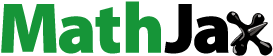
Abstract
The aim of this study was to evaluate the levels of selected heavy metals/metalloids in filling station dust from the Kumasi Metropolis, Ghana. A total of forty (40) dust samples were analysed for Fe, Ti, Zn, Zr, Mn, Sr, Ba, Cr, Pd, Ni, Cu, As and Mo using X-ray Fluorescence technique. Mean concentrations of Ba, As, Cr, Cu, Fe, Mn, Mo, Ni, Pb, Sr, Ti, Zn and Zr were 92.26, 6.20, 70.41, 50.18, 466.22, 163.68, 4.63, 44.05, 46.93, 106.69, 327.51, 280.32 and 182.05 mg/kg, respectively. The pollution index (PI) and geo-accumulation (Igeo) index values were in the order of Ba < Mn < Sr < Zr < Cu < Cr < Ni < Mo < As < Zn < Pb < Fe < Ti. The pollution load index had a mean of 2.20, signifying moderate pollution. Higher PI and Igeo value for Pb, Fe and Ti indicated high pollution. The PCA analysis identified anthropogenic inputs and natural origin as the main sources of pollution in filling station dust. The potential ecological risk index decreased as follows: As > Pb > Ni > Cu > Cr > Zn > Mn > Ba. The contribution of hazard quotient via ingestion for most of the heavy metals/metalloids were high with 11.83% for adults and 88.17% for children. For health risk assessment, non-carcinogenic values were below the threshold values, except hazard index via ingestion. The main exposure pathway for both children and adults was ingestion, followed by dermal contact and inhalation.
Public Interest Statement
The study looks at the possibility of dust blown from a fuel station causing harm to the health of humans. The likely groups of people to this potential harm are fuel station workers, patrons and residents within the vicinity of these stations. The presence of toxic chemicals in spilled fuel, oils, lubricants and exhaust emissions from vehicles that plough the station have the tendency to pollute the dust in and around these stations. This study gives an overview of the potential health implications of fuel stations on the general public if they come into contact with polluted dust.
Competing Interests
The authors declare no competing interest.
1. Introduction
Atmospheric pollution constitutes a major challenge in several countries, especially those under rapid development (He, Yun, Shi, & Jiang, Citation2013; Schleicher et al., Citation2011; Teng et al., Citation2014). During the combustion of wood and fossil fuels, waste incineration, high-temperature industrial processes and traffic, dust containing trace metals are released into the atmosphere (Allen, Nemitz, Shi, Harrison, & Greenwood, Citation2001). Dust particulates can affect human health (Ruiz-Jimenez et al., Citation2012), especially the presence of trace metals, which are noxious to humans via inhalation or ingestion (Khairy, Barakat, Mostafa, & Wade, Citation2011; Lu, Zhang, Li, & Chen, Citation2014).
Heavy metals/metalloids pollution in dust is a problem due to their non-biodegradability, wide occurrence, toxicity, as well as their ability to accumulate over time (Dong, Yu, Bian, Wang, & Di, Citation2011). Heavy metals, including Ni, Cd, Pb, Zn, Cu, Hg, Cr and others refer to metals with densities > 5 g/cm3 (Li, Ma, van der Kuijp, Yuan, & Huang, Citation2014). As a result of the similarities in chemical properties and fate in the environmental, arsenic is frequently referred to as a heavy metal (Huamain, Chunrong, Cong, & Yongguan, Citation1999). Heavy metals/metalloids pollution in dust is an irreversible process and very difficult to remove once it occurs (Zhou & Song, Citation2004). They also have the potential to cause biomagnification and bioaccumulation in the ecosystem (Manahan, Citation2000). Improper disposal of engine oil, brake fluid, transmission oil and leaded gasoline around the vicinity of fuel contributes to the heavy metals/metalloids load (Dauda & Odoh, Citation2012; Khorshid & Thiele-Bruhn, Citation2016; Luo, Yu, & Zhu, Citation2012; Pant & Harrison, Citation2013). Heavy metals/metalloids in dust can affect human health when exposed via ingestion, inhalation and dermal contact (Ling, Shen, Gao, Gu, & Yang, Citation2008; McLaughlin, Hamon, McLaren, Speir, & Rogers, Citation2000). For example, extreme exposure to Pb can harm the skeletal, nervous, endocrine, circulatory, immune and enzymatic systems (Zhang et al., Citation2012). In addition, exposure to Cd can cause pulmonary adenocarcinomas, hypertension, kidney dysfunction, lung cancer, prostatic proliferative lesions and bone fractures, while As exposure can cause dermal lesions, skin cancer, peripheral vascular disease and peripheral neuropathy (Chen, Teng, Lu, Wang, & Wang, Citation2015).
Source identification of heavy metals/metalloids is crucial for effective dust remediation and pollution control (Guo, Huo, Xi, Zhang, & Wu, Citation2015). To check the potential risk of metals, assessment tools including enrichment factor (EF), geo-accumulation index (Igeo), potential ecological risk index (PER), pollution load index (PLI) and human health risk assessment are used to measure the pollution level (Ma, Yang, Li, & Wang, Citation2016). Multivariate statistical methods have also been used to access the different sources of metals in the dust (Ma et al., Citation2016).
In relation to several studies, heavy metals/metalloids pollution in the environment mostly originate from anthropogenic sources (Wei & Yang, Citation2010). In urban road dusts, the anthropogenic sources of heavy metals/metalloids include industrial emission (chemical plant, power plants, metallurgical industry, coal combustion, auto repair shop, etc.), traffic emission (brake lining wear particles, weathered street surface particles, vehicle exhaust particles, tyre wear particles), pavement surface, weathering of building and domestic emission (Ahmed & Ishiga, Citation2006; Banerjee, Citation2003; Sezgin, Ozcan, Demir, Nemlioglu, & Bayat, Citation2004). Lately, dust is assessed as an analytical model with implications on environmental and human health (Shen et al., Citation2017; Ying, Shaogang, & Xiaoyang, Citation2016). Therefore, a number of studies on human health risk evaluation of heavy metals/metalloids pollutions in dust are reported (Han, Gao, Wei, Xu, & Gao, Citation2016; Lin, Fang, Wang, & Xu, Citation2015; Qing, Yutong, & Shenggao, Citation2015). Studies on human health risk assessment of heavy metals/metalloids in Ghana have largely focused on food, cosmetic product, soil, wastewater, ground and surface water contamination (Agorku, Kwaansa-Ansah, Voegborlo, Amegbletor, & Opoku, Citation2016; Akoto, Ephraim, & Darko, Citation2008, 2009; Asare-Donkor, Kwaansa-Ansah, Opoku, & Adimado, Citation2015; Atiemo et al., Citation2011; Boateng, Opoku, Acquaah, & Akoto, Citation2015; Eze, Udeigwe, & Stietiya, Citation2010; Guo et al., Citation2015; Kwaansa-Ansah, Agorku, & Nriagu, Citation2011; Nkansah, Opoku, & Ackumey, Citation2016; Obiri, Citation2007).
In the past decade, several studies on dust have been conducted on metal concentrations, source identification and distribution (Glorennec, Lucas, Mandin, & Le Bot, Citation2012; Laidlaw & Taylor, Citation2011; Lu et al., Citation2010). While there have been some recent studies on road and street dust (Duong & Lee, Citation2011; Li, Poon, & Liu, Citation2001), very few studies have been reported in dusts around oil filling stations (Afrifa et al., Citation2013; Dauda & Odoh, Citation2012; Ekperusi & Aigbodion, Citation2015; Emmanuel, Cobbina, Adomako, Duwiejuah, & Asare, Citation2014). Despite these serious effects of trace metals on human health, particularly for children and adults, information about pollution levels and health risks of heavy metals/metalloids in the dust around fuel filling stations in Kumasi metropolis are lacking. The metropolis houses several types of highly polluting filling station industries. Thus, the Kumasi metropolis is facing severe threats from contamination induced by the fast growth of filling station activities that adversely can influence human health in the metropolis. This study, therefore, aims: (1) to measure the concentration of Fe, Ti, Zn, Zr, Mn, Sr, Ba, Cr, Pd, Ni, Cu, As and Mo, (2) to ascertain the possible sources and their relationship using multivariate analysis and (3) to evaluate the health risk and pollution levels of heavy metals/ metalloids in dust around the vicinity of fuel filling stations from Kumasi Metropolis.
2. Materials and methods
2.1. Study area
Sampling was done from two filling stations in each of the twenty (20) sub-metros in Kumasi. The Metropolis of Kumasi is centrally situated in the Ashanti Region, Ghana with about 270 km North of Accra. Kumasi lies between longitude 1.30°–1.35° and latitude 6.35°– 6.40° with an elevation above sea level of 250–300 metres. The wet sub-equatorial region is the area where Kumasi Metropolis has a mean maximum and minimum temperature of 30.7 and 21.5°C, respectively. The average humidity is about 84.16 and 60.00%, respectively (Ghana Statistical Services, Citation2010). The double maxima rainfall regime of Kumasi (214.3 mm in June and 165.2 mm in September) has a direct effect on the environment and population growth (Ghana Statistical Services, Citation2010). The major rainy season occurs between March/April to July and September to October as the minor rainy season. Furthermore, between November and early February, Kumasi experiences dry season, which affects small streams to dry up. The land area of the Metropolis is about 254 square kilometres. Kumasi is accessible from all zones of the country due to its unique central position. The administrative capital of Ashanti Region is Kumasi, which is the second largest city of Ghana. Kumasi is a rapidly developing metropolis with about two million people and annual growth rate of more than 5.4%. The physical structure of Kumasi is global with a centrally located area for commercial activities, such as the Adum shopping centre, the central market and Kejetia Lorry Park. In addition, there are satellite markets, such as Oforikrom market, Asafo market, Atonsu market and Bantama market in the metropolis. Other economic activities include the Anloga wood market, the Kaase/Asokwa industrial area and Suame Magazine. Most industries, which deal in soap making, logging and food processing, are located at Kaase and Asokwa industrial area. According to the Ghana Statistical Service, about 60, 46 and 48% of the Metropolis are rural, peri-urban and urban, respectively, confirming the rapid rate of urbanisation (Ghana Statistical Services, Citation2010).
2.2. Sampling
Twenty fuel filling stations were chosen randomly, from each of the 20 sub-metros for study. At the sampling site, the site was zoned out into five parts, namely, the four corners and the centre. The GPS coordinates of all the sampling sites were taken and are presented in Figure .
Dust samples were sampled from surfaces by sweeping. The dust samples were gathered from these zones into brown paper envelopes using soft touch brush and plastic dustpan. The paper envelopes were labelled according to the name of the filling station and the sub-metro. Three samples were then sampled from the Kwame Nkrumah University of Science and Technology botanical gardens to serve as pristine samples since the dust location is devoid of any contamination from vehicular activity.
2.3. Sample preparation
The dust samples were dried at room temperature. The samples were then sieved with a mesh of pore size of 60 and aperture of 20 micrometres. As a measure of avoiding cross-contamination, the sieves were washed intermittently after each sieving. The sieved samples were homogenised and ground with mortar and pestle and then kept in a desiccator prior to analysis.
2.4. Sample analysis
The heavy metals/metalloids content in the dust samples was determined with Thermo Scientific Niton X-ray Fluorescence (XRF) analyser (NDTr-XL3t-86956). The sample holder was filled halfway with the digested sample and covered with a Mylar film. The cupped sample was then placed in the XRF shroud and scanned for 180 s to obtain the desired result. All the samples were treated in the same manner. All the XRF sample analysis was done in triplicate. The equipment was calibrated with reference material of OC USGS SAR-M 180-673. Recovery rates in the range of 90 and 116% were sufficient for analysis. The limits of detection for As, Cr, Ba, Fe, Cu, Mn, Mo, Pb, Sr, Ni, Ti, Zr and Zn were 0.01, 0.05, 0.03, 0.82, 0.28, 0.70, 0.56, 1.60, 0.23, 0.35, 0.64, 1.27 and 0.15 mg/kg, respectively; while limits of quantification were 0.04, 3.24, 0.52, 0.58, 2.13, 0.95, 2.62, 5.12, 3.53, 0.86, 1.56, 0.58 and 4.07 mg/kg, respectively.
2.5. Evaluation of heavy metals/metalloids contamination
Pollution levels of heavy metals/metalloids in filling station dust were investigated using PER, Igeo and PI (Pan et al., Citation2016; Ying et al., Citation2016). Geo-accumulation index was initially applied to river sediments and has also been used for the evaluation of dust pollution (Salati & Moore, Citation2010). Geo-accumulation index allows the assessment of environmental contamination between pre-industrial and current concentrations. In this study, Igeo was evaluated following the Muller (Citation1969) equation:(1)
(1)
where Bn is geochemical background value in dust and Cn is the estimated concentration of heavy metals/metalloids n. In this study, the background geochemical compositions by Taylor and McLennan (Citation1995) were used as the background values for calculating the Igeo values. The constant 1.5 allows us to analyse the natural variations in the concentration of a given substance in the environment and to detect small anthropogenic effects (Wei & Yang, Citation2010). The Igeo classification is given in Table (Chen et al., Citation2005).
Table 1. Potential ecological risks, risk category indices and the related classifications of heavy metals/metalloids pollution
The pollution index was the concentration of heavy metals/metalloids in the filling station dust relative to the background concentration of the equivalent heavy metals/metalloids (Kamani et al., Citation2015). To evaluate the pollution status of the filling station dust samples, the PLI of each heavy metals/metalloids was calculated according to Equation (Equation2(2)
(2) ):
(2)
(2)
The PLI classification of heavy metals/metalloids contamination is given in Table (Islam, Ahmed, & Habibullah-Al-Mamun, Citation2015).
2.6. Potential ecological risk
To access the heavy metals/metalloids toxicity, the PER method by Hakanson (Citation1980) was used to estimate their level of pollution in filling station dust. The PER was evaluated according to Equations (Equation3(3)
(3) )–(Equation5
(5)
(5) ):
(3)
(3)
(4)
(4)
(5)
(5)
where , Ci,
,
and
represent the pollution factor, estimated concentration, background concentration, PER index and toxic response factors for heavy metals/metalloids n, respectively. The toxic response factor for Cu, Zn, Pb, Mn, Ni, Cr, As and Ba were 5, 5, 1, 1, 5, 2, 10 and 1, respectively (Hakanson, Citation1980).
As summarised in Table , the and PER classifications defined by Hakanson (Citation1980) were used in this study.
2.7. Human health risk assessment
Human health risk assessment involves the evaluation of possible human health effect in the contaminated environmental media (Li et al., Citation2014). The carcinogenic and non-carcinogenic risks through dermal contact, ingestion and inhalation exposure pathways were evaluated using the human health risk assessment (Qing et al., Citation2015).
2.8. Exposure assessment
The health risk assessment is centred on the exposure factors and guidelines handbook of United States Environmental Protection Agency (USEPA, Citation2002, Citation2003). The average daily dose (ADD) via inhalation (ADDinh), ingestion (ADDing) and dermal contact (ADDderm) for both children and adults were evaluated following Equations (Equation6(6)
(6) ) and (Equation7
(7)
(7) ):
(6)
(6)
(7)
(7)
(8)
(8)
The exposure factors and values (Ying et al., Citation2016) used in the risk assessment are given in Table .
Table 2. Exposure factors and reference value of parameters used for the human health risk evaluation of heavy metals/metalloids in filling station dust
2.9. Non-carcinogenic risk assessment
The carcinogenic and non-carcinogenic adverse effects were evaluated with the hazard quotient (HQ), carcinogenic risk (RI) and hazard index (HI) approaches (Ying et al., Citation2016). According to (USEPA, Citation1989), the hazard quotient is the average daily dose of heavy metals/metalloids with reference to its reference dose (RfD):(9)
(9)
If HQ < 1, signifies no adverse effects, whereas HQ > 1, signifies adverse effects (USEPA, Citation2011).
To assess the overall adverse effects of non-carcinogenic risk, the hazard index approach was applied (USEPA, Citation1986). The HI is the sum of HQ through the three exposure pathways for heavy metals/metalloids. For a mixture of contaminations, the HI was evaluated according to Equation (Equation10(10)
(10) ) (USEPA, Citation1989):
(10)
(10)
HI < 1 denotes non-carcinogenic effects, whereas HI > 1 signifies adverse effects.
2.10. Carcinogenic risk assessment
The carcinogenic risk is the possibility of an individual to develop cancer during the lifetime exposure to the carcinogenic threats (Li et al., Citation2014). According to (USEPA, Citation1989), the slope factor (SF) directly convert the ADD of contaminant exposed over a lifetime risk of a cancer patient:
The values for SF in mg/kg day, RfD and other calculated parameters are presented in Table .
Table 3. The toxicity response to heavy metals/metalloids as the oral slope factor and oral reference dose
Risk value < 10−6 represents no carcinogenic risk to health from the dust, while a risk value >1 × 10−4 denotes high risk of developing cancer. A risk value ranging from 1 × 10−6–1 × 10−4 signify an acceptable risk to human health (Hu et al., Citation2012).
2.11. Statistical analysis
Descriptive statistics and multivariate analysis were calculated using IBM Statistical Package for the Social Sciences (SPSS) version 20. The normality tests were analysed using the Shapiro–Wilk test. The Shapiro–Wilk test is based on the correlation between the heavy metals/metalloids concentration and the corresponding normal scores (Peat & Barton, Citation2008) and offers better power than the Kolmogorov–Smirnov test even after the Lilliefors correction (Steinskog, Tjøstheim, & Kvamstø, Citation2007). Principal component and Pearson’s correlation analysis were used to evaluate and ascertain the possible source of heavy metals/metalloids contamination in the filling station dust. According to Lee, Qin and Lee (Citation2006), the principal component is transformed from the original variable via eigen analysis. Herein, varimax rotation with Kaiser normalisation was employed to extract components with eigenvalue >1.
3. Results and discussion
3.1. Heavy metals/metalloids concentration in filling station dust
The descriptive statistics of heavy metals/metalloids concentration in the filling station dust sampled from the Kumasi Metropolis are presented in Table .
Table 4. Descriptive data of heavy metals/metalloids concentration (mg/kg) in filling station dust collected from the Kumasi Metropolis and reference value (Taylor & McLennan, Citation1995)
The filling station dust exhibited distinct variations in the levels of heavy metals/metalloids; with concentration ranges of 2.58–13.28, 33.72–142.63, 21.81–150.04, 89.85–681.87, 14.55–248.58, 2.77–8.93, 56.08–327.06, 6.48–129.87, 9.42–183.94, 347.00–585.53, 12.95–520.68, 21.30–59.91 and 92.02–250.38 mg/kg, for As, Ba, Cr, Fe, Cu, Mo, Mn, Pb, Sr, Ti, Ni, Zn and Zr, respectively. The mean concentrations of As, Ba, Cr, Fe, Cu, Mn, Mo, Pb, Sr, Ti, Zn, Ni and Zr were 6.20, 92.26, 70.41, 466.22, 50.18, 163.68, 4.63, 46.93, 106.69, 327.51, 280.32, 44.05, and 182.05 mg/kg, respectively. The mean concentrations of As, Cr, Cu, Fe, Mn, Mo, Pd, Ti, Zn and Zr were above the corresponding reference value and this indicates pollution of these metals in the filling station dust. Considering the mean concentration, the heavy metals/metalloids in the filling station dust were in the increasing order: Fe > Ti > Zn > Zr > Mn > Sr > Ba > Cr > Cu > Pd > Ni > As > Mo. The normality distributions of heavy metals/metalloids concentrations were checked by the one-sample Shapiro–Wilk normal test. The results showed that the concentrations of Fe, Ti, Zn, Zr, Mn, Sr, Ba, Cr, Cu, Pd, Ni and Mo were normally distributed (p > 0.05) in the collected filling station dust (Wang, Markert, Chen, Peng, & Ouyang, Citation2012), while As concentration showed non-normal distribution due to (p < 0.05) (Zhou et al., Citation2014). According to Karimi Nezhad, Tabatabaii and Gholami (Citation2015), the coefficient of variation, which shows the variability degree of heavy metals/metalloids concentration are classified as low variability with coefficient of variation ≤20%, moderate variability (21% < coefficient of variation ≤ 50%) and high variability (50% < coefficient of variation ≤ 100%) (Qing et al., Citation2015). Based on this classification, the heavy metals/metalloids in the filling station dusts decreases as: Cu (99.74%) > Pb (68.68%) > Cr (52.24%) > As (43.87%) > Zn (42.74%) > Ba (41.66%) > Mn (40.15%) > Ni (36.98%) > Mo (36.07%) > Sr (34.74%) > Zr (22.18%) > Ti (7.33%) > Fe (6.75%). The coefficient of variation of Pb and Cr specified a moderate degree of variability, which reveals no homogeneous levels. A large coefficient of variation of >70% was found for Cu, an indication that Cu concentration differed greatly in the study region. The skewness values of Cu, As, Mo and Pb were >1, indicated that they were positively skewed towards low concentration. Moreover, while the negative kurtosis values of Ba, Cr, Ni, Sr, Zn and Zr, indicated that their distribution in the filling station dust was less steep than normal (Chen, Lu, & Yang, Citation2012).
3.2. Comparison with other heavy metals/metalloids dust studies
In order to compare the examined heavy metals/metalloids concentration with studies from other countries, their concentrations in filling station dust of Kumasi Metropolis were collated (Table ).
Table 5. Comparison of heavy metals/metalloids concentrations (mg/kg) observed in this study with those found in other heavy metal dust studies
In Table , the mean concentrations of Cr measured agreed with data from Guangzhou and Xiandao, both from China, but lower than those reported in other cities, such as Madrid, Ottawa, Hangzhou, Urumqi. The mean concentrations of Ni measured was comparable with those reported in Oslo, Madrid, Baoji and Urumqi, but was higher in other compared cities except for Shanghai, China. The mean concentration of Cu was lower than cites except for Xiandao. The Zn concentration is similar to Urumqi, while lower than other cities except for Ottawa and Xiandao. The mean concentrations of As and Pb were lower than other compared cites except for Ottawa. However, the mean concentrations of Mn, Fe, Sr, Zr, Ti and Ba were higher in all the compared cities (Table ).
3.3. Multivariate analysis
3.3.1. Source of heavy metals/metalloids pollution
To check the level of heavy metals/metalloids pollution and identify their possible source in the filling station dust, principal component and correlation coefficient analyses were performed (Facchinelli, Sacchi, & Mallen, Citation2001). The relationships among the estimated heavy metals/metalloids were carried out using Pearson’s correlation analysis. Table demonstrates that Mn, Cr, Ti and Zr were significantly correlated (p < 0.01) with each other, signifying similar sources of these metals.
Table 6. Pearson’s correlation matrix for heavy metals/metalloids concentration
Pb was positively correlated with Zn, Cu and Fe; Zn was positively correlated with Mn, Cu, Fe and Cr, but negative with Ni. There existed a significant positive correlation between Cu with Fe and Mn. A similar trend was observed for Fe with Mn and Cr; and for Cr with Ti and Zr. In the case of Ti, there was significant positive correlation with Sr, but negative with Zr. Though the significant positive relationship cannot always be attributed to a similar source, this correlation analysis can still offer interesting information on the pathway and source of heavy metals/ metalloids (Lu et al., Citation2010). For instance, Zn, Cu and Fe, and Pd with significant positive correlation could be originating from anthropogenic sources, such as fossil fuel combustion via atmospheric deposition and leaded fuel. Mo, Ba and As might have originated from different sources with reference to the other heavy metals/metalloids.
In addition to the principal component analysis, Bartlett’s and KMO test were employed to examine the sampling sphericity and adequacy. The PCA results of heavy metals/metalloids are given in Table .
Table 7. Varimax factor loading for heavy metals/metalloids in the filling station dust
Three components with eigenvalues >1 were obtained, which account for 97.80% of the extracted variance. The first component with positive loading for Mo, As, Mn, Ti, Ba, Zr and Sr, whose coefficients were 0.529, 0.945, 0.534, 0.946, 0.524, 0.734 and 0.928, respectively explained 45.25% of the total variance. The Mo, As, Mn, Ti, Ba, Zr and Sr in PC1 were strongly correlated, signifying their similar source. PC1 consisting of As, Mn and Ba were mainly influenced by natural sources (Lu et al., Citation2010). The second component showed strong positive loading (>0.75) for Pb, Zn and Cu, whose coefficients were 0.987, 0.999 and 0.862, respectively explained 33.30% of the total variance. PC2 including Pb, Zn and Cu could be associated with anthropogenic inputs, such as industrial and traffic pollution (Chen, Lu, Li, Gao, & Chang, Citation2014). Moreover, improper disposal of engine oil and a significant amount of emissions influences Pb, Cu and Zn pollution in the filling station dust, as shown in PC2. The third component showed strong loading (>0.75) for Mo (0.830) and Ba (0.851) with 19.25% of the extracted variance. The heavy metals/metalloids in the three PC’s demonstrate their different sources. The correlation between Mo and Ba in PC1 and PC3, suggests their origin from dust as the parent material. The poor loadings of Ni, Fe and Cr can be attributed to differences in sources of materials and geochemical behaviour of parameters (Edet, Merkel, & Offiong, Citation2003), as well as quasi-independent behaviour within the group (Lu et al., Citation2010).
3.4. Heavy metals/metalloids pollution assessment
The estimated Igeo result of heavy metals/metalloids in filling station dust is given in Figure .
Figure 2. Box-plots of the Igeo for heavy metals/metalloids in the filling station dust of Kumasi Metropolis.
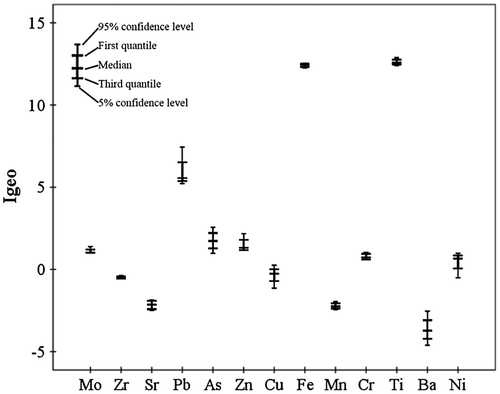
The mean values of Igeo increased following the order: Ba < Mn < Sr < Zr < Cu < Cr < Ni < Mo < As < Zn < Pb < Fe < Ti. The mean Igeo of Mo, Zr, Sr, Cu, Mn, Cr, Ba and Ni showed that dust from filling stations was practically unpolluted. The mean Igeo of As and Zn signify moderately polluted, whereas the mean Igeo for Pb, Fe and Ti designate very highly polluted. In the study area, the Igeo values signify “unpolluted” to “highly polluted” of the investigated heavy metals/metalloids. Among the estimated heavy metals/metalloids, Ti and Fe were significantly accumulated in the filling station dust, as showed by their corresponding mean geochemical accumulation values of 12.46 ± 0.41 and 12.06 ± 0.64. The presence of Zn and As in the filling station dust poses an adverse environmental concern. Similarly, Wei and Yang (Citation2010) deduced that for heavy metals in urban soils in China, Cr and Ni appeared to cause the least contamination in the selected cities, while Cu, Pb, Zn and Cd showed the highest Igeo values. Cr and Ni appear to be the least contaminated elements in all the cities, while Cu, Pb, Zn and Cd show the highest Igeo values for most cities (Wei & Yang, Citation2010). The PIs results for heavy metals/metalloids in the filling station dust varied significantly across the different heavy metals/metalloids (Figure ).
Figure 3. Box-plots of pollution load index and pollution index for heavy metals/metalloids in the filling station dust of Kumasi Metropolis.
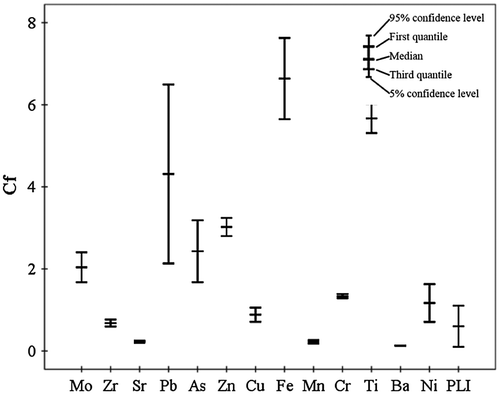
The mean PIs of Fe, Pb and Ti were much higher, varying from 16.51 to 97.02, 0.64 to 9.97 and 29.33 to 88.24, with values of 5.75, 46.67 and 58.45, respectively. This showed that Mo, Zn, As, Cu, Cr and Ni of filling station dust were classified as middle PI. Zr, Sr, Mn and Ba exhibited lower mean values and were classified as low PI. The PI value for the calculated heavy metals/metalloids was in the decreasing order: Ba (0.11) < Mn (0.19) < Sr (0.22) < Zr (2.06) < Cr (1.30) < Cu (1.36) < Ni (1.47) < Mo (2.06) < As (2.82) < Zn (2.85) < Pb (5.75) < Fe (6.54) ≤ Ti (6.54). Therefore, Pb, Fe and Ti were the main contaminants of the study area reaching a very strong contamination level. The high level of Pb in filling station dust samples can be credited to leaded fuels, emissions from tyre wear, bearing wear and lubricating oils (Kamani et al., Citation2015). The PLIs in all filling station dust samples varied between 0.10 and 6.32 with a mean value of 2.20, signifying that the filling station dust was “moderately polluted” in the Kumasi Metropolis.
3.5. Ecological risk index
The PER is a measure of the sensitivity of several biological systems to deadly substances and elucidates the potential risks induced by heavy metals/metalloids (Qing et al., Citation2015; Ying et al., Citation2016). The PER results are presented in Table .
Table 8. Potential ecological risks of heavy metals/metalloids and their ecological index in the filling station dust
The mean value for all the heavy metals/metalloids except arsenic were below 40, thus indicating low PER. This signifies that arsenic has a moderate risk to the environment and human health. The PER index showed the order: As > Pb > Ni > Cu > Cr > Zn > Mn > Ba. To access the overall PER of the observed heavy metals/ metalloids in the filling station dust in the Kumasi metropolis, PER was evaluated as the summation of the risk factors. The contribution to the overall PER displays that arsenic contributed 32% of the total PER. The PER value for all the sampling sites ranged from 1.34 to 784.73 with a mean value of 187.72, indicating low to very high potential ecological risk. Nevertheless, the mean RI shows a considerably high risk (PER > 100) in the Kumasi Metropolis, largely due to arsenic contamination. This should be remediated to prevent the possible ecological adverse effect.
3.6. Human health risk assessment
3.6.1. Exposure assessment
The calculated mean daily intake of heavy metals/metalloids for adult and children in the filling station dust via inhalation, dermal contact and ingestion are presented in Table .
Table 9. Exposure duration (mg/kg-day) for filling station dust in Kumasi Metropolis
The trends of ADDs for heavy metals/metalloids via dermal contact, inhalation and ingestion were in the order: Fe > Ti > Zn > Zr > Mn > Sr > Ba > Cr > Cu > Pb > Ni > As > Mo. The exposure route, which on the average brings about the highest risk for adults and children was ingestion followed by dermal contact with inhalation being the lowest. Qing et al. (Citation2015) observed the same order of daily intake of heavy metals.
The health risks assessment results are given in Table .
Table 10. Non-carcinogenic risk, overall toxic risk and carcinogenic risk (ADDlife × SF)
The HQ results for all the heavy metals/metalloids were < 1, signifying no risk for both adult and children. The mean HQ values for adults and children via ingestion were as follows: Zr > Cr > As > Pb > Ni > Cu > Mn > Mo ≥ Zn > Fe > Ba > Sr > Ti, while that via inhalation were Cr > As > Pb > Zn > Ni > Cu > Mn > Mo > Fe > Ba > Sr > Ti > Zr and via dermal contact were Ti > Fe > Zn > Sr > Mn > Ba > Cu > Ni > Cr > Pb > Zr > Mo > As. In summary, the mean exposure route of heavy metals/metalloids for adults and children decreased according to the following order: inhalation < dermal contact < ingestion. In the present study, cutaneous and oral exposure are more important than inhalation. The findings in this study agree well with other studies (Benhaddya, Boukhelkhal, Halis, & Hadjel, Citation2016), which also observe ingestion to be the highest exposure pathway to health risk. The contribution of HQing for most of the heavy metals/metalloids were the highest with 11.83% for adults and 88.17% for children. This confirms ingestion as the major exposure route that can adversely affect human health. The HI results via inhalation and dermal contact with all the heavy metals/metalloids were < 1, except for ingestion in adult, signifying no non-carcinogenic risk. The HI values in children were observed to develop more non-carcinogenic risk in the filling station dust compared to adults in the Kumasi Metropolis. This can be attributed to the fact that children tend to have significant contact with soil during their outdoor play activities and are more likely to have a direct hand-to-mouth exposure of dust (Luo et al., Citation2012). Similar findings can be seen from other studies including Qu, Sun, Wang, Huang, and Bi (Citation2012), Zota et al. (Citation2011), and Man et al. (Citation2010). Owing to lack of carcinogenic slope factor for Cu, Ba, Mn, Fe, Ni, Ti, Mo, Zn, Sr and Zr, only the slope factors for As, Cr and Pb were given in the literature. The RI values in the filling station dusts of Kumasi Metropolis were 8.74 x 10−8 (As), 3.31 x 10−7 (Cr), and 3.75 x 10−9 (Pb) for children and 1.17 x 10−7 (As), 4.44 x 10−10(Cr) and 5.03 x 10−9 (Pb) for adults (Table ). The risk index of Cr, As and Pb for both adults and children were < 10−6, showing no plausible carcinogenic risk. However, the HI level via ingestion indicates that the carcinogenic risks of the observed heavy metals/metalloids cannot be overlooked.
4. Conclusion
The concentration, pollution level and health risks assessment of heavy metals/metalloids in dust from filling stations within the Kumasi Metropolis, Ghana were evaluated in this study. This study offers significant information on the concentrations of Fe, Ti, Zr, Mn, Sr, Ba, Zn, Cr, Cu, Pd, As, Ni and Mo in dust impacted by fuel and other related emissions. The PCA analysis identified anthropogenic inputs and natural origin as the main sources of heavy metals/metalloids in the filling station dust of Kumasi Metropolis. The PI and Igeo revealed the order of Ba < Mn < Sr < Zr < Cu < Cr < Ni < Mo < As < Zn < Pb < Fe < Ti for both Igeo and PI. The higher PI values for Pb, Fe and Ti in the filling station dust indicated a very strong pollution level, which could be attributed to leaded fuels and emissions from bearing wear, lubricating oils and tyre wear. The PLIs in all filling station dust samples varied between 0.10 and 6.32 with an average value of 2.20, signifying moderately pollution in the Kumasi Metropolis. The mean value of r all the heavy metals/metalloids except As were below 40, indicating low risk. The PER value for all the sampling sites ranged from 1.34 to 784.73 with a mean value of 187.72, The HQ results indicate no risk for both adult and children, as the HQ values were lower than safe limit. The contributions of HQing for most of the heavy metals/metalloids were the highest with 11.83% for adults and 88.17% for children. This signifies ingestion as the major exposure route that can adversely affect human health. The HI values were <1, except for HI value for ingestion in adult, signifying no non-carcinogenic risk. Nonetheless, the carcinogenic risks values for adults were lower than the threshold value of 1 × 10−6, showing no carcinogenic risk. The main exposure pathway for both children and adults is ingestion, followed by dermal contact and inhalation. The health risk assessment revealed ingestion as the major exposure route with children being the most exposed to heavy metals/metalloids pollution compared to adults in the Kumasi Metropolis, Ghana. Therefore, the results in this study will be beneficial for environmental planning authorities in the Kumasi Metropolis to manage and control further heavy metals/metalloids pollution at the various filling station sites in the Metropolis.
Compliance with ethical standards
Yes
Authors’ contributions
All the authors contributed equally to the preparation of this manuscript. All authors read and approved the final manuscript.
Funding
The authors received no direct funding for this research.
Acknowledgement
The authors are grateful to Prof Matt Dodd of the School of Environment and Sustainability of Royal Roads University, Victoria BC, Canada for the provision of the equipment for the analysis of samples in this study.
Additional information
Notes on contributors
Marian Asantewah Nkansah
The group is involved on the determination of levels and distribution of heavy metals in the environment. The current study on fuel stations forms part of the country-wide study to determine the effects of human activities on heavy metals and the potential health associated with their exposure.
References
- Afrifa, C. G., Ofosu, F. G., Bamford, S. A., Wordson, D. A., Atiemo, S. M., Aboh, I. J., & Adeti, J. P. (2013). Heavy metal contamination in surface soil dust at selected fuel filling stations in Accra, Ghana. American Journal of Scientific and Industrial Research, 4, 404–413.
- Agorku, E. S., Kwaansa-Ansah, E. E., Voegborlo, R. B., Amegbletor, P., & Opoku, F. (2016). Mercury and hydroquinone content of skin toning creams and cosmetic soaps, and the potential risks to the health of Ghanaian women. SpringerPlus, 5, 1–5. doi:10.1186/s40064-016-1967-1
- Ahmed, F., & Ishiga, H. (2006). Trace metal concentrations in street dusts of Dhaka city, Bangladesh. Atmospheric Environment, 40, 3835–3844.10.1016/j.atmosenv.2006.03.004
- Akoto, O., Ephraim, J., & Darko, G. (2008). Heavy metals pollution in surface soils in the vicinity of abundant railway servicing workshop in Kumasi, Ghana. International Journal of Environmental Research, 2, 359–364.
- Akoto, O., Ephraim, J., & Darko, G. (2009). Heavy metals pollution in surface soils in the vicinity of abundant railway servicing workshop in Kumasi, Ghana. International Journal of Environmental Research, 3, 359–364.
- Allen, A., Nemitz, E., Shi, J., Harrison, R., & Greenwood, J. (2001). Size distributions of trace metals in atmospheric aerosols in the United Kingdom. Atmospheric Environment, 35, 4581–4591.10.1016/S1352-2310(01)00190-X
- Asare-Donkor, N. K., Kwaansa-Ansah, E. E., Opoku, F., & Adimado, A. A. (2015). Concentrations, hydrochemistry and risk evaluation of selected heavy metals along the Jimi River and its tributaries at Obuasi a mining enclave in Ghana. Environmental Systems Research, 4, 1–14.
- Atiemo, M. S., Ofosu, G. F., Kuranchie-Mensah, H., Tutu, A. O., Palm, N., & Blankson, S. A. (2011). Contamination assessment of heavy metals in road dust from selected roads in Accra, Ghana. Research Journal of Environmental and Earth Sciences, 3, 473–480.
- Banerjee, A. D. (2003). Heavy metal levels and solid phase speciation in street dusts of Delhi, India. Environmental Pollution, 123, 95–105.10.1016/S0269-7491(02)00337-8
- Benhaddya, M. L., Boukhelkhal, A., Halis, Y., & Hadjel, M. (2016). Human health risks associated with metals from Urban Soil and road dust in an oilfield area of Southeastern Algeria. Archives of Environmental Contamination and Toxicology, 70, 556.10.1007/s00244-015-0244-6
- Boateng, T. K., Opoku, F., Acquaah, S. O., & Akoto, O. (2015). Pollution evaluation, sources and risk assessment of heavy metals in hand-dug wells from Ejisu-Juaben Municipality, Ghana. Environmental Systems Research, 4, 1–18. doi:10.1186/s40068-015-0045-y
- Chabukdhara, M., & Nema, A. K. (2013). Heavy metals assessment in urban soil around industrial clusters in Ghaziabad, India: Probabilistic health risk approach. Ecotoxicology and Environmental Safety, 87, 57–64. doi:10.1016/j.ecoenv.2012.08.032.
- Chen, H., Lu, X., Li, L. Y., Gao, T., & Chang, Y. (2014). Metal contamination in campus dust of Xi’an, China: A study based on multivariate statistics and spatial distribution. Science of the Total Environment, 484, 27–35.10.1016/j.scitotenv.2014.03.026
- Chen, J., Wei, F., Zheng, C., Wu, Y., & Adriano, D. C. (1991). Background concentrations of elements in soils of China. Water, Air, and Soil Pollution, 57–58(1), 699–712. doi:10.1007/BF00282934.
- Chen, X., Lu, X., & Yang, G. (2012). Sources identification of heavy metals in urban topsoil from inside the Xi’an Second Ringroad, NW China using multivariate statistical methods. Catena, 98, 73–78.10.1016/j.catena.2012.06.007
- Chen, H., Teng, Y., Lu, S., Wang, Y., & Wang, J. (2015). Contamination features and health risk of soil heavy metals in China. Science of the Total Environment, 512, 143–153.10.1016/j.scitotenv.2015.01.025
- Chen, T.-B., Zheng, Y.-M., Lei, M., Huang, Z.-C., Wu, H.-T., Chen, H., … Tian, Q.-Z. (2005). Assessment of heavy metal pollution in surface soils of urban parks in Beijing, China. Chemosphere, 60, 542–551.10.1016/j.chemosphere.2004.12.072
- Christoforidis, A., & Stamatis, N. (2009). Heavy metal contamination in street dust and roadside soil along the major national road in Kavala’s region, Greece. Geoderma, 151, 257–263.10.1016/j.geoderma.2009.04.016
- Dauda, M., & Odoh, R. (2012). Heavy metals assessment of soil in the vicinity of fuel filling station in some selected local government areas of Benue State, Nigeria. Pelagia Research Library, Der Chemica Sinica, 3, 1329–1336.
- de Miguel, E., Llamas, J. F., Chacón, E., Berg, T., Larssen, S., Røyset, O., & Vadset, M. (1997). Origin and patterns of distribution of trace elements in street dust: Unleaded petrol and urban lead. Atmospheric Environment, 31(17), 2733–2740. doi:10.1016/S1352-2310(97)00101-5.
- Dong, J., Yu, M., Bian, Z., Wang, Y., & Di, C. (2011). Geostatistical analyses of heavy metal distribution in reclaimed mine land in Xuzhou, China. Environmental Earth Sciences, 62, 127–137.10.1007/s12665-010-0507-5
- Duong, T. T., & Lee, B.-K. (2011). Determining contamination level of heavy metals in road dust from busy traffic areas with different characteristics. Journal of Environmental Management, 92, 554–562.10.1016/j.jenvman.2010.09.010
- Duzgoren-Aydin, N. S., Wong, C. S. C., Song, Z. G., Aydin, A., Li, X. D., & You, M. (2006). Fate of heavy metal contaminants in road dusts and gully sediments in Guangzhou, SE China: A chemical and mineralogical assessment. Human and Ecological Risk Assessment: An International Journal, 12(2), 374–389. doi:10.1080/10807030500538005.
- Edet, A., Merkel, B., & Offiong, O. (2003). Trace element hydrochemical assessment of the Calabar Coastal Plain Aquifer, southeastern Nigeria using statistical methods. Environmental Geology, 44, 137–149.
- Ekperusi, O. A., & Aigbodion, I. F. (2015). Bioremediation of heavy metals and petroleum hydrocarbons in diesel contaminated soil with the earthworm: Eudrilus eugeniae. SpringerPlus, 4, 493.10.1186/s40064-015-1328-5
- Emmanuel, A., Cobbina, S., Adomako, D., Duwiejuah, A., & Asare, W. (2014). Assessment of heavy metals concentration in soils around oil filling and service stations in Tamale Metropolis, Ghana. African Journal of Environmental Science and Technology, 8, 256–266.10.5897/AJEST
- Eze, P. N., Udeigwe, T. K., & Stietiya, M. H. (2010). Distribution and potential source evaluation of heavy metals in prominent soils of Accra Plains, Ghana. Geoderma, 156, 357–362.10.1016/j.geoderma.2010.02.032
- Facchinelli, A., Sacchi, E., & Mallen, L. (2001). Multivariate statistical and GIS-based approach to identify heavy metal sources in soils. Environmental Pollution, 114, 313–324.10.1016/S0269-7491(00)00243-8
- Ghana Statistical Services. (2010). 2010 population and housing census: Summary report of final results. Ghana: Accra.
- Fergusson, J. E., Forbes, E. A., Schroeder, R. J., & Ryan, D. E. (1986). The elemental composition and sources of house dust and street dust. Science of The Total Environment, 50, 217–221. doi:10.1016/0048-9697(86)90363-3.
- Glorennec, P., Lucas, J.-P., Mandin, C., & Le Bot, B. (2012). French children's exposure to metals via ingestion of indoor dust, outdoor playground dust and soil: Contamination data. Environment International, 45, 129–134.10.1016/j.envint.2012.04.010
- Guo, W., Huo, S., Xi, B., Zhang, J., & Wu, F. (2015). Heavy metal contamination in sediments from typical lakes in the five geographic regions of China: Distribution, bioavailability, and risk. Ecological Engineering, 81, 243–255.10.1016/j.ecoleng.2015.04.047
- Hakanson, L. (1980). An ecological risk index for aquatic pollution control. A sedimentological approach. Water Research, 14, 975–1001.
- Han, L., Gao, B., Wei, X., Xu, D., & Gao, L. (2016). Spatial distribution, health risk assessment, and isotopic composition of lead contamination of street dusts in different functional areas of Beijing, China. Environmental Science and Pollution Research, 23, 3247.10.1007/s11356-015-5535-y
- He, B., Yun, Z., Shi, J., & Jiang, G. (2013) Research progress of heavy metal pollution in China: Sources, analytical methods, status, and toxicity. Chinese Science Bulletin, 1-7
- Hu, X., Zhang, Y., Ding, Z., Wang, T., Lian, H., Sun, Y., & Wu, J. (2012). Bioaccessibility and health risk of arsenic and heavy metals (Cd Co, Cr, Cu, Ni, Pb, Zn and Mn) in TSP and PM2.5 in Nanjing, China. Atmospheric Environment, 57, 146–152.10.1016/j.atmosenv.2012.04.056
- Huamain, C., Chunrong, Z., Cong, T., & Yongguan, Z. (1999) Heavy metal pollution in soils in China: Status and countermeasures. Ambio, 130–134
- Hussain, K., Rahman, M., Prakash, A., & Hoque, R. R. (2015). Street dust bound PAHs, carbon and heavy metals in Guwahati city–Seasonality, toxicity and sources. Sustainable Cities and Society, 19, 17–25.10.1016/j.scs.2015.07.010
- Islam, S., Ahmed, K., & Habibullah-Al-Mamun, S. (2015). Potential ecological risk of hazardous elements in different land-use urban soils of Bangladesh. Science of the Total Environment, 512, 94–102.10.1016/j.scitotenv.2014.12.100
- Kamani, H., Ashrafi, S. D., Isazadeh, S., Jaafari, J., Hoseini, M., Mostafapour, F. K., … Mahvi, A. H. (2015). Heavy metal contamination in street dusts with various land uses in Zahedan, Iran. Bulletin of Environmental Contamination and Toxicology, 94, 382.10.1007/s00128-014-1453-9
- Karimi Nezhad, M. T., Tabatabaii, S. M., & Gholami, A. (2015). Geochemical assessment of steel smelter-impacted urban soils, Ahvaz. Iran. Journal of Geochemical Exploration, 152, 91–109. doi:10.1016/j.gexplo.2015.02.005.
- Khairy, M. A., Barakat, A. O., Mostafa, A. R., & Wade, T. L. (2011). Multielement determination by flame atomic absorption of road dust samples in Delta Region, Egypt. Microchemical Journal, 97, 234–242.10.1016/j.microc.2010.09.012
- Khorshid, M. S. H., & Thiele-Bruhn, S. (2016). Contamination status and assessment of urban and non-urban soils in the region of Sulaimani City, Kurdistan, Iraq. Environmental Earth Sciences, 16, 1–15.
- Kwaansa-Ansah, E. E., Agorku, S. E., & Nriagu, J. O. (2011). Levels of total mercury in different fish species and sediments from the Upper Volta Basin at Yeji in Ghana. Bulletin of Environmental Contamination and Toxicology, 86, 406–409. doi:10.1007/s00128-011-0214-2
- Laidlaw, M. A., & Taylor, M. P. (2011). Potential for childhood lead poisoning in the inner cities of Australia due to exposure to lead in soil dust. Environmental Pollution, 159, 1–9.10.1016/j.envpol.2010.08.020
- Lee, J.-M., Qin, S. J., & Lee, I.-B. (2006). Fault detection and diagnosis based on modified independent component analysis. Process Systems Engineering, 52, 3501–3514. doi:10.1002/aic.10978.
- Lee-Steere, C. (2009). Environmental Risk Assessment Guidance Manual. Environment Protection and Heritage Council. Australian Environment Agency. Commonwealth of Australia.
- Li, F., Zhang, J., Huang, J., Huang, D., Yang, J., Song, Y., & Zeng, G. (2016). Heavy metals in road dust from Xiandao District, Changsha City, China: characteristics, health risk assessment, and integrated source identification. Environmental Science and Pollution Research, 23(13), 13100–13113. doi:10.1007/s11356-016-6458-y.
- Li, Z., Ma, Z., van der Kuijp, T. J., Yuan, Z., & Huang, L. (2014). A review of soil heavy metal pollution from mines in China: Pollution and health risk assessment. Science of the Total Environment, 468, 843–853.10.1016/j.scitotenv.2013.08.090
- Li, X., Poon, C.-S., & Liu, P. S. (2001). Heavy metal contamination of urban soils and street dusts in Hong Kong. Applied Geochemistry, 16, 1361–1368.10.1016/S0883-2927(01)00045-2
- Lin, Y., Fang, F., Wang, F., & Xu, M. (2015). Pollution distribution and health risk assessment of heavy metals in indoor dust in Anhui rural, China. Environmental Monitoring and Assessment, 187, 1.
- Ling, W., Shen, Q., Gao, Y., Gu, X., & Yang, Z. (2008). Use of bentonite to control the release of copper from contaminated soils. Soil Research, 45, 618–623.
- Lu, X., Wang, L., Li, L. Y., Lei, K., Huang, L., & Kang, D. (2010). Multivariate statistical analysis of heavy metals in street dust of Baoji, NW China. Journal of Hazardous Materials, 173, 744–749.10.1016/j.jhazmat.2009.09.001
- Lu, X., Zhang, X., Li, L. Y., & Chen, H. (2014). Assessment of metals pollution and health risk in dust from nursery schools in Xi’an, China. Environmental Research, 128, 27–34.10.1016/j.envres.2013.11.007
- Luo, X.-S., Yu, S., & Zhu, Y.-G. (2012). Trace metal contamination in urban soils of China. Science of the Total Environment, 421–422, 17–30.10.1016/j.scitotenv.2011.04.020
- Ma, L., Yang, Z., Li, L., & Wang, L. (2016). Source identification and risk assessment of heavy metal contaminations in urban soils of Changsha, a mine-impacted city in Southern China. Environmental Science and Pollution Research, 23, 17058–17066.10.1007/s11356-016-6890-z
- Man, Y. B., Sun, X. L., Zhao, Y. G., Lopez, B. N., Chung, S. S., Wu, S. C., … Wong, M. H. (2010). Health risk assessment of abandoned agricultural soils based on heavy metal contents in Hong Kong, the world’s most populated city. Environment International, 36, 570–576.10.1016/j.envint.2010.04.014
- Manahan, S. (2000). Environmenal chemistry (p. 898). Boca Raton, FL: Lewis.
- McLaughlin, M. J., Hamon, R., McLaren, R., Speir, T., & Rogers, S. (2000). Review: A bioavailability-based rationale for controlling metal and metalloid contamination of agricultural land in Australia and New Zealand. Australian Journal of Soil Research, 38, 1037–1086.10.1071/SR99128
- Muller, G. (1969) Index of Geoaccumulation in Sediments of the Rhine River. GeoJournal, 2, 108–118.
- Nkansah, M. A., Opoku, F., & Ackumey, A. A. (2016). Risk assessment of mineral and heavy metal content of selected tea products from the Ghanaian market. Environmental Monitoring and Assessment, 188, 1–11. doi:10.1007/s10661-016-5343-y
- Obiri, S. (2007). Determination of heavy metals in water from boreholes in Dumasi in the Wassa West District of Western Region of Republic of Ghana. Environmental Monitoring and Assessment, 130, 455.10.1007/s10661-006-9435-y
- Pan, L., Ma, J., Hu, Y., Su, B., Fang, G., Wang, Y., … Xiang, B. (2016). Assessments of levels, potential ecological risk, and human health risk of heavy metals in the soils from a typical county in Shanxi Province, China. Environmental Science and Pollution Research, 23, 19330–19340.10.1007/s11356-016-7044-z
- Pant, P., & Harrison, R. M. (2013). Estimation of the contribution of road traffic emissions to particulate matter concentrations from field measurements: A review. Atmospheric Environment, 77, 78–97.10.1016/j.atmosenv.2013.04.028
- Peat, J., & Barton, B. (2008) Medical statistics: A guide to data analysis and critical appraisal. Hoboken, NJ: John Wiley & Sons.
- Qing, X., Yutong, Z., & Shenggao, L. (2015). Assessment of heavy metal pollution and human health risk in urban soils of steel industrial city (Anshan), Liaoning, Northeast China. Ecotoxicology and Environmental Safety, 120, 377–385.10.1016/j.ecoenv.2015.06.019
- Qu, C., Sun, K., Wang, S., Huang, L., & Bi, J. (2012). Monte Carlo simulation-based health risk assessment of heavy metal soil pollution: A case study in the Qixia Mining Area, China. Human and Ecological Risk Assessment: An International Journal, 18, 733–750.10.1080/10807039.2012.688697
- Rasmussen, P. E., Subramanian, K. S., & Jessiman, B. J. (2001). A multi-element profile of house dust in relation to exterior dust and soils in the city of Ottawa. Canada. Science of the Total Environment, 267(1–3), 125–140. doi:10.1016/S0048-9697(00)00775-0.
- Ruiz-Jimenez, J., Parshintsev, J., Laitinen, T., Hartonen, K., Petäjä, T., Kulmala, M., & Riekkola, M.-L. (2012). Influence of the sampling site, the season of the year, the particle size and the number of nucleation events on the chemical composition of atmospheric ultrafine and total suspended particles. Atmospheric Environment, 49, 60–68.10.1016/j.atmosenv.2011.12.032
- Salati, S., & Moore, F. (2010). Assessment of heavy metal concentration in the Khoshk River water and sediment, Shiraz, Southwest Iran. Environmental Monitoring and Assessment, 164, 677–689.10.1007/s10661-009-0920-y
- Schleicher, N. J., Norra, S., Chai, F., Chen, Y., Wang, S., Cen, K., … Stüben, D. (2011). Temporal variability of trace metal mobility of urban particulate matter from Beijing–A contribution to health impact assessments of aerosols. Atmospheric Environment, 45, 7248–7265.10.1016/j.atmosenv.2011.08.067
- Sezgin, N., Ozcan, H. K., Demir, G., Nemlioglu, S., & Bayat, C. (2004). Determination of heavy metal concentrations in street dusts in Istanbul E-5 highway. Environment International, 29, 979–985.10.1016/S0160-4120(03)00075-8
- Shen, F., Liao, R., Ali, A., Mahar, A., Guo, D., Li, R., … Zhang, Z. (2017). Spatial distribution and risk assessment of heavy metals in soil near a Pb/Zn smelter in Feng County, China. Ecotoxicology and Environmental Safety, 139, 254–262.10.1016/j.ecoenv.2017.01.044
- Shi, G., Chen, Z., Xu, S., Zhang, J., Wang, L., Bi, C., & Teng, J. (2008). Potentially toxic metal contamination of urban soils and roadside dust in Shanghai. China. Environmental Pollution, 156(2), 251–260. doi:10.1016/j.envpol.2008.02.027.
- Soltani, B., Keshavarzi, F., Moore, T., Tavakol, A. R., Lahijanzadeh, N., & Jaafarzadeh, M. K. (2015). Ecological and human health hazards of heavy metals and polycyclic aromatic hydrocarbons (PAHs) in road dust of Isfahan metropolis, Iran. Science of the Total Environment, 505, 712–723.10.1016/j.scitotenv.2014.09.097
- Steinskog, D. J., Tjøstheim, D. B., & Kvamstø, N. G. (2007). A cautionary note on the use of the Kolmogorov–Smirnov test for normality. Monthly Weather Review, 135, 1151–1157.10.1175/MWR3326.1
- Taylor, S. R., & McLennan, S. M. (1995). The geochemical evolution of the continental crust. Reviews of Geophysics, 33, 241–265.10.1029/95RG00262
- Teng, Y., Wu, J., Lu, S., Wang, Y., Jiao, X., & Song, L. (2014). Soil and soil environmental quality monitoring in China: A review. Environment International, 69, 177–199.10.1016/j.envint.2014.04.014
- USEPA. (1986). Guidelines for the health risk assessment of chemical mixtures. Washington, DC: US Environmental Protection Agency.
- USEPA. (1989) Risk assessment guidance for Superfund. Human health evaluation manual, (part A) (Vol. 1). Washington, DC: Office of emergency and remedial response.
- USEPA. (1993). Assessing dermal exposure from soil: Region 3 technical guidance manual, risk assessment. United States Environmental PROTECTION agency. Hazardous Waste Management Division Office of Superfund Programs. Philadelphia. Retrieved from https://www.epa.gov/risk/assessing-dermal-exposure-soil
- USEPA. (2002). Supplemental guidance for developing soil screening levels for Superfund sites. Washington, DC: Soild Waste and Emergency Response.
- USEPA. (2003). Example exposure scenarios National Center for Environmental Assessment. Washington, DC. EPA/600/R-03/036. National Information Service, Springfield, VA; PB2003-103280. Retrieved from http://www.epa.gov/ncea
- USEPA. (2011). Exposure factors handbook. National Center for Environmental Assessment. Washington, DC: United State Environmental Protection Agency.
- Wang, M., Markert, B., Chen, W., Peng, C., & Ouyang, Z. (2012). Identification of heavy metal pollutants using multivariate analysis and effects of land uses on their accumulation in urban soils in Beijing, China. Environmental Monitoring and Assessment, 184, 5889–5897.10.1007/s10661-011-2388-9
- Wei, B., Jiang, F., Li, X., & Mu, S. (2009). Spatial distribution and contamination assessment of heavy metals in urban road dusts from Urumqi. NW China. Microchemical Journal, 93(2), 147–152. doi:10.1016/j.microc.2009.06.001.
- Wei, B., & Yang, L. (2010). A review of heavy metal contaminations in urban soils, urban road dusts and agricultural soils from China. Microchemical Journal, 94, 99–107.10.1016/j.microc.2009.09.014
- Ying, L., Shaogang, L., & Xiaoyang, C. (2016). Assessment of heavy metal pollution and human health risk in urban soils of a coal mining city in East China. Human and Ecological Risk Assessment: An International Journal, 22, 1359–1374.10.1080/10807039.2016.1174924
- Zhang, M. & Wang, H. (2009). Concentrations and chemical forms of potentially toxic metals in road-deposited sediments from different zones of Hangzhou. China. Journal of Environmental Sciences, 21(5), 625–631. doi:10.1016/S1001-0742(08)62317-7.
- Zhang, X., Yang, L., Li, Y., Li, H., Wang, W., & Ye, B. (2012). Impacts of lead/zinc mining and smelting on the environment and human health in China. Environmental Monitoring and Assessment, 184, 2261–2273. doi:10.1007/s10661-011-2115-6
- Zhou, Q., & Song, Y. (2004) Remediation of contaminated soils: Principles and methods (pp. 1–489). Beijing: Science.
- Zhou, L., Yang, B., Xue, N., Li, F., Seip, H. M., Cong, X., … Li, H. (2014). Ecological risks and potential sources of heavy metals in agricultural soils from Huanghuai Plain, China. Environmental Science and Pollution Research, 21, 1360–1369.10.1007/s11356-013-2023-0
- Zota, A. R., Schaider, L. A., Ettinger, A. S., Wright, R. O., Shine, J. P., & Spengler, J. D. (2011). Metal sources and exposures in the homes of young children living near a mining-impacted Superfund site. Journal of Exposure Science and Environmental Epidemiology, 21, 495.10.1038/jes.2011.21