom of the regulatory hierarchy in L. cuprina appears to be the same as Drosophila. However, it is tra RNA splicing that is autoregulatory in females. The presence of the Y-linked M factor inhibits the female-mode of tra RNA splicing in male embryos. As in Drosophila, only the female form of tra mRNA codes for functional protein. In female embryos, maternal TRA likely contributes to the initiation of the female mode of tra splicing. The sex determination regulatory mechanisms appear to be identical in L. cuprina and C. hominivorax. Remarkably, the genetic mechanisms that determine sex in the red flour beetle, T. casteneum, appear to be very similar to the L. cuprina regulatory hierarchy. Adapted from Scott, Pimsler, and Tarone (Citation2014) and Shukla and Palli (Citation2013).
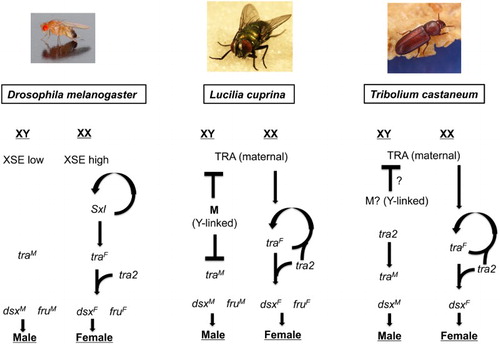
What would be the ecological impact of screwworm eradication from the Western hemisphere? Screwworm is an ectoparasite of warm-blooded animals, including humans. The eradication of screwworm from Texas correlated with an increase in white-tailed deer populations. However, other factors such as the absence of large predators (Wolverton, Kennedy, and Cornelius Citation2007) also likely contributed to the increase in deer populations in Texas, as well as across North America (VerCauteren Citation2003). There is insufficient literature regarding the ecological impact of eradication of the screwworm, which likely reflects that researchers have placed the primary focus of their limited resources on the interaction of the pest with livestock rather than with native hosts. Nevertheless, there is an opportunity to carry out ecological studies on screwworm and its hosts in the large Caribbean islands and/or South America before commencing a screwworm eradication/suppression program. Further, the screwworm experience highlights the need for more basic ecological studies for other pest insects before and after a population suppression program.
Lastly, there is a potential for resistance to develop to a Cas9-based gene drive system (Esvelt et al. Citation2014; Unckless, Clark, and Messer Citation2017). For example, resistance could arise due to repair errors or rare alleles with nucleotide polymorphisms in the PAM sequence. Consequently, it may be advantageous to follow a gene drive release with a male-only SIT, if the gene drive is effective at significantly reducing the population in a few generations. SIT is particularly effective if the population size of the pest species is low (Klassen and Curtis Citation2005). Thus the gene drive could provide a means for suppressing pest populations to the low levels at which SIT would be effective and economical. Further, SIT could eradicate the pest before resistance to the drive mechanism leads to a rebound in the population.
Spotted wing Drosophila: an invasive pest of soft-skinned fruits
Drosophila suzukii (commonly called spotted-wing Drosophila) larvae are capable of infesting a wide range of host fruit but appear to be most significant pests in stone fruits (peach, cherry, and plum) and berries (raspberries, blackberries, blueberries, and strawberries) (Lee et al., “In Focus,” Citation2011; Asplen et al. Citation2015). Unlike most other Drosophila species, female D. suzukii use their highly developed serrated ovipositor to pierce the skin of soft fruits and lay their eggs inside the fruit (Lee et al., “The Susceptibility of Small Fruits,” Citation2011). D. suzukii has a short generation time and multiple generations per year (Tochen et al. Citation2014). In contrast, the larger tephritid fruit flies (Rhagoletis sp.) native to North America and Europe have only one generation a year. D. suzukii is endemic in Asia but in 2008 the fly was found in California and Europe. Since then, D. suzukii has spread rapidly and is now found in temperate regions in North America and Europe (Cini et al. Citation2014). In the USA, any fruit that contain developing D. suzukii larvae can cause an entire shipment to be rejected. Growers are currently using broad-spectrum insecticides to protect fruit from damage caused by D. suzukii. Growers in North Carolina are using more insecticide to manage this invasive fruit fly, but the effectiveness of these treatments is weather dependent (Diepenbrock et al. Citation2016; Diepenbrock, Hardin, and Burrack Citation2017). It is also anticipated that D. suzukii will develop resistance to some of the more commonly used insecticides. Non-chemical means for controlling D. suzukii are needed.
Since several tropical fruit flies (e.g. the Mediterranean fruit fly) have been successfully controlled using SIT (Enkerlin Citation2005), the corresponding author’s lab and others are working on developing transgenic male-only strains. As discussed above for screwworm, these strains could be used for an efficient SIT program. Further, if the strains carry dominant female-lethal genes, it would not be necessary to sterilize the males before release. This is because all of the female offspring of the released males will die and the male offspring will pass on the dominant female-lethal gene. However, since any fruit containing a single D. suzukii maggot (wild type or transgenic) would be rejected, it is unclear if this approach would be acceptable to growers. Assuming a transgenic strain can be made with the desired properties and is approved by regulators, would an efficient SIT program be successful and economical for controlling D. suzukii? We are not aware of accurate measurements of D. suzukii population sizes, but trapping data suggest populations can be high, particularly in the warmer months. Consequently, a genetic control program would most likely be attempted early in the growing season when populations appear to be relatively low and in conjunction with intensive application of conventional methods for controlling D. suzukii.
As discussed above for screwworm, a Cas9-mediated gene drive targeting an essential gene could provide an effective means for population suppression. A high-quality reference genome sequence for D. suzukii is available (Chiu et al. Citation2013), which will facilitate gRNA design. Germ-line transformation of D. suzukii is relatively straightforward using procedures developed for D. melanogaster, a close relative that is a widely studied model genetic organism. Further, the engineering should be easier than for screwworm, as genetic tools developed for manipulating D. melanogaster should function in D. suzukii. Indeed, we have found that the D. melanogaster vasa and U6:3 gene promoters can be used to drive expression of Cas9 and gRNAs respectively in D. suzukii embryos (Li and Scott Citation2016). We have shown that Cas9 can be used to make loss of function mutations in the D. suzukii white and Sex lethal genes. The latter would appear to be a good target for a gene drive system since Sex lethal is essential for female Drosophila but not male. However, in D. melanogaster Sxl is required for female germ-cell identity (Schupbach Citation1985). If drive is very efficient, females would all be sterile. This would require that drive occur in the male germline. However, since the Sxl gene is on the X chromosome, if males carrying the mutant Sxl gene with the Cas9 gene cassette are mated with wild-type females, all of the male offspring will inherit a wild-type X chromosome. Thus the gene drive could not occur. An alternative would be to target the D. suzukii tra gene, which is essential for female development but not required in the female germline (Schupbach Citation1982) and is not on the X chromosome. The ecological consequences of D. suzukii suppression in North America and Europe are unknown. However, since it is a relatively recent invader from Asia, the ecological impacts are not be predicted to be significant.
In areas where D. suzukii is a recent invader and localized, such as on an island or around a port/airport, it may be reasonable to deploy a gene drive strategy aimed at population eradication rather than population suppression. For recent incursions, populations may be sufficiently localized and genetically homogeneous to achieve an eradication outcome. As for any biological control project, species specificity of the gene drive construct would need to be ensured. As discussed above, the gene drive would target a gene essential for female development or viability. Thus, the drive should be self-limiting. However, given the invasive nature of D. suzukii, additional measures should be taken to ensure containment of the drive and eradication of the species only in the non-native range. For example, both human-assisted and natural movement must be considered (Webber, Raghu, and Edwards Citation2015).
Diamondback moth
The diamondback moth (DBM), Plutella xylostella, feeds on plants in the mustard family (Brassicaceae). These plants include cabbage and an array of vegetable crops related to it (e.g. broccoli, collards, cauliflower, Brussels sprouts). Beyond vegetables, this moth also feeds on canola and wild mustards. The global economic cost of the DBM is estimated at between $4 and $5 billion, annually (Zalucki et al. Citation2012).
The DBM cannot overwinter in cold climates but has continuous generations in more tropical areas. Because each generation can be two-weeks to a month and each female can lay a total of approximately 150 eggs, the caterpillar densities on a crop can increase rapidly. Although the DBM has many biological control agents, the typical response to an infestation is application of synthetic insecticides, often applied more than once a week (Grzywacz et al. Citation2010). The DBM is notorious for evolving resistance to synthetic insecticides, with loss of control often emerging within a few years of commercialization of a new class of insecticide (Wang and Wu Citation2012). Although DBM cannot withstand cold winters, it is still a major, if sporadic, pest in temperate geographic areas because of its ability to migrate hundreds of miles, and in some cases over a thousand miles (Chapman et al. Citation2002; Fu et al. Citation2014). Broccoli has been genetically engineered to produce toxins from Bacillus thuringiensis (Bt toxins) that are effective against many DBM populations, but commercialization does not appear to be likely in the near future (Grzywacz et al. Citation2010). It is also important to note that the DBM was the first insect found to have resistance to Bt toxins. This occurred based on overuse of B. thuringiensis bacterial spores as a pesticide in Hawaii (Tabashnik Citation1994).
The problem of crop losses due to DBM, as well as concerns about human health and environmental impacts of insecticide use have persisted for over four decades (Talekar and Shelton Citation1993; Furlong, Wright, and Dosdall Citation2013). This made the concept of using area-wide suppression based on SIT (Knipling Citation1955) very appealing for this pest. Sutrisno and Hoedaya (Citation1993) reported on early efforts at inducing sterility using gamma radiation of DBM pupae. They found a dose of radiation that could cause substantial sterility in the moths and the offspring of treated moths. They tested the efficacy of this treatment on moth populations, initially using small cages in the laboratory. After success at this level of testing, they moved on to field cages, and then to open field plots. With replicated single 9:1 releases of irradiated to normal males and females they reduced field cage populations by approximately 50% after one generation. In the field experiment the release ratio was 14:1 and the reduction was about 40%. The second generation moths also showed some sterility and in 9:1 releases caused about 20% population reduction. These results were somewhat encouraging. Furlong, Wright, and Dosdall (Citation2013) indicate that there were no successful larger scale tests of this SIT approach with DBM, but it is difficult to discern how much effort was put into follow-up testing because of a lack of available research articles.
With the advent of insect genetic engineering, the British company, Oxitec, developed a strain of DBM in which the female larvae died if they did not have tetracycline in their food (Jin et al. Citation2013). Release of such a strain should be an improvement over use of irradiated moths, because only males would be released and heterozygous male offspring that carry one copy of the transgene would transmit the female-killing element to half of the next generation of offspring (Schliekelman and Gould Citation2000; Thomas et al. Citation2000). In a laboratory experiment, these moths had a fitness of about one half that of moths from a normal laboratory strain (Harvey-Samuel et al. Citation2014). This decreased fitness is substantial but not as great as expected due to radiation.
Recently, Oxitec and collaborators at Cornell University petitioned the USDA for a permit for a field cage and open field plot test of this female-lethal strain of diamondback moth. The petition was examined and approved based on an environmental assessment (Accessed 18 September 2017 at https://www.aphis.usda.gov/brs/aphisdocs/13_297102r_fonsi.pdf). The experiments themselves were delayed by Cornell University, but field cage experiments were conducted in 2015 (A. Shelton Personal Communication). In 2016 the permit for release was withdrawn by USDA because the original permitting process was flawed (Accessed 18 September 2017 at https://www.aphis.usda.gov/aphis/ourfocus/biotechnology/sa_environmental_documents/sa_environmental_assessments/diamondback-moth-permit-withdrawal). The following year, the USDA released the final environmental assessment with a finding of no significant impact (FONSI) (Accessed 23 September 2017 at https://www.aphis.usda.gov/aphis/ourfocus/biotechnology/brs-news-and-information/final_diamondback_moth). With an approved permit, open field tests with the transgenic DBM strain were planned for later in 2017 (Accessed 23 September 2017 at https://shelton.entomology.cornell.edu/diamondbackmoth/diamondback-moth-project-at-cornell-university-faq/).
One of the arguments in favor of the field test was that it would be conducted in upstate New York where the moths could not overwinter and so were expected to be biologically contained (APHIS Citation2014). The DBM migrates from the Southern USA to the Northern USA and to Canada in the summer, but there are no data indicating that there is return migration (Furlong, Wright, and Dosdall Citation2013; APHIS Citation2014). Because some data indicate that once in a field with host plants the DBM moves very little (Shirai and Nakamura Citation1994; Mo et al. Citation2003), the argument was made that released moths would not infest neighboring fields (APHIS Citation2014).
If the assumptions in the USDA APHIS environmental assessment hold, it is very possible that releases of this moth strain in the northern summer range of the DBM could suppress local population growth. A question remains of whether this approach will be appropriate for DBM in areas where they can breed all year or overwinter successfully. While there appears to be limited movement of moths when they are in suitable habitats, they are known for long distance movement at other times (Chapman et al. Citation2002; Fu et al. Citation2014), so local releases of the engineered strain could lower numbers temporarily, but this would be expected to have limited value if there was re-infestation from other areas. Furthermore, in the northern areas, the population size in the early summer is very low, making small releases of the engineered strain feasible, but in tropical and sub-tropical areas, the population size tends to remain high, making suppression difficult.
Suppression of DBM with a female-killing strain, even in areas where feasible, would require repeated release of large numbers of moths. This might be less expensive than use of insecticides when resistance to all available insecticides was a problem, but it would require community-level coordination in order to be most effective. Given that resource-poor farmers in tropical and sub-tropical areas are having the greatest problem suppressing DBM, a more efficient, cheaper means of control without associated environmental or health problems would be useful. This is where the use of a gene drive system could be of special interest.
As noted above, the DBM females lay approximately 150 eggs in their lifetimes. This suggests that they have the capacity to increase in density by about 75-fold per generation, given a 1:1 sex ratio and ideal conditions for survival. The actual rate of increase is substantially lower than that, but field experiments have found that DBM populations can triple in a 6-week period (e.g. Riggin-Bucci and Gould Citation1997). Burt (Citation2003) has shown theoretically that a meiosis-based, gene-drive system with 90% efficiency could reduce survival by 80%. If the maximal rate of increase of the drive in a DBM field population was 5-fold per generation, then such a gene drive could be effective. Burt (Citation2003) and others (Deredec, Burt, and Godfray Citation2008) outline additional gene drive systems that could be even more efficient and would be theoretically predicted to limit even tropical populations of DBM.
Given the generally limited movement of the DBM adults when host plants are abundant, local release of a suppressive gene-drive strain even at low frequencies could begin to have effects within one season. A suppressive gene-drive approach reverting insecticide resistance to susceptibility (Esvelt et al. Citation2014) may be a useful mechanism to assist in DBM population suppression. Insecticide (including Bt toxin) resistance has been successfully managed in several pest species, often using a combination of high-dose applications, pyramiding of active ingredients, and maintenance of untreated refuges (Ives et al. Citation2011). Management of insecticide resistance is only possible while the resistance allele frequencies remain low in target populations (Alstad and Andow Citation1995). DBM is prone to develop insecticide resistance, and most existing active ingredients are no longer useful against this pest (Grzywacz et al. Citation2010). In many cases, the genetic basis of resistance to these active ingredients has been determined (You et al. Citation2013). For some of these, it may be possible to deploy a gene-drive approach to return the resistance allele frequency to a level that would allow for management of resistance using other strategies. In the longer term, release of resistance-suppressive gene-drive strains of DBM could form an integral part of an integrative resistance management (IRM) strategy.
A major caveat to the development of gene drives against DBM is that between harvest and the next planting, when there are no host plants locally available, some DBM adults are likely to move long distances. With a gene drive system that has no minimum for release ratio needed to ensure successful drive, there would seem to be no method to contain the suppression strain to a single country, so trans-boundary issues would arise immediately. Before implementing such an approach, it may also be necessary determine if phenotypically-similar, distinct species are present, which could reduce the efficacy of the treatment. Indeed, a new diamondback moth species (Plutella australiana) was recently identified in Australia (Landry and Hebert Citation2013).
The red flour beetle: a worldwide pest of stored grains
The red flour beetle, Tribolium castaneum, is a global pest of stored grains and cereals. T. castaneum has a relatively short generation time, developing from egg to adult in approximately 4 weeks at 30°C (Sokoloff Citation1972, 1974, 1977). Eggs are laid in flour or grain and hatch within 3–5 days. Larvae burrow into flour or damaged kernels of grain and will feed for about 2–3 weeks until they become pupae, when they will spend 3–5 days undergoing major physical changes to develop the adult form. T. castaneum is a problem for agriculture because it has evolved a number of behavioral traits that help it survive and spread under adverse conditions. The first trait helps T. castaneum to deal with food shortages – larvae are cannibalistic and prey on eggs and pupae when food is scarce. This trait not only helps them survive periods of low-food supply, but is also thought to facilitate their adaptation to new environments (Via Citation1999). Second, T. castaneum are capable of broad dispersal. The global distribution of this beetle has long been thought to be due in large part to human conveyance. However, it was recently discovered that adults are strong flyers and actively disperse between spatially separated resources (Ridley et al. Citation2011). Ridley et al. (Citation2011) also discovered that the majority of beetles caught in their pheromone traps were females. Since a single female can produce thousands of offspring in a relatively short period of time, the migration of mated females is expected to have a major impact on beetle infestations in poorer countries where grain storage is less secure (i.e. not stored in well-maintained grain elevators). Together, these traits make T. castaneum an especially problematic pest in developing countries.
T. castaneum and other stored grain pests have traditionally been controlled via methyl bromide fumigation of grain elevators, granaries, and flour mills. However, use of methyl bromide has been banned due to its impact on atmospheric ozone (Gareau Citation2010). Despite having a worldwide ban on methyl bromide, exemptions still exist for the use of methyl bromide as a fumigant, since alternatives are more expensive, labor intensive, and/or simply not viable for particular applications (Fields and White Citation2002). Since T. castaneum is a greater problem in poorer countries, less expensive alternatives are needed. Is traditional SIT a viable option for T. castaneum population control? If we consider the key factors that Knipling lists for determining the suitability of a species for SIT-based control (Knipling Citation1955), T. castaneum does fairly well. These beetles (1) are easily reared, (2) have a relatively short generation time, (3) have good dispersal, (4) readily mate, and (5) can have low initial population sizes.
Since infestations in poorer countries are frequently the result of a few beetles invading locally stored grain, and migration of mated females pose a significant problem, skewing sex ratios could be a better option. How could this be accomplished? While there is still much to learn about sex determination in T. castaneum, several critical genes have already been identified. The T. castaneum doublesex (Tcdsx) gene produces sex-specific transcription factors critical for proper production of gametes (Shukla and Palli Citation2012a). The production of these specific transcription factors produced by Tcdsx transcripts is controlled by the proteins encoded by the T. castaneum transformer (Tctra) and Transformer-2 (Tctra-2) genes. Like Tcdsx, Tctra functions in a sex-specific way, but unlike Tcdsx, only the female version of Tctra RNA produces an active protein (Shukla and Palli Citation2012b). Interestingly, the female-specific TcTRA protein is necessary to maintain its own production (Shukla and Palli Citation2012a). TcTRA-2 is necessary for the sex-specific versions of both Tcdsx and Tctra (Shukla and Palli Citation2013). Because of their important roles in regulating sex determination and reproduction, each of these genes could be targeted to control pest populations. However, the most useful gene is likely to be Tctra. Reduced expression of this gene has little effect on males, but females are masculinized, and no female progeny are produced (Shukla and Palli Citation2012b). Skewing sex ratios could serve a number of purposes. SIT is more effective when only males are used (Rendon et al. Citation2004). Using males that can only produce male progeny would also be effective at reducing pest populations. If this male-only mutation is coupled with gene drive, then a self-maintaining population control can easily be established. Penetrance of this approach in target populations is dependent on random mating, so its success may be impacted by the high levels of pre- and post-zygotic reproductive incompatibilities present in Tribolium species, which can lead to insipient speciation events (Wade, Chang, and McNaughton Citation1995; Demuth and Wade Citation2007a, Citation2007b).
Despite its pest status, T. castaneum has emerged as a sophisticated model system. Since T. castaneum has a wealth of genetic and genomic tools, it is a logical choice for testing the feasibility and economic value of Cas9-mediated gene drive in a coleopteran. Among these tools is a sequenced and well-annotated genome (Tribolium Genome Sequencing Consortium et al. Citation2008), which provides the necessary sequences for Cas9 targeting. Furthermore, a number of essential genes have been identified (Lorenzen et al. Citation2007; Trauner et al. Citation2009; Ulrich et al. Citation2015), and their RNAi phenotypes characterized by the iBeetle Project (Ulrich et al. Citation2015), establishing which candidate genes are the most efficient to target for population reduction.
Interestingly, T. castaneum also possesses naturally occurring selfish genetic elements, known as Medea elements (Beeman, Friesen, and Denell Citation1992), which have the ability to drive tightly-linked genes into non-Medea populations. For example, a piggyBac transposon that contains the enhanced green fluorescent protein (EGFP) gene inserted very close to the Medea-1 element (∼1 centiMorgan away) (Lorenzen et al. Citation2008), has been shown to be inherited at super-Mendelian rates (5-fold higher than predicted) (Lorenzen et al. Citation2007). Medea might also provide a more stable and reliable gene-drive mechanism than Cas9-based systems, and efforts are currently underway in the Lorenzen lab to clarify this element’s mode of action, and to develop its use for pest control. Moreover, the existence of these elements in natural populations gives us an unprecedented opportunity to observe the stability and persistence of gene-drive elements as they spread through wild populations, work that cannot currently be done with transgenic mechanisms.
All of this research will be important in providing safer, cheaper methods for controlling T. castaneum in the developing world. T. castaneum research will also be important in developing Cas9-mediated gene-drive systems for beetles of greater economic importance, but with fewer genetic and genomic resources. Serious agricultural pests, such as the Western corn rootworm, Diabrotica virgifera virgifera, currently lack the genomic tools available to T. castaneum, so advances in using Cas9 gene drive in T. castaneum will lay important groundwork for similar research in these more critical pests.
Whitefly and other vectors of plant diseases
Plant sucking insects in the order Hemiptera include some of the world’s most important insect pests in agriculture. Their impact results not only from stress and damage caused by feeding, but also from the plant pathogens they transmit while probing plant tissue and feeding. Notable examples include: Diaphorina citri, vector of the bacterium Candidatus Liberibacter asiaticus which causes citrus greening disease, also known as huanglongbing (Hall et al. Citation2013); Nilaparvata lugens, one of the most important pests of rice because of its feeding damage and transmission of viruses (Bottrell and Schoenly Citation2012); and Bemisia tabaci, a species of whitefly known to feed on over 500 host plants, and to transmit many viruses from multiple genera, including Begomavirus, Torradovirus, Ipomovirus, and Crinivirus (Byrne and Bellows Citation1991; Luan et al. Citation2014).
Insecticides, and to a lesser extent biological control (parasitoids), have been used to control many species of Hemiptera. The plant-feeding habits of many of these insects make it likely that future population control strategies are likely to include the use of plant-based systemic RNA interference (RNAi) to some extent (Upadhyay et al. Citation2011). Basic functional genomics tools still need to be developed for many of these species to aid in identifying genes that can act as targets for plant-based gene silencing strategies. Genomic studies that elucidate the physiological genetics of Hemipterans will be important in designing and producing plants that are resistant to these insects and the diseases they carry. In particular, plant-feeding Hemiptera act as vectors of plant pathogenic viruses through a variety of mechanisms. Some insects essentially only serve as physical carriers of viruses; viruses simply attach to specialized regions of their mouthparts. In other cases, viruses infect the insect, in ways that may or may not involve viral replication, and then circulate within the insect (Whitfield, Falk, and Rotenberg Citation2015). The high degree of specificity involved with these virus/insect interactions involves a number of insect proteins and genes. However, in most cases, validating the function of these proteins/genes has been difficult because of the absence of genetic technologies that allow for rigorous testing of structure/function relationships. Consequently, there has been no confirmed identification of insect receptor proteins responsible for specific interactions with circulative viruses (Blanc, Drucker, and Uzest Citation2014). Thus, without a better molecular understanding of virus-host interactions it will be difficult to develop plant-resistance mechanisms or gene-drive systems that will inhibit viral transmission. Further, multiple anti-pathogen genes would need to be developed for species such as B. tabaci that are vectors for several viruses. For these species, a population suppression strategy is more feasible. While RNAi-based gene downregulation, through ingestion or injection, has proven highly effective in several species of Hemipterans, including Acyrthosiphon pisum, Lygus lineolaris, Oncopeltus fasciatus, Rhodnius prolixus, N. lugens, and B. tabaci (You et al. Citation2013), more sophisticated genetic manipulations involving both gene knockout and transgenic technologies are needed for the types of genetic experiments required to validate putative virus-receptor proteins in these insects.
Historically, Hemiptera have not been the focus of efforts to develop genetics-based population control programs like SIT. There are some species, such as B. tabaci, that might be amenable to gene drive-based control or eradication strategies for a number of reasons. First, B. tabaci is easy to rear and has life history characteristics that make the development and introduction of various genetic technologies highly feasible in the laboratory (David O’Brochta, unpublished results). B. tabaci is highly fecund with a short life cycle of about three weeks under appropriate temperature conditions. Its eggs are about 150–200 microns in length, are easily collected, and have a very thin, translucent chorion (outer membrane) that is easily penetrated by the glass needles used to inject genetic technologies (nucleic acids and/or proteins) into developing embryos (Crisione, O’Brochta, and Reid Citation2015). B. tabaci has a long, slow embryonic development requiring approximately nine days to complete. Blastoderm formation, the cutoff for effective injection of most genetic technologies, occurs between the sixth and ninth hours of development (David O’Brochta, unpublished results). This extended embryonic development is convenient for developing embryo microinjection protocols for preblastoderm embryos and performing the various manipulations needed to introduce gene drive or other genetic technologies into the germ-line of insects. Preblastoderm embryo microinjection is currently the only method for introducing genetic technologies into the germ-line of insects. B. tabaci have haplo/diploid sex determination, with haploid males developing from unfertilized eggs and females developing from fertilized eggs, making them excellent subjects for genetic studies. Haploidy simplifies a number of genetic analyses and procedures, such as mutant screens and the assembly of complex genotypes involving linked and unlinked loci, among other things. One consequence of haploidy is that Cas9-mediated gene drive would only occur in heterozygous females as the drive mechanism involves copying the Cas9 gene casette to the homologous chromosome (Esvelt et al. Citation2014), which cannot occur in haploids.
The second reason B. tabaci is amenable to gene drive-based control strategies is that, while the species is thought to have originated in Asia, it is currently widely distributed throughout the tropics and is a pest almost wherever it is found (Byrne and Bellows Citation1991). Because it is an introduced species in most locations, local eradication of B. tabaci is likely to be highly desirable and beneficial. In northern climates, it can be a significant pest in greenhouses. These contained environments might make gene drive-based population suppression and control strategies particularly feasible because the environment is highly controlled and the establishment of the species outdoors is precluded by cold winter temperatures, providing ideal biological containment. Indeed, this setting could be an ideal situation for developing and testing gene drive systems in general, making B. tabaci a potential model system for exploring these technologies in meaningful ways, as containment is likely to be a concern during the early phases of development of these technologies (Akbari et al. Citation2015).
Concluding remarks
SIT has been very successfully used for control of a few major agricultural pest insect species. Transgenic male-only strains that carry dominant female-lethal genes offer a potentially significant improvement over traditional SIT but nevertheless may not be economical for some pest species. Insect pest population suppression via Cas9-mediated genetic drive systems could provide an economical and effective method for control. To achieve suppression, the drive would target genes that are essential for females but not males, or required for germ-cell development or reproduction in one sex. Since targeting such genes would make it difficult to rear a strain in a factory, we argue that drives should be conditional so that drive would take place in the field but not during rearing. For example, by controlling Cas9 expression with the tetracycline transactivator, Cas9 is only produced if the insect diet lacks tetracycline. Construction of modified insect strains with gene drives will benefit from ongoing genome sequencing efforts for rapid identification of gene promoters with the desired properties such as germ-line specific activity. However, a major impediment for some pest species is our current inability to deliver DNA to the germline and select for genetically modified insects.
With a drive mechanism that targets a gene essential for female development or reproduction, far fewer transgenic males would need to be released than strains that carry a conditional female-lethal gene and produce only males when raised on diet that lacks tetracycline. Although both approaches could involve disrupting normal female development, the gene drive approach is more efficient due to the non-Mendelian copying mechanism that spreads the transgene through the targeted population. Although transgenic strains carrying conditional female-lethal genes have been developed for several important agricultural insect pests (Ant et al. Citation2012; Schetelig and Handler Citation2012; Jin et al. Citation2013; Li et al. Citation2014; Concha et al. Citation2016), they have not yet been utilized for pest control. For these strains, multiple successive releases of transgenic males in large excess over the wild-type males (e.g. 10:1) would need to be performed to achieve population suppression (Schliekelman and Gould Citation2000). The need for multiple releases of relatively large numbers of insects makes such genetic control programs relatively expensive. In contrast, only a single release of transgenic males carrying a female-specific gene drive at 1:10 ratio relative to wild type would be necessary to suppress a population after a few generations. The ability to release far fewer males (100× or more) and yet achieve population reduction is a direct consequence of the copying mechanism of the Cas9-mediated gene drive. Consequently, genetic control using a gene drive system should be more economical than the release of males carrying a conditional female-lethal gene.
All of these general conclusions are based on the expectation that the gene drive mechanisms will function as expected and that the decrease or elimination of the target organism will not cause unexpected problems. Therefore, prior to any release of a strain with a gene drive it will be important to carry out ecological studies investigating the possible impact of pest suppression. The strains will likely express more than one guide RNA complementary to highly conserved regions of the targeted genes which is expected to decrease the potential for the target species to evolve resistance to the drive mechanism or the female-lethal/fertility gene. Nevertheless, we should anticipate the development of resistance to the gene drive mechanism at some point after the release and have backup plans in case this happens. If a gene drive suppresses a population for a number of years without complete eradication, practioners could become complacent and be caught off guard by rapid resistance evolution. To avoid such a scenario, post-release monitoring for resistance will be needed. If a second strain with a different gene drive mechanism is available, monitoring could trigger release of the second strain. Alternatively, SIT could be used to maintain local population suppression/eradication as the released sterile males would mate with all females in the area, including those that may carry resistance to the initial gene drive.
Disclosure statement
No potential conflict of interest was reported by the authors.
Notes on contributors
Maxwell J. Scott is a professor of entomology at North Carolina State University with a specialty in developing genetically modified strains of insect pests for genetic control programs. Max received a PhD in Cell Biology from Baylor College of Medicine in Houston, Texas. The insect pests of most interest are the Australian sheep blowfly, the New World screwworm, spotted wing Drosophila and the human disease vector Aedes aegypti.
Fred Gould is a Distinguished University Professor of Entomology and is Co-Director of the Genetic Engineering and Society Center of North Carolina State University. He studies the ecology and genetics of pests to improve food production and human and environmental health. Dr. Gould conducts research on the application of evolutionary biology and population genetics to enable sustainable use of insect resistant crops. He also does research aimed at development of strategies for using engineered insect vectors of pathogens to decrease human disease. In 2011, he was elected to the U.S. National Academy of Sciences (NAS). He recently chaired the NASEM–NRC committee on ‘Genetically Engineered Crops: Experiences and Prospects’.
Marcé Lorenzen is an Associate Professor of Entomology at North Carolina State University. She uses a range of functional genomic tools, including germline transformation and CRISPR/Cas9-genome editing to study the selfish behavior of Medea elements in the red flour beetle. One of her research goals is to utilize Medea-based gene drive systems in agricultural pests such as the western corn rootworm.
Nathaniel Grubbs is a postdoctoral research associate in the lab of Dr. Marcé Lorenzen. He received his PhD in Genetics from North Carolina State University, and now works to deploy the tools of molecular genetics to study non-model pest insects.
Owain Edwards, research at CSIRO has focused primarily on the molecular basis of aphid-host plant interactions, leading to board membership in the International Aphid Genomics Consortium (IAGC) and strong collaborations with the Institute of Zoology (CAS Beijing), Kansas State University (USA), INRA Rennes (France) and BGI Shenzhen (China). His work on aphids has broadened to examine the molecular basis of all aphid interactions with their environment, including the genetic and epigenetic factors controlling aphid polyphenism and the molecular basis of insecticide resistance. Building on his expertise in invertebrate genomics, he leads a CSIRO research program in genetic pest control technologies. Most recently, Dr Edwards was given a leadership role in the development of CSIRO's new Future Science Platform in Synthetic Biology. Within this platform, Dr Edwards oversees projects delivering environmental outcomes including gene drives for biological control, and engineering resistance/resilience into threatened ecosystems.
David A. O'Brochta is a Scientific Program Manager at the Foundation for the National Institutes of Health, and Professor Emeritus in the Department of Entomology at the University of Maryland College Park. As a Program Manager he is involved in providing technical expertise in the area of gene drive and developing programs that address issues of policy, regulation and governance associated with gene drive research and development. As a professor at the University of Maryland he conducted research on the interactions between malaria parasites and Anopheles mosquitoes and developed genetic technologies enabling function genomics of non-model insect systems.
ORCID
Maxwell J. Scott http://orcid.org/0000-0001-6536-4735
Marcé Lorenzen http://orcid.org/0000-0003-2318-1388
Nathaniel Grubbs http://orcid.org/0000-0002-3631-7427
Additional information
Funding
References
- Akbari, O. S., H. J. Bellen, E. Bier, S. L. Bullock, A. Burt, G. M. Church, K. R. Cook, et al. 2015. “BIOSAFETY. Safeguarding Gene Drive Experiments in the Laboratory.” Science 349 (6251): 927–929. doi:10.1126/science.aac7932.
- Alexander, J. L. 2006. “Screwworms.” Journal of the American Veterinary Medical Association 228 (3): 357–367. doi:10.2460/javma.228.3.357.
- Alstad, D. N., and D. A. Andow. 1995. “Managing the Evolution of Insect Resistance to Transgenic Plants.” Science 268 (5219): 1894–1896. doi:10.1126/science.268.5219.1894.
- Ant, T., M. Koukidou, P. Rempoulakis, H. F. Gong, A. Economopoulos, J. Vontas, and L. Alphey. 2012. “Control of the Olive Fruit fly Using Genetics-enhanced Sterile Insect Technique.” BMC Biology 10: 51. doi:10.1186/1741-7007-10-51.
- APHIS, USDA. 2014. Proposal to Permit the Field Release of Genetically Engineered Diamondback Moth in New York: Environmental Assessment.” In, 1–141.
- Asplen, MarkK, Gianfranco Anfora, Antonio Biondi, Deuk-Soo Choi, Dong Chu, KentM Daane, Patricia Gibert, et al. 2015. “Invasion Biology of Spotted Wing Drosophila (Drosophila suzukii): A Global Perspective and Future Priorities.” Journal of Pest Science 88 (3): 469–494. doi:10.1007/s10340-015-0681-z.
- Beeman, R. W., K. S. Friesen, and R. E. Denell. 1992. “Maternal-effect Selfish Genes in Flour Beetles.” Science 256 (5053): 89–92. doi: 10.1126/science.1566060
- Bhaya, D., M. Davison, and R. Barrangou. 2011. “CRISPR-Cas Systems in Bacteria and Archaea: Versatile Small RNAs for Adaptive Defense and Regulation.” Annual Review of Genetics 45: 273–297. doi:10.1146/annurev-genet-110410-132430.
- Blanc, S., M. Drucker, and M. Uzest. 2014. “Localizing Viruses in Their Insect Vectors.” In Annual Review of Phytopathology, Vol 52, edited by N. K. VanAlfen, 403–425.
- Bottrell, D. G., and K. G. Schoenly. 2012. “Resurrecting the Ghost of Green Revolutions Past: The Brown Planthopper as a Recurring Threat to High-Yielding Rice Production in Tropical Asia.” Journal of Asia-Pacific Entomology 15 (1): 122–140. doi: 10.1016/j.aspen.2011.09.004
- Burt, A. 2003. “Site-specific Selfish Genes as Tools for the Control and Genetic Engineering of Natural Populations.” Proceedings of the Royal Society B: Biological Sciences 270 (1518): 921–928. doi:10.1098/rspb.2002.2319.
- Byrne, D. N., and T. S. Bellows. 1991. “Whitefly Biology.” Annual Review of Entomology 36 (1): 431–457. doi:10.1146/annurev.en.36.010191.002243.
- Carvalho, D. O., D. Nimmo, N. Naish, A. R. McKemey, P. Gray, A. B. Wilke, M. T. Marrelli, J. F. Virginio, L. Alphey, and M. L. Capurro. 2014. “Mass Production of Genetically Modified Aedes aegypti for Field Releases in Brazil.” Journal of Visualized Experiments (83): e3579. doi:10.3791/3579.
- Casida, J. E., and G. B. Quistad. 1998. “Golden Age of Insecticide Research: Past, Present, or Future?” Annual Review of Entomology 43: 1–16. doi:10.1146/annurev.ento.43.1.1.
- Catteruccia, F., J. P. Benton, and A. Crisanti. 2005. “An Anopheles Transgenic Sexing Strain for Vector Control.” Nature Biotechnology 23 (11): 1414–1417. doi: 10.1038/nbt1152
- Chapman, J. W., D. R. Reynolds, A. D. Smith, J. R. Riley, D. E. Pedgley, and I. P. Woiwod. 2002. “High-altitude Migration of the Diamondback Moth Plutella xylostella to the UK: A Study Using Radar, Aerial Netting, and Ground Trapping.” Ecological Entomology 27: 641–650. doi: 10.1046/j.1365-2311.2002.00472.x
- Chaudhury, M. F., and S. R. Skoda. 2007. “A Cellulose Fiber-based Diet for Screwworm (Diptera: Calliphoridae) larvae.” Journal of Economic Entomology 100 (1): 241–245. doi: 10.1093/jee/100.1.241
- Chen, C. H., H. Huang, C. M. Ward, J. T. Su, L. V. Schaeffer, M. Guo, and B. A. Hay. 2007. “A Synthetic Maternal-effect Selfish Genetic Element Drives Population Replacement in Drosophila.” Science 316 (5824): 597–600. doi:10.1126/science1138595 doi: 10.1126/science.1138595
- Chiu, J. C., X. Jiang, L. Zhao, C. A. Hamm, J. M. Cridland, P. Saelao, K. A. Hamby, et al. 2013. “Genome of Drosophila suzukii, the Spotted Wing Drosophila.” G3 (Bethesda) 3 (12): 2257–2271. doi:10.1534/g3.113.008185.
- Cini, A., G. Anfora, L. A. Escudero-Colomar, A. Grassi, U. Santosuosso, G. Seljak, and A. Papini. 2014. “Tracking the Invasion of the Alien Fruit Pest Drosophila suzukii in Europe.” Journal of Pest Science 87 (4): 559–566. doi:10.1007/s10340-014-0617-z.
- Concha, C., A. Palavesam, F. D. Guerrero, A. Sagel, F. Li, J. A. Osborne, Y. Hernandez, et al. 2016. “A Transgenic Male-Only Strain of the New World Screwworm for an Improved Control Program Using the Sterile Insect Technique.” BMC Biology 14: 72. doi:10.1186/s12915-016-0296-8.
- Concha, C., and M. J. Scott. 2009. “Sexual Development in Lucilia cuprina (Diptera, Calliphoridae) Is Controlled by the Transformer Gene.” Genetics 182 (3): 785–798. doi:10.1534/genetics.109.100982.
- Crisione, F., D. A. O’Brochta, and W. Reid. 2015. “Genetic Technologies for Disease Vectors.” Current Opinion in Insect Science 10: 90–97. doi: 10.1016/j.cois.2015.04.012
- Crystal, M. M. 1979. “Sterilization of Screwworm Flies (Diptera: Calliphoridae) with Gamma Rays: Restudy After Two Decades.” Journal of Medical Entomology 15 (2): 103–108. doi: 10.1093/jmedent/15.2.103
- Culliney, T. W. 2014. “Crop Losses Due to Arthropods.” In Integrated Pest Management: Pesticide Problems, edited by D. Pimentel, and P. Peshin, 201–225. Dordrecht: Springer.
- Curtis, C. F. 1968. “Possible Use of Translocations to Fix Desirable Genes in Insect Pest Populations.” Nature 218 (5139): 368–369. doi: 10.1038/218368a0
- Demuth, J. P., and M. J. Wade. 2007a. “Population Differentiation in the Beetle Tribolium castaneum. I. Genetic Architecture.” Evolution 61 (3): 494–509. doi:10.1111/j.1558-5646.2007.00049.x doi: 10.1111/j.1558-5646.2007.00048.x
- Demuth, J. P., and M. J. Wade. 2007b. “Population Differentiation in the Beetle Tribolium castaneum. II. Haldane’s Rule and Incipient Speciation.” Evolution 61 (3): 694–699. doi:10.1111/j.1558-5646.2007.00049.x.
- Deredec, A., A. Burt, and H. C. Godfray. 2008. “The Population Genetics of Using Homing Endonuclease Genes in Vector and Pest Management.” Genetics 179 (4): 2013–2026. doi:10.1534/genetics.108.089037.
- Diepenbrock, L. M., J. A. Hardin, and H. J. Burrack. 2017. “Season-Long Programs for Control of Drosophila suzukii in Southeastern U.S. Blackberries.” Crop Protection 98: 149–156. doi:10.1016/j.cropro.2017.03.022.
- Diepenbrock, L. M., D. O. Rosenstell, J. A. Hardin, A. A. Sial, and H. J. Burrack. 2016. “Season-Long Programs for Control of Drosophila suzukii in Southeastern U.S. Blueberries.” Crop Protection 81: 76–84. doi:10.1016/j.cropro.2015.12.012.
- Enkerlin, W. R. 2005. “Impact of Fruit Fly Programmes Using the Sterile Insect Technique.” In Sterile Insect Technique. Principles and Practice in Area-Wide Integrated Pest Management, edited by V. A. Dyck, J. Hendrichs, and A. S. Robinson, 651–676. Dordrecht: Springer.
- Esvelt, K. M., A. L. Smidler, F. Catteruccia, and G. M. Church. 2014. “Concerning RNA-Guided Gene Drives for the Alteration of Wild Populations.” Elife 3: e03401. doi:10.7554/eLife.03401.
- Fields, P. G., and N. D. G. White. 2002. “Alternatives to Methyl Bromide Treatments for Stored-Product and Quarantine Insects.” Annual Review of Entomology 47: 331–359. doi: 10.1146/annurev.ento.47.091201.145217
- Fu, X. W., Z. L. Xing, Z. F. Liu, A. Ali, and K. M. Wu. 2014. “Migration of Diamondback Moth, Plutella xylostella, Across the Bohai Sea in Northern China.” Crop Protection 64: 143–149. doi: 10.1016/j.cropro.2014.06.021
- Furlong, M. J., D. J. Wright, and L. M. Dosdall. 2013. “Diamondback Moth Ecology and Management: Problems, Progress, and Prospects.” Annual Review of Entomology 58: 517–541. doi:10.1146/annurev-ento-120811-153605.
- Gareau, B. J. 2010. “A Critical Review of the Successful CFC Phase-Out Versus the Delayed Methyl Bromide Phase-Out in the Montreal Protocol.” International Environmental Agreements: Politics, Law and Economics 10 (3): 209–231. doi:10.1007/s10784-010-9120-z.
- Gossen, M., and H. Bujard. 1992. “Tight Control of Gene Expression in Mammalian Cells by Tetracycline-Responsive Promoters.” Proceedings of the National Academy of Sciences 89 (12): 5547–5551. doi: 10.1073/pnas.89.12.5547
- Grzywacz, D., A. Rossbach, A. Rauf, D. A. Russell, R. Srinivasan, and A. M. Shelton. 2010. “Current Control Methods for Diamondback Moth and Other Brassica Insect Pests and the Prospects for Improved Management with Lepidopteran-Resistant Bt Vegetable Brassicas in Asia and Africa.” Crop Protection 29: 68–79. doi: 10.1016/j.cropro.2009.08.009
- Hall, D. G., M. L. Richardson, E. D. Ammar, and S. E. Halbert. 2013. “Asian Citrus Psyllid, Diaphorina citri, Vector of Citrus Huanglongbing Disease.” Entomologia Experimentalis et Applicata 146 (2): 207–223. doi:10.1111/eea.12025.
- Harvey-Samuel, T., T. Ant, H. Gong, N. I. Morrison, and L. Alphey. 2014. “Population-level Effects of Fitness Costs Associated with Repressible Female-Lethal Transgene Insertions in two Pest Insects.” Evolutionary Applications 7 (5): 597–606. doi:10.1111/eva.12159.
- Ives, A. R., P. R. Glaum, N. L. Ziebarth, and D. A. Andow. 2011. “The Evolution of Resistance to Two-Toxin Pyramid Transgenic Crops.” Ecological Applications 21 (2): 503–515. doi: 10.1890/09-1869.1
- Jin, L., A. S. Walker, G. Fu, T. Harvey-Samuel, T. H. Dafa’alla, A. Miles, T. Marubbi, et al. 2013. “Engineered Female-Specific Lethality for Control of Pest Lepidoptera.” ACS Synthetic Biology 2 (3): 160–166. doi: 10.1021/sb300123m
- Jinek, M., K. Chylinski, I. Fonfara, M. Hauer, J. A. Doudna, and E. Charpentier. 2012. “A Programmable Dual-RNA-Guided DNA Endonuclease in Adaptive Bacterial Immunity.” Science 337 (6096): 816–821. doi:10.1126/science.1225829.
- Klassen, W., and C. F. Curtis. 2005. “History of the Sterile Insect Technique.” In Sterile Insect Technique. Principles and Practice in Area-Wide Integrated Pest Management, edited by V. A. Dyck, J. Hendrichs, and A. S. Robinson, 3–36. Dordrecht: Springer.
- Knipling, E. F. 1955. “Possibilities of Insect Control or Eradication Through the Use of Sexually Sterile Males.” Journal of Economic Entomology 48 (4): 459–462. doi: 10.1093/jee/48.4.459
- Knipling, E. F. 1960. “The Eradication of the Screw-Worm fly.” Scientific American 203 (4): 54–61. doi: 10.1038/scientificamerican1060-54
- Koukidou, M., and L. Alphey. 2014. “Practical Applications of Insects’ Sexual Development for Pest Control.” Sexual Development 8 (1–3): 127–136. doi:10.1159/000357203.
- Krafsur, E. S. 1998. “Sterile Insect Technique for Suppressing and Eradicating Insect Population: 55 Years and Counting.” Journal of Agricultural Entomology 15 (4): 303–317.
- Kunz, S. E., K. D. Murrell, G. Lambert, L. F. James, and C. E. Terrill. 1991. “Estimated Losses of Livestock to Pests.” In CRC Handbook of Pest Management in Agriculture, edited by D. Pimentel, 68–69. Boca Raton, FL: CRC Press.
- Lachance, L. E., A. C. Bartlett, R. A. Bram, R. J. Gagne, O. H. Graham, D. O. McInnis, C. J. Whitten, and J. A. Seawright. 1982. “Mating Types in Screwworm Populations?” Science 218 (4577): 1142–1143. doi:10.1126/science.218.4577.1142.
- Landry, J. F., and P. D. Hebert. 2013. “Plutella australiana (Lepidoptera, Plutellidae), an Overlooked Diamondback Moth Revealed by DNA Barcodes.” Zookeys 327: 43–63. doi:10.3897/zookeys.327.5831.
- Lee, J. C., D. J. Bruck, H. Curry, D. Edwards, D. R. Haviland, R. A. Van Steenwyk, and B. M. Yorgey. 2011. “The Susceptibility of Small Fruits and Cherries to the Spotted-Wing Drosophila, Drosophila suzukii.” Pest Management Science 67 (11): 1358–1367. doi:10.1002/ps.2225.
- Lee, J. C., D. J. Bruck, A. J. Dreves, C. Ioriatti, H. Vogt, and P. Baufeld. 2011. “In Focus: Spotted Wing Drosophila, Drosophila suzukii, Across Perspectives.” Pest Management Science 67 (11): 1349–1351. doi:10.1002/ps.2271.
- Li, F., and M. J. Scott. 2016. “CRISPR/Cas9-Mediated Mutagenesis of the White and Sex Lethal Loci in the Invasive Pest, Drosophila suzukii.” Biochemical and Biophysical Research Communications 469 (4): 911–916. doi:10.1016/j.bbrc.2015.12.081.
- Li, F., S. P. Vensko, 2nd, E. J. Belikoff, and M. J. Scott. 2013. “Conservation and Sex-Specific Splicing of the transformer Gene in the Calliphorids Cochliomyia hominivorax, Cochliomyia macellaria and Lucilia sericata.” PLoS One 8 (2): e56303. doi:10.1371/journal.pone.0056303.
- Li, F., H. A. Wantuch, R. J. Linger, E. J. Belikoff, and M. J. Scott. 2014. “Transgenic Sexing System for Genetic Control of the Australian Sheep Blow fly Lucilia cuprina.” Insect Biochemistry and Molecular Biology 51: 80–88. doi:10.1016/j.ibmb.2014.06.001.
- Lorenzen, M. D., A. Gnirke, J. Margolis, J. Garnes, M. Campbell, J. J. Stuart, R. Aggarwal, S. Richards, Y. Park, and R. W. Beeman. 2008. “The Maternal-Effect, Selfish Genetic Element Medea is Associated with a Composite Tc1 Transposon.” Proceedings of the National Academy of Sciences 105 (29): 10085–10089. doi:10.1073/pnas.0800444105.
- Lorenzen, M. D., T. Kimzey, T. D. Shippy, S. J. Brown, R. E. Denell, and R. W. Beeman. 2007. “piggyBac-based Insertional Mutagenesis in Tribolium castaneum Using Donor/Helper Hybrids.” Insect Molecular Biology 16 (3): 265–275. doi:10.1111/j.1365-2583.2007.00727.x.
- Luan, J. B., X. W. Wang, J. Colvin, and S. S. Liu. 2014. “Plant-mediated Whitefly-Begomovirus Interactions: Research Progress and Future Prospects.” Bulletin of Entomological Research 104 (3): 267–276. doi:10.1017/s000748531400011x.
- Mali, P., K. M. Esvelt, and G. M. Church. 2013. “Cas9 as a Versatile Tool for Engineering Biology.” Nature Methods 10 (10): 957–963. doi:10.1038/nmeth.2649.
- Mastrangelo, T., and J. B. Welch. 2012. “An Overview of the Components of AW-IPM Campaigns Against the New World Screwworm.” Insects 3 (4): 930–955. doi:10.3390/insects3040930.
- Melvin, R., and R. C. Bushland. 1936. “A Method of Rearing Cochliomyia americana C. & P. on Artificial Media.” USDA Bur. Entomol. Plant Quar. ET-88.
- Min, J., A. Smidler, and K. Esvelt. 2017. “Harnessing Gene Drive.” Journal of Responsible Innovation 5 (S1): S40–S65.
- Mo, J., G. Baker, M. Keller, and R. Roush. 2003. “Local Dispersal of the Diamondback Moth (Plutella xylostella (L.)) (Lepidoptera: Plutellidae).” Environmental Entomology 32: 71–79. doi: 10.1603/0046-225X-32.1.71
- Rendon, P., D. McInnis, D. Lance, and J. Stewart. 2004. “Medfly (Diptera: Tephritidae) Genetic Sexing: Large-scale Field Comparison of Males-Only and Bisexual Sterile Fly Releases in Guatemala.” Journal of Economic Entomology 97 (5): 1547–1553. doi: 10.1603/0022-0493-97.5.1547
- Tribolium Genome Sequencing Consortium, Richards, S., R. A. Gibbs, G. M. Weinstock, S. J. Brown, R. Denell, R. W. Beeman, R. Gibbs, et al. 2008. “The Genome of the Model Beetle and Pest Tribolium castaneum.” Nature 452 (7190): 949–955. doi:10.1038/nature06784.
- Ridley, A. W., J. P. Hereward, G. J. Daglish, S. Raghu, P. J. Collins, and G. H. Walter. 2011. “The Spatiotemporal Dynamics of Tribolium castaneum (Herbst): Adult Flight and Gene Flow.” Molecular Ecology 20 (8): 1635–1646. doi: 10.1111/j.1365-294X.2011.05049.x
- Riggin-Bucci, T., and F. Gould. 1997. “Impact of Intraplot Mixtures of Toxic and Nontoxic Plants on Population Dynamics of Diamondback Moth (Lepidoptera: Plutellidae) and Its Natural Enemies.” Journal of Economic Entomology 90: 241–251. doi: 10.1093/jee/90.2.241
- Schetelig, M. F., and A. M. Handler. 2012. “A Transgenic Embryonic Sexing System for Anastrepha suspensa (Diptera: Tephritidae).” Insect Biochemistry and Molecular Biology 42 (10): 790–795. doi:10.1016/j.ibmb.2012.07.007.
- Schliekelman, P., and F. Gould. 2000. “Pest Control by the Release of Insects Carrying a Female-killing Allele on Multiple Loci.” Journal of Economic Entomology 93 (6): 1566–1579. doi: 10.1603/0022-0493-93.6.1566
- Schupbach, T. 1982. “Autosomal Mutations that Interfere with Sex Determination in Somatic Cells of Drosophila Have no Direct Effect on the Germline.” Developmental Biology 89 (1): 117–127. doi: 10.1016/0012-1606(82)90300-1
- Schupbach, T. 1985. “Normal Female Germ Cell Differentiation Requires the Female X Chromosome to Autosome Ratio and Expression of sex-lethal in Drosophila melanogaster.” Genetics 109 (3): 529–548.
- Scott, M. J. 2014. “Development and Evaluation of Male-Only Strains of the Australian Sheep Blowfly, Lucilia cuprina.” BMC Genetics 15 (2): S3. doi: 10.1186/1471-2156-15-S2-S3
- Scott, M. J., C. Concha, J. B. Welch, P. L. Phillips, and S. R. Skoda. 2017. “Research Advances in the Screwworm Eradication Program Over the Past 25 Years.” Entomologia Experimentalis et Applicata in press, 164: 226–236. doi: 10.1111/eea.12607
- Scott, M. J., M. L. Pimsler, and A. M. Tarone. 2014. “Sex Determination Mechanisms in the Calliphoridae (Blow Flies).” Sexual Development 8 (1–3): 29–37. doi: 10.1159/000357132
- Shirai, Y., and A. Nakamura. 1994. “Dispersal Movement of Male Adults of the Diamondback Moth, Plutella xylostella (Lepidoptera: Yponomeutidae), on Cruciferous Vegetable Fields, Studied Using the Mark-Recapture Method.” Applied Entomology and Zoology 29: 339–348. doi: 10.1303/aez.29.339
- Shukla, J. N., and S. R. Palli. 2012a. “Doublesex Target Genes in the Red Flour Beetle, Tribolium castaneum.” Scientific Reports 2: 175. doi: 10.1038/srep00948
- Shukla, J. N., and S. R. Palli. 2012b. “Sex Determination in Beetles: Production of All Male Progeny by Parental RNAi Knockdown of transformer.” Scientific Reports 2: 117. doi:10.1038/srep00602.
- Shukla, J. N., and S. R. Palli. 2013. “Tribolium castaneum Transformer-2 Regulates Sex Determination and Development in Both Males and Females.” Insect Biochemistry and Molecular Biology 43 (12): 1125–1132. doi:10.1016/j.ibmb.2013.08.010.
- Sokoloff, A. 1972, 1974, 1977. The Biology of Tribolium with Special Emphasis on Genetic Aspects I–III. Oxford: Clarendon Press and Oxford Univ. Press.
- Sutrisno, S., and M. Hoedaya. 1993. The Sterile Insect Technique and Transmission of Inherited Sterility to Control The Diamondback Moth, plutella xylostella (l.), and the Cabbage Web Worm, crocidolomia binotalis zell. Paper Presented at the Management of Insect Pests: Nuclear and Related Molecular and Genetic Techniques, Vienna.
- Tabashnik, B. E. 1994. “Evolution of Resistance to Bacillus thuringiensis.” Annual Review of Entomology 39: 47–79. doi: 10.1146/annurev.en.39.010194.000403
- Talekar, N. S., and A. M. Shelton. 1993. “Biology, Ecology, and Management of the Diamondback Moth.” Annual Review of Entomology 38: 275–301. doi: 10.1146/annurev.en.38.010193.001423
- Thomas, D. D., C. A. Donnelly, R. J. Wood, and L. S. Alphey. 2000. “Insect Population Control Using a Dominant, Repressible, Lethal Genetic System.” Science 287 (5462): 2474–2476. doi:10.1126/science.287.5462.2474.
- Tochen, S., D. T. Dalton, N. Wiman, C. Hamm, P. W. Shearer, and V. M. Walton. 2014. “Temperature-related Development and Population Parameters for Drosophila suzukii (Diptera: Drosophilidae) on Cherry and Blueberry.” Environmental Entomology 43 (2): 501–510. doi: 10.1603/EN13200
- Trauner, J., J. Schinko, M. D. Lorenzen, T. D. Shippy, E. A. Wimmer, R. W. Beeman, M. Klingler, G. Bucher, and S. J. Brown. 2009. “Large-scale Insertional Mutagenesis of a Coleopteran Stored Grain Pest, the Red Flour Beetle Tribolium castaneum, Identifies Embryonic Lethal Mutations and Enhancer Traps.” BMC Biology 7: 73. doi:10.1186/1741-7007-7-73.
- Tween, G., and P. Rendon. 2007. “Current Advances in the Use of Cryogenics and Aerial Navigation Technologies for Sterile Insect Delivery Systems.” In Area-wide Control of Insect Pests, edited by M. J. B. Vreysen, A. S. Robinson, and J. Hendrichs, 603–615. Dordrecht: Springer.
- Ulrich, J., V. A. Dao, U. Majumdar, C. Schmitt-Engel, J. Schwirz, D. Schultheis, N. Strohlein, et al. 2015. “Large Scale RNAi Screen in Tribolium Reveals Novel Target Genes for Pest Control and the Proteasome as Prime Target.” BMC Genomics 16: 2270. doi:10.1186/s12864-015-1880-y.
- Unckless, R. L., A. G. Clark, and P. W. Messer. 2017. “Evolution of Resistance Against CRISPR/Cas9 Gene Drive.” Genetics 205: 827–841. doi:10.1534/genetics.116.197285.
- Upadhyay, S. K., K. Chandrashekar, N. Thakur, P. C. Verma, J. F. Borgio, P. K. Singh, and R. Tuli. 2011. “RNA Interference for the Control of Whiteflies (Bemisia tabaci) by Oral Route.” Journal of Biosciences 36 (1): 153–161. doi:10.1007/s12038-011-9009-1.
- Urlinger, S., U. Baron, M. Thellmann, M. T. Hasan, H. Bujard, and W. Hillen. 2000. “Exploring the Sequence Space for Tetracycline-Dependent Transcriptional Activators: Novel Mutations Yield Expanded Range and Sensitivity.” Proceedings of the National Academy of Sciences 97 (14): 7963–7968. doi: 10.1073/pnas.130192197
- Vargas-Teran, M., H. C. Hofmann, and N. E. Tweddle. 2005. “Impact of Screwworm Eradication Programmes Using the Sterile Insect Technique.” In Sterile Insect Technique. Principles and Practice in Area-Wide Integrated Pest Management, edited by V. A. Dyck, J. Hendrichs, and A. S. Robinson, 629–650. Dordrecht: Springer.
- VerCauteren, K. 2003. “The Deer Boom: Discussins on Population Growth and Range Expansion of the White-Tailed Deer.” In Bowhunting Records of North American Whitetail Deer. Pope and Young Club. Chatfield,MN: USDA National Wildlife Research Center - Staff Publications. Paper 281.
- Via, S. 1999. “Cannibalism Facilitates the Use of a Novel Environment in the Flour Beetle, Tribolium castaneum.” Heredity (Edinb) 82 (Pt 3): 267–275. doi: 10.1038/sj.hdy.6884820
- Wade, M. J., N. W. Chang, and M. McNaughton. 1995. “Incipient Speciation in the Flour Beetle, Tribolium confusum: Premating Isolation Between Natural Populations.” Heredity (Edinb) 75 (Pt 5): 453–459. doi: 10.1038/hdy.1995.161
- Wang, X., and Y. Wu. 2012. “High Levels of Resistance to Chlorantraniliprole Evolved in Field Populations of Plutella xylostella.” Journal of Economic Entomology 105 (3): 1019–1023. doi: 10.1603/EC12059
- Webber, B. L., S. Raghu, and O. R. Edwards. 2015. “Opinion: Is CRISPR-Based Gene Drive a Biocontrol Silver Bullet or Global Conservation Threat?” Proceedings of the National Academy of Sciences 112 (34): 10565–10567. doi: 10.1073/pnas.1514258112
- Whitfield, A. E., B. W. Falk, and D. Rotenberg. 2015. “Insect Vector-Mediated Transmission of Plant Viruses.” Virology 479–480: 278–289. doi: 10.1016/j.virol.2015.03.026
- Wolverton, S., J. H. Kennedy, and J. D. Cornelius. 2007. “A Paleozoological Perspective on White-Tailed Deer (Odocoileus virginianus texana) Population Density and Body Size in Central Texas.” Environmental Management 39 (4): 545–552. doi: 10.1007/s00267-006-0240-8
- Wyss, J. H. 2000. “Screwworm Eradication in the Americas.” Annals of the New York Academy of Sciences 916: 186–193. doi: 10.1111/j.1749-6632.2000.tb05289.x
- You, M., Z. Yue, W. He, X. Yang, G. Yang, M. Xie, D. Zhan, et al. 2013. “A Heterozygous Moth Genome Provides Insights Into Herbivory and Detoxification.” Nature Genetics 45 (2): 220–225. doi: 10.1038/ng.2524
- Zalucki, M. P., A. Shabbir, R. Silva, D. Adamson, L. Shu-Sheng, and M. J. Furlong. 2012. “Estimating the Economic Cost of One of the World’s Major Insect Pests, Plutella xylostella (Lepidoptera: Plutellidae): Just How Long is a Piece of String?” Journal of Economic Entomology 105 (4): 1115–1129. doi: 10.1603/EC12107