Abstract
Marine natural products have recently been recognized as a promising source of NF-κB inhibitors. In this study, extracts of bacterial isolates from the coral Favis sp. were screened for NF-κB modulatory capability using an NF-κB luciferase reporter gene assay. The bacterial extracts had variable effects on NF-κB activity: from the 39 extracts tested, only one exhibited significant NF-κB inhibition and two caused NF-κB up regulation. In addition, we showed that coral bacteria New-33 extract inhibits NF-κB alternative pathway subunits in a non-cytotoxic manner. HPLC analysis confirmed the presence of a low molecular mass compound and NCBI megaBLAST revealed that the New-33 16S rRNA gene sequences are similar to Vibrio mediterranei. This specific modulation of the NF-κB by alternative pathway could contribute to therapeutic implications and also raises questions about host-symbiont interaction in the marine environment.
Public Interest Statement
Considering the great potentials of active molecules from natural sources in the treatment of cancer and inflammation, many small molecules are identified to combat against diseases. Among which, the marine source is a unique resource in search of unique biologically active chemicals which we can translate into novel biomedicines. Unlike the undesirable side effects produced by the synthetic molecules, natural products are preferred to overcome the unwanted consequences. NF-κB is a pharmacologically important protein which is involved in inflammation and cancer by their upstream molecular mechanisms in various types of cancer. Therefore, inhibition of NF-κB activity and suppression of their sub-units are an important strategies in the treatment of cancer by the novel molecules. Thus, there is a call for international collaborative researchers to find novel drugs from natural sources. The present study resulted in the contribution by investigating marine bacterial extracts that inhibits cellular NF-κB pathway.
1. Introduction
The marine environment represents a unique resource that enfolds an enormous biological diversity, which will potentially lead to unique biologically active chemical diversity that we can translate into novel biomedicines (Montaser & Luesch, Citation2011).The ocean has a vast diversity of micro-organisms and it is estimated to be more than 10 million species, of which more than 60% are unknown (Jensen & Fenical, Citation1994) and these marine organisms are a prolific source of bioactive and complex secondary metaboliteswith the potential to treat several diseases. Marine natural products have recently been recognized as a promising source of Nuclear factor-kappaB (NF-κB) inhibitors (Haefner, Citation2003; Folmer, Jaspars, Dicato, & Diederich, Citation2008; Folmer, Schumacher et al., Citation2008; Folmer et al., Citation2009). NF-κB is a family of inducible transcription factors found in a variety of cell types and in various marine organisms; the NF-κB include five genes NF-κB 1 (P50/p105), NF-κB 2 (P52/p100), RelA (p65), c-Rel, and RelB that are activated in two major signaling pathways, the canonical and alternative activation pathways (Dolcet, Llobet, Pallares, & Matias-Guiu, Citation2005; Garg & Aggarwal, Citation2002; Haefner, Citation2003; Tsuchiya et al., Citation2015). To function as transcription factors, they assemble as protein dimers, predominantly P65 and P50 in the canonical pathway, and RelB and P52 in the alternative pathway (non-canonical) (Haefner, Citation2003). Moreover, since NF-κB dimers are sustained in the cytoplasm and remain transcriptionally inactive due to their interaction with NF-κB inhibitors (IκBs), they must be activated before they can be translocated to the nucleus (Dolcet et al., Citation2005). The NF-κB signaling pathway has provided insight for pharmacological intervention and deregulation of NF-κB expression is a phenomenon linked to a variety of diseases, including those related to inflammation, asthma, atherosclerosis, AIDS, septic shock, arthritis, and cancer. Hence, NF-κB has become a major target in drug discovery (Garg & Aggarwal, Citation2002; Grivennikov, Greten, & Karin, Citation2010; Golan-Goldhirsh & Gopas, Citation2014; Ozer et al., Citation2009). Remarkably, marine invertebrates and their associated micro-organisms produce secondary metabolites with NF-κB modulatory properties. One potential explanation is that these organisms possess NF-κB or closely related analogs, and therefore, they developed metabolites that can modulate its expression and action (Müller et al., Citation1994; Pancer, Rast, & Davidson, Citation1999; Wang, Tan, Ho, & Ding, Citation2006). Indeed, the NF-κB/IκB signaling cascade is of ancient evolutionary origin. The NF-κB proteins As-rel1 and As-rel2 have been found in ascidians and were shown to be involved in regulating notochord formation and degradation (Shimada, Satoh, & Yokosawa, Citation2001). They were also found in the ancient arthropod Carcinoscorpius rotundicauda (horseshoe crab) (Friedman & Hughes, Citation2002; Wang et al., Citation2006). In addition, it has been shown that sea urchins use a NF-κB analog to protect themselves against apoptosis-inducing compounds released by the diatoms on which they graze and in response to bacterial infection and other pathogens (Pancer et al., Citation1999). Recently, cnidarians such as corals were also shown to possess an NF-κB–like protein (Nv-NF-κB) (Schwarz et al., Citation2008; Sullivan, Kalaitzidis, Gilmore, & Finnerty, Citation2007).
Several bacteria and viruses have been reported to modulate NF-κB activity in host cells to increase their chances of survival as parasites within the host (Hiscott, Kwon, & Génin, Citation2001; Schwarz et al., Citation2008). The discovery of NF-κB in cnidarians and ascidians, both groups of which are known to engage in a variety of symbiotic relationships with marine micro-organisms, led to the hypothesis that NF-κB may be involved in host-symbiont interaction. Moreover, the recent Ectinascidin 743, a marine-derived alkaloid, is approved by the European Commission and US FDA for the treatment of ovarian cancer (Lisowska & Witkowski, Citation2003; Miller et al., Citation2010). This study, therefore, attempts to explore the NF-κB modulatory potential of micro-organisms found in association with marine invertebrates collected from the Red Sea and to assess whether these properties can be used in the modulation of mammalian NF-κB.
2. Results and discussion
2.1. Screening of marine bacteria with NF-κB modulating activity
Samples from the coral Favia sp. collected from the Red Sea stretch of water adjacent to the Interuniversity Institute for Marine Sciences (IUI) in the Gulf of Eilat, Israel and the bacteria associated with the samples were isolated in an axenic culture. The bacterial isolates were extracted and screened for NF-κB modulatory capability using an NF-κB luciferase reporter gene assay. The isolated bacterial extracts had a variable effect on NF-κB activity (Figure ); out of 39 bacterial extracted tested, only one showed significant NF-κB inhibition and two instigated upregulation of NF-κB. The isolate New-33, which exhibited 23% ± 5.37 inhibition of NF-κB, compared to the control and was chosen for further study. Similar screening for marine NF-κB inhibitors was conducted by Folmer et al. (Citation2009) in marine sediment bacteria, 74% inhibited the extracts and 8% induced NF-κB expression at a concentration of 100 μg/ml. After the initial screening, the marine bacteria New-33 isolated from the coral Favia sp. exhibited the highest and most consistent NF-κB inhibitory capability and was therefore selected for further analysis. Though there are many reports of terrestrial microbial products inhibiting NF-κB (Berleth et al., Citation1992; Bremner & Heinrich, Citation2002; Hochstrasser, Citation2006; Rüngeler et al., Citation1999; Sekizawa et al., Citation2002), the study on marine microbial compounds possess NF-κB inhibitory activity is very limited (Folmer, Jaspars, et al., Citation2008 ; Folmer, Schumacher et al., Citation2008, Citation2009)
2.2. New-33 extract modulates NF-κB by alternative pathway
To identify which of the NF-κB subunits undergoes changes following New-33 treatment and to ascertain dose dependence, western blots were performed to detect P65, P50, RelB, and P52 in cytoplasmic and nuclear extracts of treated (New-33 extract or 0.2%, v/v, ethanol) and untreated L428 cells. The results were normalized to actin in the cytoplasmic and nuclear extracts, respectively. All NF-κB subunits were marginally decreased in 0.2% (v/v) of ethanol-treated cells (control) compared to untreated cells. A subsequent comparison of the changes induced by New-33 extract to those observed in the ethanol–treated cells showed a significantly decreased expression of P52 and RelB subunits in both the nuclear and cytoplasmic extracts of treated cells compared to control (Figure (A)). However, no significant change in protein expression amounts of P50 and P65 was detected in the cytoplasmic (Figure (A)) and nuclear fraction (Figure (B)). These results confirm that the New-33 extract inhibits by alternative NF-κB pathway proteins since there is a significant decrease in expression of P52 and RelB.
Figure 2. Detection of NF-kB canonical and alternative pathway subunits in L428 cells following New-33 treatment. Western blot analysis of NF-kB subunits from cytoplasm and nuclei of L428 cells treated with 0.02% MeOH or increasing concentrations of the extract was performed. In order to detect NF-κB nuclear translocation, separate nuclear and cytoplasmic lysates (10 × 10 power 6 cells per sample) were prepared using the NucBuster kit (Novagen). Protein concentrations were estimated using the Bradford method (BioRad). Detection of NF-κB canonical subunits in cytoplasm (A) and nuclei (B).
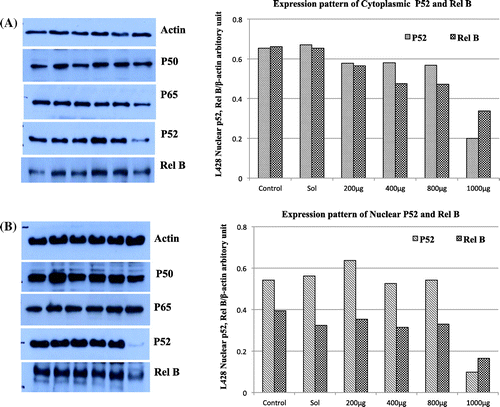
The non-canonical (alternative) NF-κB pathway is an important arm of NF-κB signaling that predominantly targets activation of the P52/RelB NF-κB complex. This pathway depends on the inducible processing of P100, a molecule functioning as both the precursor of P52 and a RelB-specific inhibitor (Sun, Citation2011). To date, there are already several known NF-κB inhibitors of marine origin, and those whose mechanism of action are known only affect the canonical NF-κB activation pathway subunits: P65, P50, or IκBα(Folmer, Jaspars, et al., Citation2008 ; Folmer, Schumacher et al., Citation2008). New-33 is a novel marine bacterium that to the best of our knowledge is the first such marine NF-κB alternative pathway inhibitor discovered. Although the NF-κB signaling cascade is phylogenetically ancient (Friedman & Hughes, Citation2002; Jin et al., Citation2014; Schreiber, Nikolaus, & Hampe, Citation1998; Wang et al., Citation2006), very little is known about its action in marine organisms. Most of the work describing NF-κB signaling in marine invertebrates relies mainly on gene discovery and bioinformatic analysis, and the biological function of NF-κB has only been hypothesized. It has recently been discovered that cnidarians possess an NF-κB–like protein (Nv-NF-κB). Research of this protein from the sea anemone Nematostella vectensis showed that the gene structure and its RHD predicted amino acid sequence were similar to those of the vertebrate NF-κB p50/p52 proteins. In addition, the gene found in the corals Acropora palmate and Montastraea faveolata resembled p105 (Schwarz et al., Citation2008; Sullivan et al., Citation2007).
In vertebrates, NF-κB is known to mediate the immune response to microbial infection. Many bacteria are known NF-κB activators that trigger the innate immune response receptors (TLRs and Nod1/Nod2) to activate the NF-κB signal transduction pathway and the expression of genes encoding pro-inflammatory cytokines (Kelly & Conway, Citation2005; Kravchenko et al., Citation2008). However, some pathogenic bacteria and commensal flora have been shown to attenuate host immune response by interfering with its signal transduction systems (Kelly & Conway, Citation2005). The discovery of NF-κB in cnidarians and ascidians, both of which have symbiotic relationships with marine micro-organisms, supports the idea that it may be involved in host-symbiont interaction (Schwarz et al., Citation2008). Because cnidarian or ascidian hosts is associated with numerous bacteria, it is possible that some of their innate immune responses will not completely eliminate certain bacterial symbionts (Loker, Adema, Zhang, & Kepler, Citation2004).
2.3. The in vitro scratch assay
The in vitro scratch assay, also known as the migration assay, is a model system that enables the effects of cell–matrix and cell–cell interactions on cell migration to be studied and that mimics cell migration during wound healing in vivo Liang, Park, & Guan, Citation2007). When scratched, cell monolayers respond to the disruption of cell–cell contacts via a combination of cell proliferation and migration (Coomber & Gotlieb, Citation1990; Yarrow, Perlman, Westwood, & Mitchison, Citation2004). We performed this assay on MCF-7 human breast cancer cell line and A549 human lung carcinoma cell line to determine whether incubation with New-33extracts affects the ability of these cells to proliferate and migrate. Monolayers of the cell lines were scratched and treated with 0.2%, v/v, ethanol (control) or with New-33 extract at two different concentrations (400 or 800 μg/ml). To track gap closure, the cells were photographed every 24 h and gap widths were measured and compared. The exposure of New-33 extract to MCF-7 cells resulted in varying degrees of invasion and migration inhibition when compared with the control (Figure ). The migration of New-33 extract-treated cells was decreased compared with control group cells, and of particular note, the wound closure was significantly inhibited at higher concentration. Similar result was also observed with A549 cells (Figure ). Our results showed that New-33 extract dramatically reduced both the size of MCF-7 cells at concentration of 800 μg/ml. Observations showed that some cells were detached from the monolayer and some were rounded up. These findings further confirmed the notion that New-33 extract strongly inhibits the proliferation, migration, and invasive capacities of carcinoma cells. Lu and Li (Citation2014) also have reported similar inhibition pattern in MCF-7 cells with salinomycin extracts.New-33 extract’s potential to inhibit growth of A549 and MCF-7 could partially be attributed to its capability to induce apoptosis which is associated with the activation of caspases-3 and -7 as well as DNA fragmentation. As evidenced from the above results, New-33 extract might be an important candidate as a chemo-preventive agent against cancer metastasis.
2.4. Effect of New-33 extract on the cell viability of L428 cells
Most of the marine compounds that have been reported to possess NF-κB inhibitory activity have also been found to have cytotoxic effects (Folmer, Jaspars, et al., Citation2008; Folmer, Schumacher et al., Citation2008). Cytotoxicity test results were displayed in Figure and the results indicate that the New-33 extract was not cytotoxic to the tested L428 cells, as assessed by the tetrazolium-formazan XTT viability assay kit (BeitHaemek). Thus, the New-33 NF-κB inhibitory effect is not due to cell death but only by selective regulation of genes.
2.5. Partial purification of the new-33 extract
In TLC separation of the crude extract, among the different solvent systems trailed, combination of methanol:dichloromethane:distilled water in the ratio of 7:93:10, (v/v) was found to be a suitable solvent system for the separation of components. The bacterial extract showed three bands in under UV illumination (Figure ) with Rf values of 0.88, 0.77, and 0.68, respectively. Out of the three different bands, one fraction with Rf value of 0.77 had significant inhibitory activity towards NF-κB. Moreover, the extract was heat stable and retained 68% of the original activity when heated for 95°C for one minute. The C18 HPLC analyses of the active fraction confirmed the presence of a low molecular weight compound.
2.6. Bacteria sequencing and phylogeny
Quarry of the bacteria sequences in the NCBI megaBLAST revealed that the New-33 16S sequences are similar to the 16S sequences of the Vibrio mediterranei (99%). Goodson et al. (Citation2005) studied the role of symbiotic Vibrio fischeri upregulating NF-κB signaling pathway with microbe-associated molecular patterns (MAMPs) signal in a cephalopod mollusk Euprymna scolopes. There was no study describing the symbiotic association of V. mediterranei with coral Faviasp. However, V. mediterraneiis reported to cause bleaching disease in Oculina patagonica coral (Rubio-Portillo, Yarza, Peñalver, Ramos-Esplá, & Antón, Citation2014). Several bacteria and viruses have been reported to control NF-κB activity in host cells in order to increase their chances to survive as parasites within the host. Pancer et al. (Citation1999) have shown that sea urchins use SpNF-κB a NF-κB analog, to defend against apoptosis-inducing compounds to respond to bacterial infection.
3. Experimental section
3.1. Bacteria cultivation
The mucus samples from coral Favia sp. were collected from the Red Sea stretch of water adjacent to the Interuniversity Institute (IUI) in Eilat (N 29° 30.211′ E 34° 55.068′). Bacteria were isolated via a serial dilution and plating technique as follows: the mucus sample tubes were centrifuged and then diluted 10-fold, after which the samples were spread at concentrations of 10% over marine agar plates (Hi-media Laboratories). The plates were then incubated at 26°C (ambient sea temperature) and sub-cultured to get isolated to axenic cultures.
3.2. Bacteria extraction
During screening for active compounds, each isolate was sampled into 10 ml 10%, w/v, MB and cultivated for three days at 26°C. After cultivation, the bacterial culture was centrifuged at 17,000 rpm for 10 min to obtain two phases, the bacterial pellet and the culture supernatant. The pellet was extracted using a 10-ml mixture of methanol:dichloromethane (1:1, v/v) (protocol adjusted from (Kelman, Kashman, Rosenberg, Kushmaro, & Loya, Citation2006). After 24 h, the extraction phase was transferred to a pre-weighed vials and the solvent is evaporated under low nitrogen stream. The vial containing the dry extract was then weighed again and the amount of dry extract was calculated. Dried extracts were stored at 4°C and then reconstituted with 0.2%, (v/v) ethanol to obtain the desired concentration and used immediately.
3.3. Luciferase reporter gene assay
L428 cells (106 per sample) expressing the luciferase-NF-κB reporter gene inserted upstream of a NF-κB binding consensus sequence were incubated for 2 h in 500 μl of RPMI medium that contained 20 μl of ethanolic bacteria extracts calculated to achieve a final concentration of 60 μg/ml or in 20 μl of 0.2% (v/v) ethanol as control. Cells were then harvested, lysed, and monitored by a luciferase reporter assay kit (Promega) according to the manufacturer’s instructions. Measurements were carried out using a luminometer at 300 nm. Data were normalized to the protein concentration in each lysate as measured by the Bradford method (Bio-Rad). Treated cells were compared to control cells treated with the same volume of the dilution media, 0.2% (v/v) ethanol.
3.4. Western blot
L428 cells (106 per well) were incubated for 2 h in 3.5 ml of RPMI medium containing incremental concentrations (100, 200, 400, and 800 μg/ml).After incubation, the cells were washed three times with PBS and lysed using the NucBuster protein extraction kit (Novagen) according to manufacturer instructions to retain two fractions, cytoplasmic and nuclear. The lysates were kept at −70°C until experimentation. Protein was quantified using the Bradford method (Bio-Rad) and was measured by ELISA at 590 nm. Nuclear and cytoplasmic lysate proteins (50 μg) were separated in 10% SDS-polyacrylamide gel and were then blotted onto nitrocellulose membranes (Scleicher & Schuel). After protein transfer, the membranes were blocked with TBS-T (Tris base- 2.4 gr/L, NaCl- 8 gr/L and TWEEN at pH 7.6) containing 3% milk powder and incubated with appropriate primary antibodies. Subsequently, the membranes were incubated with peroxidase-linked anti-mouse, anti-rabbit, and anti-goat IgG, and protein bands were detected by chemiluminescence with EZ-ECL (Beit- Haemek).
3.5. Migration and invasion assay
Tumor cell migration was analyzed using the Scratch assay. MCF-7 human breast cancer cell line and A549 human lung carcinoma cell line (106) were incubated in 2-ml RPMI or DMEM medium in six-well plates. After a full cell monolayer was obtained, a scratch was made with a sterile tip of a 1-ml pipet and detached cell debris was removed by replacing the growth medium. The cells were then treated with 0.2% (v/v) ethanol as control, or with 400 and 800 μg/ml of the bacterial extracts. The plates were then immediately photographed to establish time 0 images and were photographed again at 24 and 48 h.
3.6. Cell viability assay
Cell survival was measured by a tetrazolium-formazan XTT assay kit (BeitHaemek) based on the color reaction generated by the reduction of tetrazolium salts into colored formazan compounds by the mitochondria in the live cells. L428 cells (1 × 104 per 100 μl RPMI) were incubated for 144 h at 37°C in 96-well plates with incremental concentrations (100, 200, 400 and 800 μg/ml) of bacterial extract. After incubation, 25 μl of XTT solution was added, and the plates were incubated again for 4 h at 37°C. Absorbance was read at 450 nm by an ELISA reader. Cell viability was calculated as percent of control cells treated by the dilution media, 0.2%, (v/v) ethanol.
3.7. Separation of bioactive compound by TLC and HPLC analysis
The separation of crude extract was carried out by Thin layer chromatography (TLC) C18-silica TLC plate and with different combinations of Methanol, DCM, DMSO, chloroform, ethyl acetate, and acetone as solvent system. The different separated compound bands in the silica gel plate were marked under UV and the fractions were carefully collected in separate sterile vials. The separated compounds were re-extracted with acetonitrile, dried under nitrogen stream and dissolved in 0.2% (v/v) ethanol and checked for its efficacy in inhibition of NF-κB in L428 cells.
Analysis of active compounds from TLC was performed on an Agilent 1100 series Rapid Resolution LC (Agilent Technologies, Santa Clara, CA) consisting an auto-sampler G 1329A and 1200 series with Frizzier G1330B FC/ALS Therm. This instrument was equipped with Kromasil 5U 100A C18 column (2.0 × 250 mm, 5 μm). Acetonitrile and formic acid in water (0.1% Formic acid, v/v) were used as mobile phases A and B, respectively. The gradient was programmed as follows: 0 min, 35% A; 5 min, 35% A; 38 min, 95% A; 40 min, 95% B; 45 min, 100% B; 50 min, 100% B. The flow from the HPLC system into the Ion Trap MS Esquire detector 3000 Plus (BrukerDaltonics, Inc., MA, USA) was 0.25 ml/min and the auto-sampler was operated at 4°C. The injection volume was 20 μl and the column temperature was maintained at 30°C.
3.8. Bacterial sequencing and phylogeny
Bacterial DNA of New33 strain was extracted using an UltraClean Microbial DNA Isolation kit (MoBio Laboratories, Solana Beach, CA). Nearly complete genomic 16S rRNA was amplified by PCR with a Mastercycler gradient thermocycler (Eppendorf, Westbury, NY) using the universal forward and reverse primers 8F and 1512R, respectively. An initial 4 min denaturation step at 95°C was followed by 30 cycles of the following program: 30s at 94°C, 40 s at 54°C, 70 s at 72°C, and a final extension step at 72°C for 20 min concluded the reaction. The PCR products, with sizes of approximately 1,500 bp, were separated by electrophoresis on a 0.8% agarose gel. Sequencing was performed using the ABI PRISM dye terminator cycle sequencing ready reaction kit with AmpliTaq DNA polymerase FS and an ABI model 373A DNA sequencer (Perkin-Elmer). 16S rRNA gene sequences were aligned with the best-matched relative sequences in the GenBank database using the basic alignment nucleotide search tool (BLAST) at the National Center for Biotechnology Information (NCBI) website.
4. Conclusions
The present study led to the conclusion that coral-associated bacteria New-33 is capable of inhibiting NF-κB by the non-canonical pathway without being cytotoxic. This specific modulation of the NF-κB alternative pathway may have therapeutic implications of NF-κB-associated diseases and potential to inhibit carcinogenesis. These findings await an in-depth analysis on the purification and identification of the active compounds, with an emphasis on elucidating the mechanism of action.
Funding
This work was supported ISF Grant 1169/07, The Israel Cancer Association through the Miriam and Shlomo Hasid Memorial fund, The Genetics and Cancer Goldyne Savad Estate, Ben Gurion University and The Richard H. Holzer Foundation.
Acknowledgments
The PBC research fellowship extended by the Planning and Budgeting Committee (PBC) of the Council for Higher Education in Israel, to E.O and NN is gratefully acknowledged. We gratefully acknowledge Hila Ben Hamo and Shimrit Laor for their help in collecting the samples from Red sea.
Additional information
Notes on contributors
Ariel Kushmaro
Ariel Kushmaro’s research activities focus on the development of various techniques for environmental sensing, bioremediation, and environmental microbiology. His research aimed at understanding the structure and function of microbial communities and their dynamics with regard to the environment. These investigations include environmental monitoring of various pollutants, the isolation of bacteria, and their study under defined conditions in the laboratory. This includes characterization of the enrichment and pure cultures by nucleic acid analysis. Research projects include wastewater treatment and bioremediation, microbial encapsulation technology, discovery of novel antibiotics from cultured and uncultured micro-organisms.
References
- Berleth, E. S., Kasperek, E. M., Grill, S. P., Braunscheidel, J. A., Graziani, L. A., & Pickart, C. M. (1992). Inhibition of ubiqutin-protein ligase (E3) by mono functional and bifunctional phenylarsenoxides - evidence for essential vicinal thiols and a proximal nuclephile. Journal of Biological Chemistry, 267, 16403–16411.
- Bremner, P., & Heinrich, M. (2002). Natural products as targeted modulators of the nuclear factor-kappa B pathway. Journal of Pharmacy and Pharmacology, 54, 453–472.10.1211/0022357021778637
- Coomber, B. L., & Gotlieb, A. I. (1990). In vitro endothelial wound repair. Interaction of cell migration and proliferation. Arteriosclerosis, 10, 215–222.10.1161/01.ATV.10.2.215
- Dolcet, X., Llobet, D., Pallares, J., & Matias-Guiu, X. (2005). NF-kB in development and progression of human cancer. Virchows Archiv, 446, 475–482. doi:10.1007/s00428-005-1264-9
- Folmer, F., Jaspars, M., Dicato, M., & Diederich, M. (2008). Marine natural products as targeted modulators of the transcription factor NF-kappaB. Biochemical Pharmacology, 75, 603–617. doi:10.1016/j.bcp.2007.07.044
- Folmer, F., Jaspars, M., Solano, G., Cristofanon, S., Henry, E., Tabudravu, J., … Diederich, M. (2009). The inhibition of TNF-alpha-induced NF-kappaB activation by marine natural products. Biochemical Pharmacology, 78, 592–606. doi:10.1016/j.bcp.2009.05.009
- Folmer, F., Schumacher, M., Diederich, M., & Jaspars, M. (2008). Finding NEMO (inhibitors) the search for marine pharmacophores targeting the nuclear factor-kappa B. Chimica Oggi-Chemistry Today, 26, 40–46.
- Friedman, R., & Hughes, A. L. (2002). Molecular evolution of the NF-kappa B signaling system. Immuno Genetics, 53, 964–974. doi:10.1007/s00251-001-0399-3
- Garg, A., & Aggarwal, B. B. (2002). Nuclear transcription factor-kappa B as a target for cancer drug development. Leukemia, 16, 1053–1068. doi:10.1038/sj/leu/2402482
- Golan-Goldhirsh, A., & Gopas, J. (2014). Plant derived inhibitors of NF-kB. Phytochemistry Reviews, 13, 107–121. doi:10.1007/s11101-013-9293-5
- Goodson, M. S., Kojadinovic, M., Troll, J. V., Scheetz, T. E., Casavant, T. L., Soares, M. B., & McFall-Ngai, M. J. (2005). Identifying Components of the NF-κB Pathway in the Beneficial Euprymna scolopes-Vibrio fischeri Light Organ Symbiosis. Applied and Environmental Microbiology, 71, 6934–6946.10.1128/AEM.71.11.6934-6946.2005
- Grivennikov, S. I., Greten, F. R., & Karin, M. (2010). Immunity, inflammation, and cancer. Cell, 140, 883–899. doi:10.1016/j.cell.2010.01.025
- Haefner, B. (2003). Drugs from the deep: marine natural products as drug candidates. Drug Discovery Today, 8, 536–544. doi:10.1016/S1359-6446(03)02713-2
- Hiscott, J., Kwon, H., & Genin, P. (2001). Hostile takeovers: viral appropriation of the NF-kappa B pathway. Journal of Clinical Investigation, 107, 143–151.10.1172/JCI11918
- Hochstrasser, M. (2006). Lingering mysteries of ubiquitin-chain assembly. Cell, 124, 27–34.10.1016/j.cell.2005.12.025
- Jensen, P. R., & Fenical, W. (1994). Strategies for the discovery of secondary metabolites from marine bacteria: Ecological perspectives. Annual Review of Microbiology, 48, 559–584.10.1146/annurev.mi.48.100194.003015
- Jin, J., Hu, H., Li, H. S., Yu, J., Xiao, Y., Brittain, G. C., … Sun, S. C. (2014). Noncanonical NF-kappa B Pathway Controls the Production of Type I Interferons in Antiviral Innate Immunity. Immunity, 40, 342–354.10.1016/j.immuni.2014.02.006
- Kelly, D., & Conway, S. (2005). Bacterial modulation of mucosal innate immunity. Molecular Immunology, 42, 895–901. doi:10.1016/j.molimn.2004.12.003
- Kelman, D., Kashman, Y., Rosenberg, E., Kushmaro, A., & Loya, Y. (2006). Antimicrobial activity of Red Sea corals. Marine Biology, 149, 357–363.10.1007/s00227-005-0218-8
- Kravchenko, V. V., Kaufmann, G. F., Mathison, J. C., Scott, D. A., Katz, A. Z., Grauer, D. C., … Ulevitch, R. J. (2008). Modulation of gene expression via disruption of NF-kappa B signaling by a bacterial small molecule. Science, 321, 259–263. doi:10.1126/science.1156499
- Liang, C. C., Park, A. Y., & Guan, J. L. (2007). In vitro scratch assay: a convenient and inexpensive method for analysis of cell migration in vitro. Nature Protocols, 2, 329–333. doi:10.1038/nprot.2007.30
- Lisowska, K., & Witkowski, J. M. (2003). Viral strategies in modulation of NF-kappa B activity. Archivum Immunologiae Et Therapiae Experimentalis, 51, 367–375.
- Loker, E. S., Adema, C. M., Zhang, S. M., & Kepler, T. B. (2004). Invertebrate immune systems–not homogeneous, not simple, not well understood. Immunological Reviews, 198, 10–24.10.1111/imr.2004.198.issue-1
- Lu, W., & Li, Y. (2014). Salinomycin Suppresses LRP6 Expression and Inhibits Both Wnt/beta-catenin and mTORC1 Signaling in Breast and Prostate Cancer Cells. Journal of Cellular Biochemistry, 115, 1799–1807.10.1002/jcb.24850
- Miller, S. C., Huang, R., Sakamuru, S., Shukla, S. J., Attene-Ramos, M. S., Shinn, P., … Xia, M. (2010). Identification of known drugs that act as inhibitors of NF-kappa B signaling and their mechanism of action. Biochemical Pharmacology, 79, 1272–1280.10.1016/j.bcp.2009.12.021
- Montaser, R., & Luesch, H. (2011). Marine natural products: A new wave of drugs? Future Medicinal Chemistry, 3, 1475–1489.10.4155/fmc.11.118
- Müller, W. E. G., Gamulin, V., Rinkevich, B., Spreitzer, I., Weinblum, D., & Schroder, H. C. (1994). Ubiquitin and ubiquitination in cells from the marine sponge geodia cydonium. Biological Chemistry Hoppe-Seyler, 375, 53–60.10.1515/bchm3.1994.375.1.53
- Ozer, J., Eisner, N., Ostrozhenkova, E., Bacher, A., Eisenreich, W., Benharroch, D., … Gopas, J. (2009). Nuphar lutea thioalkaloids inhibit the nuclear factor kappaB pathway, potentiate apoptosis and are synergistic with cisplatin and etoposide. Cancer Biology & Therapy, 8, 1860–1868. doi:10.4161/cbt.8.19.9567
- Pancer, Z., Rast, J. P., & Davidson, E. H. (1999). Origins of immunity: transcription factors and homologues of effector genes of the vertebrate immune system expressed in sea urchin coelomocytes. Immuno genetics, 49, 773–786.10.1007/s002510050551
- Rubio-Portillo, E., Yarza, P., Penalver, C., Ramos-Espla, A. A., & Anton, J. (2014). New insights into Oculinapatagonica coral diseases and their associated Vibrio spp. communities. The ISME Journal, 8, 1794–1807.10.1038/ismej.2014.33
- Rüngeler, P., Castro, V., Mora, G., Gören, N., Vichnewski, W., Pahl, H. L., … Schmidt, T. J. (1999). Inhibition of transcription factor NF-kappa B by sesquiterpene lactones: a proposed molecular mechanism of action. Bioorganic & Medicinal Chemistry, 7, 2343–2352.10.1016/S0968-0896(99)00195-9
- Schreiber, S., Nikolaus, S., & Hampe, J. (1998). Activation of nuclear factor kappa B in inflammatory bowel disease. Gut, 42, 477–484.10.1136/gut.42.4.477
- Schwarz, J. A., Brokstein, P. B., Voolstra, C., Terry, A. Y., Miller, D. J., Szmant, A. M., … Medina, M. (2008). Coral life history and symbiosis: Functional genomic resources for two reef building Caribbean corals, Acroporapalmata and Montastraeafaveolata. Bmc Genomics, 9, 1–16. doi:10.1186/1471-2164-9-97
- Sekizawa, R., Ikeno, S., Nakamura, H., Naganawa, H., Matsui, S., Iinuma, H., & Takeuchi, T. (2002). Panepophenanthrin, from a Mushroom Strain, a Novel Inhibitor of the Ubiquitin-Activating Enzyme. Journal of Natural Products, 65, 1491–1493.10.1021/np020098q
- Shimada, M., Satoh, N., & Yokosawa, H. (2001). Involvement of Rel/NF-kappa B in regulation of ascidian notochord formation. Development Growth & Differentiation, 43, 145–154.10.1046/j.1440-169X.2001.00560.x
- Sullivan, J. C., Kalaitzidis, D., Gilmore, T. D., & Finnerty, J. R. (2007). Rel homology domain-containing transcription factors in the cnidarian Nematostella vectensis. Development Genes and Evolution, 217, 63–72. doi:10.1007/s00427-006-0111-6
- Sun, S. C. (2011). Non-canonical NF-kappa B signaling pathway. Cell Research, 21, 71–85.10.1038/cr.2010.177
- Tsuchiya, R., Tanaka, T., Hozumi, Y., Nakano, T., Okada, M., Topham, M. K., … Goto, K. (2015). Down regulation of diacylglycerol kinase ζ enhances activation of cytokine-induced NF-κB signaling pathway. Biochimicaet Biophysica Acta (BBA)-Molecular Cell Research, 1853, 361–369.
- Wang, X. W., Tan, N. S., Ho, B., & Ding, J. L. (2006). Evidence for the ancient origin of the NF-kappa B/I kappa B cascade: Its archaic role in pathogen infection and immunity. Proceedings of the National Academy of Sciences of the United States of America, 103, 4204–4209. doi:10.1073/pnas.0507044103
- Yarrow, J. C., Perlman, Z. E., Westwood, N. J., & Mitchison, T. J. (2004). A high-throughput cell migration assay using scratch wound healing, a comparison of image-based readout methods. Bmc Biotechnology, 4, 1–9. doi:10.1186/1472-6750-4-21