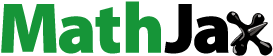
Abstract
This study sought to examine the impact of dynamic mathematics software, GeoGebra, on university mathematics students’ understanding of polar coordinates. A quasi-experimental design with an equivalent group of 42 participants in each group was used. A simple random sampling approach was used to select the 84 participants from the population and a purposive sampling technique was used to assign the participants to the various groups. A t-test analysis was conducted on the pre-test and post-test activities. It was revealed that students who were taught polar coordinates with the aid of GeoGebra performed better than those who were taught with the conventional approach. Analysis of students in the experimental group’s responses to the questionnaire showed that they demonstrated positive attitudes and perceptions concerning the use of GeoGebra software in the learning of polar coordinates. It was concluded that GeoGebra is more effective in improving university mathematics students’ understanding of polar coordinates.
1. Introduction
The utilization of computer-assisted instruction and innovative devices in instruction are picking up much acknowledgment in this 21st century (Oral et al., Citation2004). Current technology such as educational technological applications has proven to aid in the creation of interactive classroom learning, which incorporates a great impact on students’ knowledge of scientific concepts and achievement levels in mathematics. (Alabdulaziz et al., Citation2020).
According to the National Council of Teachers of Mathematics (NCTM, Citation2003), accessibility of educational technology has improved the teaching and learning of mathematics. In mathematics instruction, computers have expanded the significance of some mathematical thoughts and ideas. Also, they have brought new techniques to represent and process mathematical ideas, as well as content and pedagogical options (Masri et al., Citation2016).
A lot of mathematical software have been widely practised around the globe. Some of this mathematical software are Geometer’s Sketchpads (GSP), Autograph, Maple, Matlab Mathematics, and others (Kamariah et al., Citation2010). The evolution of mathematical software programs occurred during the last few decades (Y. F. Zengin & Kutluca, Citation2012). Computer Algebra Systems (CAS) and Dynamic Geometry Software (DGS) are two prominent types of software packages that have contributed to the teaching and learning of mathematics. CAS and DGS have been combined into one software package called GeoGebra, which combines their capabilities for mathematics education (Antohe, Citation2010; Dikovic, Citation2009; Hohenwarter, et al., , Citation2007). According to Masri et al. (Citation2016), such tools have justified their importance in teaching and learning mathematics. With regards to autographs, Kamariah et al. (Citation2010) asserted that they are important educational software that students can use to animate and change graphs, shapes or vectors already sketched. Computer-assisted instruction activities stimulate students’ interest and encourage their understanding of mathematical concepts or phenomenon (Masri et al., Citation2016).
Studies on the usage of GeoGebra in teaching some concepts in mathematics contents like calculus, trigonometry, statistics, algebra, and geometry have been done over the years. In the area of geometry, researchers have investigated concepts like coordinate geometry, geometric transformation, analytic geometry, triangles, circle, circle theorem, and polar coordinates. Alabdulaziz et al. (Citation2020) examined the effectiveness of GeoGebra on selected high school students in Saudi Arabia in their understanding of polar coordinates and complex numbers. They found that students who were taught with GeoGebra had higher achievement scores and higher learning outcomes than those who were not taught with GeoGebra. Y. Zengin and Tatar (Citation2015) conducted a similar study on the effectiveness of GeoGebra on their polar coordinate performance in student teachers in Turkey, using qualitative and quantitative designs in a single group in the study. They also found that computer-assisted instruction with GeoGebra positively contributed to future teachers’ success in understanding polar coordinates.
Polar coordinates were used for the specific purpose of studying particular curves long before it was revealed to be a general geometrical instrument. Bonaventura Cavalieri, according to Alabdulaziz et al. (Citation2020), was the first to use polar coordinates to find the area inside an Archimedean Spiral by correlating it with the parabola. Newton thought about polar coordinates as a technique to fix any point in space (Coolidge, Citation1952). Newton just looked at polar coordinates in relation to Cartesian, bipolar, and other systems, with the goal of demonstrating how they can be used to compute the tangent when the curve’s equation is known. Since that era, polar coordinates have been found to be such an important concept in mathematics, which has been incorporated in most mathematics curricula worldwide. Most universities in Ghana have polar coordinates as part of the contents to be taught to students offering varied mathematics programmes due to its numerous applications in all spheres of human life.
A meaningful understanding of polar coordinates is as important as many mathematical concepts due to its applications. It is important in many fields like mathematics, physics, and engineering (Y. Zengin & Tatar, Citation2015). According to Alabdulaziz et al. (Citation2020), polar coordinates are very important for the study of concepts like calculus, complex numbers, and solving problems that make use of correspondence or motion around a central purpose of victimization modeling. However, several studies have shown that students regularly have difficulty with understanding the concept of polar coordinates (Montiel et al., Citation2009; Paoletti et al., Citation2013; Sayre & Wittman, Citation2007). The use of GeoGebra in the instruction of mathematical concepts that are most difficult to students such as the Circle theorem has shown to be effective in improving their understanding of students’ learning in Ghana (Tay & Wonkyi, Citation2018).
The advocacy for using ICT and its tools in the teaching and learning of mathematics in the classroom started long time ago. The Mathematical Association of Ghana (MAG) took a significant step toward the use of ICT in the teaching and learning of Mathematics, which to them was going to provide an effective tool for social transformation (Suleman, Citation2012). The Association believes that using technology to teach and learn Mathematics will help students visualize, think, reason, analyze, and articulate logically. The use of computers allows teachers to design lessons that increase learners’ interest and eagerness to study and enjoy Mathematics (Suleman, Citation2012). Despite the importance of educational technology and strong advocacy for its use, Classrooms in Ghana are still characterized by traditional methods of teaching and learning mathematics. This teaching method is more teacher-centered than learner-centered. This situation results in students who can calculate but do not know how to solve real world problems that require concepts and mathematical skills. This phenomenon still persists because enough studies on the effectiveness of using ICT tools in teaching and learning mathematics have not been done in Ghana.
The few studies that have been conducted on the effect of ICT tools (GeoGebra) in the teaching and learning of mathematical concepts in Ghana (Tay & Wonkyi, Citation2018) focused on the teaching and learning of mathematics at the pre-tertiary education level. This is the phenomenon that is observed from literature on the world stage as well. The characterization of the Ghanaian mathematics classroom is caused by this, since the tertiary level is where teachers for all educational levels are trained. This demands that the use of ICT tools in learning mathematics be studied at that level to contribute to the advocacy of using ICT tools in teaching mathematical concepts. A study at the tertiary level on the effectiveness of polar coordinates however is one of the concepts in mathematics that the effect of GeoGebra has not been felt. This study sought to determine the impact of using GeoGebra on the understanding of polar coordinate system by mathematics students at the university.
2. Theoretical framework
This study uses the social constructivist theory and the zone of proximal development to teach university students the concept of polar coordinates in an interactive classroom using GeoGebra software. Constructivists believe that when learners reflect on their experiences, they construct their own understanding of the world around them. Social constructivism did not start today. According to Sivan (Citation1986), based on the evolving research in educational tradition, sociology, and cognitive psychology, social constructivism did not start recently. He reported that Cloe and others who engaged in comparative cognitive research reintroduced the writings of the Soviet Social Constructivism. Considering the increasing importance of social constructivism in the thought of school teaching, and learning, educational psychologists such as Shulman and Carey (Citation1984) have described social constructivism as a representation of a change of focus toward the consideration of meaning-making as a psychological event via mutual interaction with the influence of the individual and the context. Lev Vygotsky was convinced that social interaction fundamentally contributes to the development of cognition (Wood, Citation1999). In his view, culture was a determining factor in individual development. He then proposed that culture makes two types of contributions to learners’ intellectual development. The first is that the learner acquires most of the content of their cognition from it and the second is that they get the processes or means of their cognition from the culture. Culture simply teaches learners what to do and how to think. To him, learning is based on social interaction. Vygotsky highlighted that a child (learner) learns best with the help of someone (adult) who has much knowledge of the concepts. The interest of this is on the learner who is limited to a time frame that he called Zone of Proximal Development (ZPD).
In this study, the zone of proximal development (ZPD) was applied in a way that when learning polar coordinates, the instructor helped the students to understand concepts. This helped their peers to gain a clear view and develop a different way of understanding the polar coordinates system. Whilst they are working in groups as students, they gain different insight and understanding of the polar coordinates since they have different ZPD. This happens because individuals’ views may differ, which means that the interaction with their colleagues could lead to shared understanding in situations like this and there must be an agreement of the insights and ideas by the individual student. The students reach this understanding after the sharing of views and justification of same. A process of such nature helps students participate in critical thinking skills. ZPD can be explained as the difference between a person’s mental age and the level the person can achieve in solving problems with guidance. GeoGebra was used as a scaffold to mitigate students challenge in understanding polar coordinates as an educational activity and as a means for individual students to achieve their ZPD. The intervention was introduced at the beginning of the lesson on the first day. Students were allowed to work in pairs whilst following the steps provided as a guide for their learning. The students were encouraged to actively participate in the activities. Whilst interacting with each other, they got the opportunity to guide them and reach an understanding. This learning environment is the kind Vygotsky advocates for, where he placed emphases on social interaction and cultural environment in order to make a contribution to the cognitive development of learners. During the intervention stage in this study, students were allowed to provide assistance to each other and in some cases, they were put into groups which allowed the scaffolding process to take place to learn the concepts. Such arrangement put the instructor in the role of a facilitator.
3. GeoGebra in mathematics instructions
GeoGebra allows both students and teachers to generate mathematical objects and relate with them. They use an interactive environment to explain, search and handle concepts and relationships to create a mathematical model (Hohenwarter, et al., , Citation2007). Teachers can use the interactive nature to create appropriate mathematical models in areas such as geometry, algebra, calculus, and trigonometry. The GeoGebra software receives geometric, algebraic, and mathematical rules and links multiple representations (Y. F. Zengin & Kutluca, Citation2012). It allows its user to track the activities performed with it. Khalil et al. (Citation2018), found that GeoGebra provides the activity process in a specific way using the construction protocol to know the whole activity. The authors also pointed out that the slider and dynamic tool can be used to design concepts. According to Doğan and İçel (Citation2011), GeoGebra is a correlative geometry system that can use points to construct vectors, segments, lines, and conics, as well as functions, while dynamically modifying them afterward.
The GeoGebra software has two views in its user interface, the Graph window and the Algebra window, which work interactively. What is displayed in the Graph window has a corresponding expression in the Algebra view and vice versa. One can use the geometry tools provided to do geometric constructions in the graphics window or an input command of functions into the input field which displays all the graphical representation of the object in the graphics window, and the associated algebraic representations are shown in the algebra window. Doğan and İçel (Citation2011) posited that one can use GeoGebra by using the algebra view, input field coordinate axes with grid, drawing pad, and many geometric tools. These algebraic tools can be accessed easily even by those who have used the software before. GeoGebra’s used can be easy for teachers if they make themselves ready and committed to learning it for teaching and learning.
The difference between students learning mathematics in the conventional teaching approach and the use of software like GeoGebra is that it helps them build a fun learning environment, which makes it necessary for the student to explore the mathematical concepts they learn (Masri et al., Citation2016). The interactive nature of it is necessary for such a learning environment and through that, collaborative learning can be encouraged. GeoGebra software application is not complex to use and provides students the opportunity to visualize ideas by the use of some concepts and graphic illustrations (Zulnaidi & Oktavika, Citation2018). Antohe (Citation2010) stressed that anyone who uses GeoGebra is required to be specific, dispassionate, must allow for experimentation and should guide any experiment. In addition to visualization, colour and animation, GeoGebra offers the ability to represent the real world in the classroom.
4. Students’ attitude and perceptions on technology in teaching and learning mathematics
The perception that students have about the teaching and learning of mathematics is a crucial aspect in determining the quality of a learning experience (Teh & Fraser, Citation1995). This helps to identify how students’ view the approach of learning and what they are learning in general. Several research (Ng & Gunstone, Citation2002; Shyu, Citation2000) investigated the impact of using technology in learning mathematics and science and came to the conclusion that technology might stimulate students to learn mathematics and science. According to Galbraith and Haines (Citation1998), not all students felt comfortable using technology, and many were skeptical about the value of technology-based learning. Although they recognize the benefits of employing technology, no student in their study stated that they preferred to learn science solely through the use of computers. When developing a learning model or instructional approach, students’ perceptions must be taken into account. Students’ perceptions, according to Ramsden (Citation1991), can be quantified using a Course Experience Questionnaire (CEQ) that focuses on performance factors such as competent teaching, clear goals and standards, acceptable workload, suitable assessment and emphasis on independence. Ginns and Ellis (Citation2007) used a questionnaire to assess student perspectives in a mixed learning environment based on good teaching, adequate resources, student interaction and acceptable workload. The perception of students on the use of GeoGebra in learning polar coordinates as a mathematical concept is the focus of this study and this was done using a Questionnaire. Attitude has been defined in various ways in literature. Attitude, according to Allport’s earlier definition, is “a mental or neural state of readiness, organized through experience, exerting a directive influence on the individual’s response to all objects and situations to or dynamic with which it is related“ (Allport, Citation1935, p. 810). It can also be defined as ”the affect of or against a psychological object” (Thurstone, Citation1931, p. 261). Despite the fact that the definition of attitude has evolved over time, all definitions emphasize a positive or negative disposition towards an object or a psychological condition. Attitude was defined by Fishbein and Ajzen (Citation1975) and Aiken (Citation2000) as a learned tendency to react positively or negatively to an object, situation, institution, or person.
The MODE model (Motivation and Opportunity as Determinants of Attitude-Behavior Relationship) defines attitude as a “memory association between an object and one’s evaluation of it” (Aiken, Citation2000, p. 101). Although it does not explicitly emphasize a favour or disfavour tendency to an object or aspect of experience, this definition shares the aspect of evaluation with other definitions. Some scales of attitude toward computers have recently been developed based on this theory (Teo & Noyes, Citation2008).
Some factors have been considered to explain the effectiveness of technology in the school curriculum and classrooms. One of the factors influencing it is student engagement. Student engagement was classified by Fredricks et al. (Citation2004, p. 60) into three categories: ‘behavioral engagement, emotional engagement, and cognitive engagement. ‘ Emotional engagement in technology refers to attitudes toward technology (Enger & Searle, Citation2008). Taking into account some theories and scales on attitudes, some studies, for example, studies like (Galbraith & Haines, Citation1998; Pierce et al., Citation2007; Vale & Leder, Citation2004), have adapted and developed attitude scales on using technology in mathematics lessons.
Pierce et al. (Citation2007, p. 288), developed the “Mathematics and Technology Attitudes Scale,” which includes subscales like mathematics confidence, confidence with technology, attitude to learning mathematics with technology, affective engagement, and behavioral engagement. However, this modified the items on the three of the subscales. This includes confidence with technology, attitude to learning mathematics with technology and affective engagement. Several researchers have focused their experimental studies on the use of technology in mathematics lessons on student achievement. However, not much has been done on students’ attitudes about technology in learning mathematics concepts. Furthermore, students’ reactions to technology are an important factor in determining how to best use mathematical tools (Pierce et al., Citation2007). As a result, identifying the students’ attitudes toward technology as part of the study’s objective, is one of the critical elements in understanding whether the students agree to use technology, thereby predicting the students’ computer-related behaviors. Attitude toward mathematics, which refers to a student’s self-reported enjoyment, interest, and level of anxiety toward mathematics (Akgül, Citation2014), is important in mathematics learning and achievement. As a result, investigating the effectiveness of instruction using GeoGebra, which may foster a positive attitude toward mathematics learning, is critical for students’ mathematics learning and achievement.
5. The effect of GeoGebra on students’ mathematics understanding, perceptions and attitude
Several studies investigate the use of GeoGebra in teaching some mathematical concepts at different levels of education. Saha, et al. (Citation2010) used a quasi-experimental design of 60 students aged 16–17 in two groups (GeoGeba group and conventional group) with spatial abilities (high-visual and low-visual) in each of the groups. Saha and his colleagues examined the effect of GeoGebra on learning coordinate geometry in students and classified them as high visual-spatial (HV) and low visual-spatial (LV) skills. It was revealed that students in the GeoGebra groups’ mean score was significantly higher than those in the conventional group. In a study conducted by Arbain and Shukor (Citation2015), a quasi-experimental design was used on 62 college students in Malaysia to determine whether student performance in statistics was affected by GeoGebra software. Their analyses of pre-test and post-test statistics problems given to students showed that GeoGebra affects students’ performance in statistics. The study also found out that students had positive attitudes in the confidence, motivation, and enthusiasm dimensions on GeoGebra. Contrary to other studies, Masri et al. (Citation2016) found that the difference between two groups’ performances in circles was not significant. This means that using GeoGebra while teaching students showed no significant difference in improving their performance compared to those who used conventional approach to study. The authors attributed this result to some insufficient factors for the implementation of the intervention. These factors have been confirmed by some studies. Shadaan and Leong (Citation2013) found that GeoGebra software improved mathematics lessons and in particular the compass allows. This conclusion was reached because students’ conceptual understanding increased significantly in the experimental group. They also revealed that GeoGebra software made the classroom cooperative and collaborative.
Emaikwu et al. (Citation2015), used a quasi-experimental group with non-equivalents, which revealed that GeoGebra improved students’ performance in statistics more than the conventional approach. It was also shown that GeoGebra group students showed greater interest in learning statistics than students in the conventional group. Khalil et al. (Citation2018) reported a finding that GeoGebra-assisted instruction had significant effects on the mathematical performance of the experimental group compared to traditional teaching methods. Previous data from students was used to select them into groups, and the next test was performed only after the surgery. In Ghana, Tay and Wonkyi (Citation2018) conducted a study on using GeoGebra to teach high school students and reported that GeoGebra software as an instructional tool provides a better understanding than teaching them without it. Khalil et al. (Citation2019), examined the mathematical thinking of students with high and low achievement in analytical geometry using GeoGebra and concluded that GeoGebra is the best tool for the development of students’ analytical geometry thinking. It was revealed that GeoGebra significantly affected both the high and low students’ in GeoGebra group compared to the conventional group. Their study used four groups: two each in experimental and control groups. They show that no matter how students’ achievement level is the uses of GeoGebra as an instructional aid that improves their achievement.
5.1. Research hypothesis/ questions
One research question and one hypothesis was used for the study:
There is no significant difference in the mean scores of students taught polar coordinate with the aid of GeoGebra and those taught without the aid of GeoGebra (tested at a 5% level of significance).
What are university students’ perceptions and attitudes on the uses of GeoGebra as a tool for learning polar coordinates?
6. Methods
This study used a quantitative method that adopted a quasi-experimental design because of its characteristics of “providing the best approach to investigate cause and effect relationships” (McMillan, Citation2000). Frankel and Wallen (Citation2010) argue that quasi-experimental design is a means of establishing cause and effect relationships. Two equivalent groups were involved in this study, the experimental group was taught using the GeoGebra software in addition to the conventional method, and the control group was taught using the conventional approach only.
The level 200 Mathematics Education students of the Akenten Appiah-Menka University of Skills Training and Entrepreneurial Development (a public university in Ghana) for the 2021/2022 academic year was selected as the population of the study. They were five hundred and eleven (511) in number. A power analysis was performed to identify a significant difference between the two groups. Based on past literature, a two-tailed effect size of d = 0.8 and a two-tailed α = 0.05 was assumed. The calculation was performed by running a G*Power software program as proposed by Faul et al. (Citation2009) to estimate the sample size. The other parameters used for the calculation were the family of tests: t-tests; Statistical test: Means: difference between two independent means (two groups); Type of power analysis: A priori: Calculate required sample size—given α, power and effect size; Power: = 0.8; and allocation ratio : this gave a sample of 84 A G*Power software program as suggested by Faul et al. (Citation2009). This was used to calculate the sample size of 84 students. The students were randomly selected from the population as participants for the study. The participants comprised of both gender (Male = 54 and female = 30). The participants were then assigned to either control or experimental group using a purposive sampling technique. Participants with a computer (this was determined from the consent form) were purposively assigned to the experimental group because the computer was a key material for the experimental group. The participant own computer was need because, the availability of the university’s computer could not be assured and to also the participants to get opportunity of practicing on what have been discussed whiles at home.
Achievement tests on polar coordinates and questionnaires measuring perceptions and attitudes were used as the instrument for data collection. The achievement test was a modification of the Polar Coordinate Knowledge Test (PCKT) and achievement test on polar coordinates used in studies by Y. Zengin and Tatar (Citation2015) and Alabdulaziz et al. (Citation2020) respectively. Trust with Technology (TC); Attitude to learning mathematics with technology (MT); and the affective involvement (AE) aspect were adapted from the whole scale of mathematical and technological attitudes (MTAS) developed by Pierce et al. (Citation2007). Student perceptions questionnaire was also adapted from a study by Shadaan and Leong (Citation2013). The questionnaire contained 10 statements for each construct, which was measured on a five-point Likert scale ranging from strongly disagree (1) to strongly agree (5). Students consent was sought by giving them informed consent form to fill before their participation in the study. The study also sought the consent of the Department of Mathematics in the university through permission letter.
The reliability of the test tools was verified using the instrument pilot data. Due to the nature of the test (both multiple choice and essay), the split half-reliability method using the Spearman-Brown procedure was used (Webb et al., Citation2006). A reliability coefficient of 0.85 and 0.77 for pre-test and post-test respectively were calculated using this approach, which was greater than 0.60 threshold for acceptable test instrument to be used (Gay, & Felding-Habermann, Citation2011). The reliability analyses on data obtained from the respondents after the administration of questionnaire on students’ perception and attitude showed a Cronbach’s alpha of ∝ = 0.888 and ∝ = 0.817 for perceptions and attitudes respectively.
The data collected on the participant using the test was entered into SPSS v. 21; the analysis of mean scores for the two groups was performed using an independent t-test and a paired sample t-test. Data obtained on students’ perception and attitude on learning of polar coordinates with GeoGebra was analysed by using descriptive statistics (mean and standard deviation).
7. Results
7.1. Preliminary analysis
In a parametric test, there are basic assumptions about the distribution of the populations. The assumptions for conducting a t-test require that: data values are measured on a scale level, that sample is randomly selected, that the data is normally distributed, that it uses appropriate sample size and homogeneity of variance for the two groups (Kim & Park, Citation2019). Two tests of normality (Kolmogorov-Smirnov and Shapiro-Wilk) were conducted (see, Table ).
Table 1. Test for normality across groups for various tests
From the two tests, the null hypothesis is rejected (p < 0.05) for the tests in both groups and concludes that statistically there is no evidence of distribution of normality of the tests scores.
For the homogeneity of variance, Levene’s test for equality of variance was conducted for all the various independent groups (see, Table ).
Table 2. Levene’s test for equality of variance on test scores for various independent groups
Levene’s test revealed that p > 0.05 for pre-tests in the control groups. Hence, equal variance is assumed for this test. It was also revealed that p < 0.05 for post-tests in the experiment. This also shows that equal variance is not assumed for this test. In the independent t-test of post-test for the control and experimental group, the equal variance not assumed row of the values is reported in such circumstance.
Some of the assumptions required for conducting t-tests have not been met. However, Field (2013) intimated that, the assumption of normality and homogeneity of variance can be violated in conducting a t-test by running it with Bootstrapping for a sample size of more than 30. Based on this, the t-test was run by performing Bootstrapping in SPSS version 21, and the results are shown in the following sections.
7.2. The difference in the mean scores of students taught polar coordinates with and without the aid of GeoGebra software
In each of the groups, the pre-test and post-test scores of students were compared by conducting a paired sample t-test in each case. The groups’ performance in both tests was compared using an independent t-test. The comparison of students’ performance in the pre-test to see the performance level of the students (see, Table ).
Table 3. Independent samples t-test for both groups’ pre-test scores
Table shows that the mean for the experiment group was 6.24 and that of the control group was 5.07. A standard deviation of 3.45 and 2.70 was obtained for the experimental and control groups respectively. However, at p = 0.09 > 0.05 with a mean difference of 1.17. This shows that there was no significant difference in between the pre-test performance of the two groups. This shows that the experimental group’s performance in the pre-test was statistically not different from those in the control group. Each group’s performance in the pre-test and the post-test was also analysed by conducting a paired sample t-test (see, Table ).
Table 4. Paired sample t-test for pre-test and post-test in both groups
The results in Table reveal that the difference of 2.69 and 8.26 in the mean score of the pre-test and post-test in the control group and experimental group respectively. The t-test (t = −3.62, p < 0.05) shows that there was a significant difference between the mean scores of the control group in the two test. For the experimental group, the t-test (t = −3.62, p < 0.05), indicates a significant difference between the mean scores obtained in the two tests. To determine the difference between the groups after the treatment had been implemented, an independent t-test analysis was done on the post-test scores of the two groups and the results are presented in Table .
Table 5. Independent sample t-test for experimental and control groups post-test scores
From Table , the control group had a mean of 7.76 whilst the experimental group had 14.50 on a score of 20 as the maximum. The difference in the mean was 6.74 with a t-statistic of 8.82 and a p-value of 0.00. Since the p ≤ 0.05, it shows that there was a statistically significant difference between the post-test score for the two groups. Cohen’s effect size of 1.92 suggests that the experimental group students performed better in the post-test than the control group.
7.3. Students’ perception and attitude on the uses of GeoGebra as a tool for learning polar coordinates
The responses from students on the Likert-scale questionnaire items on perception and attitude were analysed by using descriptive statistics, thus mean and standard deviation of the responses to the items. The results of students’ responses to the items on attitude toward the uses of GeoGebra in learning polar coordinates are presented in Table .
Table 6. Descriptive statistics on students’ attitude toward the uses of GeoGebra
From Table , the lowest mean was 3.67, and a standard deviation of 0.874 represents the response for the statement “I am good at using technological tools that can teach maths. This means that students” level of agreement was skewed towards agreement. The highest mean was recorded for the statement ‘Polar coordinate is more interesting when using GeoGebra, which is 4.64. The overall mean of 4.24 is an indication that students generally agree with the statements on the attitude towards the uses of GeoGebra in learning polar coordinates. This shows that the students in the group that used the GeoGebra software to learn polar coordinates expressed a positive attitude.
Table shows the results from the descriptive analysis of students’ responses to statements on their perception of the uses of GeoGebra in the polar coordinate lesson.
Table 7. Descriptive statistics on students’ perception of the uses of GeoGebra
The results from Table shows that students’ responses to all the perception statements were generally in agreement with them (Overall Mean = 4.19). This shows that students agreed with positive statements about the GeoGebra software. The lowest mean was on the statement I felt confident using the GeoGebra software during activities in class, which was 3.81. The highest mean of 4.48 was obtained for the statement I prefer to learn mathematics with GeoGebra. This indicates that students agreed to learning polar coordinates with GeoGebra than learning it through the conventional approach only. It can be concluded that students had an increase in their interest and confidence in learning polar coordinates with GeoGebra software.
8. Discussion
Since the results of the analysis of the pre-test for the two groups indicated that their performance was not significantly different, then it can be said that there was not any difference between the achievement of students in the experimental and controlled in polar coordinates before the treatments. However, the results in the analysis of the post-test indicated that students exposed to GeoGebra software in the learning of polar coordinates obtained higher average scores as compared to students in the control group. This can be due to the fact that the software helped students in the experimental group in checking the accuracy of their task in learning polar coordinates.
The use of GeoGebra in polar coordinate lessons allowed the students to work on more examples, especially when sketching polar curves and converting polar points to the Cartesian point and vice-versa. This could not be said about students who did not use GeoGebra and worked with few examples and did not have the opportunity of checking the correctness of their learning. When it comes to the sketching of polar curves, the teaching of it was limited to a few examples because the conventional approach consumes space and time. This applies to Ljajko and Ibro (Citation2013) position that GeoGebra gives students the chance to explore in real-time, which helps to improve the speed and quality of the learning process. GeoGebra gave students in the experimental group the opportunity to abreast themselves with the types and nature of polar curves. The uses of GeoGebra in the learning of polar coordinates involved a lot of cooperation and interactions, which gave them more skills in the use of the software and the opportunity to guide their peers in grasping the concept as supported by the social constructivist on the theory of Zone of Proximal Development.
This finding is consistent with several studies in the literature. Saha, et al. (Citation2010) found that the performance of high visual ability students was significantly higher than that of low visual ability students in the experimental and control group. This is similar to the finding in this study. Their finding suggested that regardless of the visual ability of students, GeoGebra helps to increase their understanding of coordinate geometry. This finding in this study is consistent with Shadaan and Leong (Citation2013), however, their study focused on how GeoGebra increases students’ conceptual understanding of circles. It was also revealed that apart from GeoGebra increasing the conceptual understanding of circles, it was also an enabler of the mathematics classroom, which made the classroom cooperative and collaborative. This study also established it as an enabler for students’ cooperation.
In this study, students’ understanding of polar coordinates increased for those in the Geogebra group more than those in the conventional group. This was also in agreement with Zulnaidi and Oktavika (Citation2018) who found that GeoGebra software could lower the level of students’ misconceptions about the limit of functions. Their study was not focused on students’ achievement; however, the analysis of both tests revealed that students in the GeoGebra had low misconception on the limit of a function. In the present study, the experimental group was able to get a conceptual understanding better than the control group, especially the concept of polar curves which was reflected in their performance. Ocal (Citation2017) investigated the conceptual and procedural knowledge of the application of derivatives on students and revealed that there was not any significant difference between the groups on procedural knowledge. However, there was a statistically meaningful difference between the experimental and control groups’ conceptual understanding. GeoGebra helped improve students’ conceptual understanding of polar coordinates, which is reflected in the performance in the polar coordinates test.
Students’ responses to the statements on their perceptions and attitudes on the uses of GeoGebra software in learning polar coordinates showed that they had high interest and confidence in using the software. This finding is consistent with Arbain and Shukor (Citation2015) revelation on Malaysian secondary school students that GeoGebra not only increases their performance, but also increases their interest and enjoyment level in using technology in learning mathematics. Learning with GeoGebra can ignite interaction on task. Emaikwu et al. (Citation2015) found that the average rating of students’ interest in the GeoGebra group was higher as compared to the conventional group. GeoGebra aroused students’ interest in learning statistics. However, in this study, only students in the GeoGebra group’s perception were found, and it was very positive and affects the students’ interest. Shadaan and Leong (Citation2013) also revealed that students provided positive feedback on the GeoGebra software. About 82% of the students made an indication of their excitement about using GeoGebra software, whilst 75% of students mention their ability to think creatively and critically in the learning process. All these are in line with a positive perception towards the teaching method of using GeoGebra in teaching and learning polar coordinates in the present study.
9. Conclusion and Recommendations
The findings from the study have a range of implications for mathematics education. Mathematics teachers at all levels including university lecturers should be encouraged to integrate GeoGebra or similar technological software capable of teaching and learning mathematics concepts. This will not only help increase students’ achievement in the mathematics content but also develop their interest and positive attitudes toward learning the particular content and mathematics in general. University mathematics students are encouraged to integrate the dynamic mathematics software, GeoGebra, into their learning to improve their understanding of the numerous mathematics concept they are studying. The findings in this study have implications for the instruction of mathematics. The Mathematics Departments in the various universities and tertiary institutions in Ghana should evaluate the content of the various mathematics courses and make suggestions for the integration of an appropriate technological tool for teaching mathematics in areas challenging to students. The number of ICT courses taken by mathematics students that focus on the use of technological tools for mathematics instructions should be more in the mathematics programme structure for institutions that have such mathematics programmes and those institutions that do not have such programmes should be encouraged to create some.
This study used two equivalent groups selected randomly from the population of a one-year group in selected universities, a study using intact classes from universities using more than two groups of students can be done to expand the scope. In addition, this study considered students in the experimental groups’ attitudes and perceptions concerning the usage of the GeoGebra in learning polar coordinates only. Further studies can be done to examine both the control and experimental groups with respect to their test achievements.
Disclosure statement
No potential conflict of interest was reported by the author(s).
Additional information
Funding
References
- Aiken, L. R. (2000). Psychological testing and assessment (10th ed.). Allyn and Bacon. https://books.google.com.gh/books?id=R1l9AAAAMAAJ
- Akgül, M. B. (2014). The effect of using dynamic geometry software on eight grade students’ achievement in transformation geometry, geometric thinking and attitudes toward mathematics and technology y [Master’s thesis, Middle East Technical University]. http://etd.lib.metu.edu.tr/upload/12616880/index.pdf
- Alabdulaziz, M. S., Aldossary, S. M., Alyahya, S. A., & Althubiti, H. M. (2020). The effectiveness of the GeoGebra programme in the development of academic achievement and survival of the learning impact of the mathematics among secondary stage students. Education and Information Technologies, 26(3), 2685–16. https://doi.org/10.1007/s10639-020-10371-5
- Allport, G. W. (1935). Attitudes: A handbook of social psychology, Worcester, mass. Clark University Press.
- Antohe, V. N. (2010). New methods of teaching and learning mathematics involved by GeoGebra. Third international conference on innovations in learning for the future 2010: e-Learning (pp. 1–8). Istanbul: TC Istanbul University. https://www.researchgate.net/profile/
- Arbain, N., & Shukor, N. A. (2015). The effects of GeoGebra on students achievement. Procedia - Social and Behavioral Sciences, 172, 208–214. https://doi.org/10.1016/j.sbspro.2015.01.356
- Coolidge, J. L. (1952). The Origin of Polar Coordinates. The American Mathematical Monthly, 59(2), 78–85. https://doi.org/10.1080/00029890.1952.11988074
- Dikovic, L. (2009). Applications GeoGebra into teaching some topics of mathematics at the college level. Computer Science and Information Systems, 6(2), 191–203. https://doi.org/10.2298/CSIS0902191D
- Doğan, M., & İçel, R. (2011). The role of dynamic geometry software in the process of learning: GeoGebra example about triangles. International Journal of Human Sciences. http://www.InsanBilimleri.com/En
- Emaikwu, S. O., Iji, C. O., & Abari, M. T. (2015). Effect of GeoGebra on senior secondary school students’ interest and achievement in statistics in Makurdi local government area of Benue State, Nigeria. Journal of Mathematics (IOSRJM), 2(3), 14–21. https://www.researchgate.net/profile/Terseer-Abari/publication/357165114_
- Enger, A. J., & Searle, D. (2008). Students in a digital age: Implications of ICT for teaching and learning. In International handbook of information technology in primary and secondary education (pp. 63–80). Springer. https://doi.org/10.1007/978-0-387-73315-9_4
- Faul, F., Erdfelder, E., Buchner, A. & Lang, A. (2009). Statistical power analyses using G*Power 3.1: Tests for correlation and regression analyses. Behavior Research Methods, 41(4), 1149–1160. https://doi.org/10.3758/BRM.41.4.1149
- Fishbein, M., & Ajzen, I. (1975). Belief, attitude, intention, and behavior: An introduction to theory and research. Reading (MA). Addison-Wesley.
- Frankel, N., & Wallen, E. (2010). Student Research companion: How to design and evaluate research in education. New York: McGaw-Hill.
- Fredricks, J. A., Blumenfeld, P. C., & Paris, A. H. (2004). School engagement: Potential of the concept, state of the evidence. Review of Educational Research, 74(1), 59–96. https://doi.org/10.3102/00346543074001059
- Galbraith, P., & Haines, C. (1998). Disentangling the nexus: Attitudes to mathematics and technology in a computer learning environment. Educational Studies in Mathematics, 36, 275–290. https://doi.org/10.1023/A:1003198120666
- Gay, L. J. & Felding-Habermann, B. (2011). Contribution of platelets to tumour metastasis. Nat Rev Cancer, 11(2), 123–134. https://doi.org/10.1038/nrc3004
- Ginns, P., & Ellis, R. (2007). Quality in blended learning: Exploring the relationships between on-line and face-to-face teaching and learning. Internet and Higher Education, 10, 53–64. https://doi.org/10.1016/j.iheduc.2006.10.003
- Hohenwarter, M., & Jones, K. (2007). Ways of linking geometry and algebra: The case of geogebra. British Society for Research. BSRLM Geometry Working Group. http://eprints.soton.ac.uk/id/eprint/50742
- Kamariah, A. B., Ahmad Fauzi, M. A., Wong, S. L., & Rohani, A. T. (2010). Exploring secondary school students’ motivation using technologies in teaching and learning mathematics. Rocedia Social and Behavioral, 4650–4654. https://doi.org/10.1016/j.sbspro.2010.03.744
- Khalil, M., Farooq, R. A., Çakıroğlu, E., Khalil, U., & Khan, D. M. (2018). The development of mathematical achievement in analytic geometry of grade-12 students through GeoGebra activities. Eurasia Journal of Mathematics, Science and Technology Education, 14(4), 1453–1463. http://doi.org/10.29333/ejmste/83681
- Khalil, M., Khalil, U., & Haq, Z. (2019). Geogebra as a scaffolding tool for exploring analytic geometry structure and developing mathematical thinking of diverse achievers. International Electronic Journal of Mathematics Education, 14(2), 427–434. https://doi.org/10.29333/iejme/5746
- Kim, T. K., & Park, J. H. (2019). More about the basic assumptions of t-test: Normality and sample size. Korean Journal of Anesthesiology, 331–335. https://doi.org/10.4097/kja.d.18.00292
- Ljajko, E., & Ibro, V. (2013). DEvelopment of ideas in a GeoGebra-aided mathematics instruction. Mevlana International Journal of Education (MIJE), 3(3), 1–7. https://eric.ed.gov/?id=ED544150
- Masri, R. M., Ting, S. H., Zamzamir, Z., & Ma’amor, R. L. (2016). The effects of using GeoGebra teaching strategy in Malaysian secondary schools: A case study from Sibu, Sarawak. Geografia-Malaysian Journal of Society and Space, 12(7). https://www.researchgate.net/profile
- McMillan, A. (2000). Research in education: Evidence based inquiry. Pearson Education Limited. https://www.researchgate.net/profile/Norain-Mohd-Tajudin/publication/303876551_
- Montiel, M., Wilhelmi, M. R., Vidakovic, D., & Elstak, I. (2009). Using the onto-semiotic approach to identify and analyze mathematical meaning when transiting between different coordinate systems in a multivariate context. Educational Studies in Mathematics, 72(2), 139–160. https://doi.org/10.1007/s10649-009-9184-2
- National Council of Teachers of Mathematics. (2003). Principles and standards for school. In Reston. NCTM.
- Ng, W., & Gunstone, R. (2002). Students’ perceptions of the effectiveness of the World Wide Web as a research and teaching tool in science learning. Research in Science Education, 32, 489–510. https://doi.org/10.1023/A:
- Ocal, M. F. (2017). The effect of geogebra on students’ conceptual and procedural knowledge: The case of applications of derivative. Higher Education Studies, 7(2), 67. https://doi.org/10.5539/hes.v7n2p67
- Oral, E., Wannomae, K. K., Hawkins, N., Harris, W. H., & Muratoglu, O. K. (2004). α-Tocopherol-doped irradiated UHMWPE for high fatigue resistance and low wear. Biomaterials, 25(24), 5515–5522. https://doi.org/10.1016/j.biomaterials.2003.12.048
- Paoletti, T., Moore, K. C., Gammaro, J., & Musgrave, S. (2013). Students’ emerging understandings of the polar coordinate system. The sixteenth annual conference of research in undergraduate mathematics education (pp. 366–380). https://www.researchgate.net
- Pierce, R., Stacey, K., & Barkatsas, A. N. (2007). A scale for monitoring students’ attitudes to learning mathematics with technology. Computers & Education, 48(2), 285–300. https://doi.org/10.1016/j.compedu.2005.01.006
- Ramsden, P. (1991). A performance indicator of teaching quality in higher education: The course experience questionnaire. Higher Education Studies, 16, 129–150. https://doi.org/10.1080/03075079112331382944
- Saha, R. A., Ayub, A. F. & Tarmizi, R. A. (2010). The Effects of GeoGebra on Mathematics Achievement: Enlightening Coordinate Geometry Learning. Procedia - Social and Behavioral Sciences, 8, 686–693. https://doi.org/10.1016/j.sbspro.2010.12.095
- Sayre, E., & Wittman, M. (2007). Intermediate mechanics students’ coordinate system choice. In electronic proceedings for the tenth special interest group of the mathematical association of America on Research in Undergraduate Mathematics Education Conference on Research in Undergraduate Mathematics Education. https://d1wqtxts1xzle7.cloudfront.net/30797423/sayre-wittmann-with-cover-page-
- Shadaan, P. & Leong, K. E. (2013). Effectiveness of using GeoGebra on students' Understandiing in learning Cricle.The Malaysian Online Journal of Educational,1(1), 1–11. EISSN-2289-2990.
- Shulman, L. S. & Carey, N. B. (1984). Psychology and the Limitations of Individual Rationality: Implications for the Study of Reasoning and Civility. Review of Educational Research, 54(4), 501–524. https://doi.org/10.3102/00346543054004501
- Shyu, H. Y. (2000). Using video-based anchored instruction to enhance learning: Taiwan’s experience. British Journal of Educational Technology, 31(1), 57–69. https://doi.org/10.1111/1467-8535.00135
- Sivan, E. (1986). Motivation in social constructivist theory. Educational Psychologist, 21(3), 209–233. https://doi.org/10.1111/1467-8535.00135
- Suleman, A. H. (2012). Challenges facing the teaching and learning of mathematics in this technological age. The keynote address of the MAG 38th national annual conference. In Workshop. WA, Ghana.
- Tay, M. K., & Wonkyi, T. M. (2018). Effect of using Geogebra on senior high school students’ performance in circle theorems. African Journal of Educational Studies in Mathematics and Sciences, 14, 1–18. https://www.ajol.info/index.php/ajesms/article/view/173391
- Teh, G. P. L., & Fraser, B. J. (1995). Development and validation of an instrument for assessing the psychosocial environment of computer-assisted learning classrooms. Journal of Educational Computing Research, 12, 177–193. https://doi.org/10.2190/PKXE-VTTA-4PTA-B
- Teo, T., & Noyes, J. (2008). Development and validation of a computer attitude measure for young students. Computers in Human Behaviour, 24, 2659–2667. https://doi.org/10.1016/j.chb.2008.03.006
- Thurstone, L. L. (1931). Measurement of social attitudes. Journal of Abnormal and Social Psychology, 26, 249–269. https://psycnet.apa.org/doi/10.1037/h0070363
- Vale, C., & Leder, G. (2004). Student views of computer-based mathematics in the middle years: Does gender make a difference? Educational Studies in Mathematics, 56, 287–312. https://doi.org/10.1023/B:EDUC.0000040411.94890.56
- Webb, N. M., Shavelson, R. J., & Haertel, E. H. (2006). Reliability coefficients and generalizability theory. Handbook of Statistics, 26, 81–124. https://doi.org/10.1016/S0169-7161(06)
- Wood, D. (1999). Social interaction as tutoring. Lev Vygotsky: Critical Assessments, 4, 282–303. https://books.google.com.gh/books?
- Zengin, Y. F., & Kutluca, T. (2012). The effect of dynamic mathematics software geogebra on student achievement in teaching of trigonometry. Procedia-Social and Behavioral Sciences, 31(183–187). https://doi.org/10.1016/j.sbspro.2011.12.038
- Zengin, Y., & Tatar, E. (2015). The teaching of polar coordinates with dynamic mathematics software. International Journal of Mathematical Education in Science and Technology, 46(1), 127–139. https://doi.org/10.1080/0020739X.2014.904529
- Zulnaidi, H., & Oktavika, E. (2018). The effect of geogebra on students’misconceptions of limit function topic. JuKu: Jurnal Kurikulum & Pengajaran Asia Pasifik, 6(1), 1–6. http://jice.um.edu.my/index.php/JUKU/article/view/11168