Abstract
The aim of the study was to evaluate the effects of diabetes on episodic memory and working memory after controlling for other comorbidities and several demographic and biological variables. We conducted a cross-sectional study with 1656 participants. From this sample, 100 were diagnosed with type 2 diabetes mellitus. This group was compared with participants without diabetes matched by age, education and sex. Hierarchical regression analyses revealed that after controlling for demographic variables (age, sex, years of education, vocabulary scores, Mini-Mental State Exam scores and Beck Depression Inventory scores) and biological variables (body mass index, glucose, cholesterol, triglycerides, heart rate, systolic blood pressure and diastolic blood pressure), the diabetes status significantly predicted recollection (β = .62), recognition (β = .28), verbal working memory in low (β = .39) and high (β = .29) difficulty tasks, spatial working memory in low (β = .32) and high (β = .38) difficulty tasks, and speed in verbal high difficulty tasks (β = −.33) and spatial low difficulty (β = −.30) working memory tasks. The most essential memory processes for autonomous everyday living are widely affected in diabetic individuals free from other comorbidities despite the administration of glycemic medication.
PUBLIC INTEREST STATEMENT
Previous studies have identified that individuals affected with diabetes experience cognitive decline. However, these studies have evaluated cognition through neuropsychological tests that provide information about global cognition or general functioning in one particular domain. Therefore, our study contributes to the field by characterizing the effects of diabetes on complex cognitive domains, such as memory. In particular, we examined episodic memory and working memory, the types of memory more affected with advancing age, through computerized tasks. Moreover, the use of computerized tasks allowed us to accurately measure the speed of memory processing, which is a variable that has been practically unexplored in individuals affected by diabetes. We provided evidence that both episodic memory and working memory decline in participants with diabetes compared to individuals without diabetes after controlling for several comorbidities, demographic factors and biological variables. These outcomes highlight the need to improve control of this medical condition.
1. Introduction
Milles and Root (Citation1922) published the first study providing evidence that diabetic individuals performed lower in memory and attention tasks than persons without diabetes. Since then, numerous studies have been conducted to further identify which cognitive processes are impaired in diabetes mellitus type 2 (referred to as diabetes hereafter) and to estimate the magnitude of those impairments (for meta-analyses, see Monette et al., Citation2014; Palta et al., Citation2014). The majority of these studies have found modest deficits in several cognitive domains (Monette et al., Citation2014; Palta et al., Citation2014).
However, the cognitive domains in these studies were evaluated through dissimilar neuropsychological tests that are frequently used to assess mixed processes within each domain; hence, the great variability of results among studies does not allow for the identification of the specific processes within each domain that may be affected by diabetes; thus, only general conclusions about the affected cognitive domains have been proposed. For example, the Rey Auditory Verbal Learning Test has been the most frequently used test to measure verbal memory in studies about diabetes (Palta et al., Citation2014). This procedure assesses immediate and delayed memory, learning strategies, interference control, confusion or confabulation (Lezak, Citation1995), and composite measures of this test are often used (e.g., Hugenschmidt et al., Citation2013). Moreover, paper-and-pencil neuropsychological tests do not allow for an examination of the speed of mental processing. Therefore, our knowledge about the effects of diabetes on cognition still lacks precision.
The aim of the present study was to exclusively examine the effects of diabetes on memory to provide detailed evidence of the actual processes that are affected within this domain. We focus on working memory and episodic memory because they are the most vulnerable types of memory to the effects of age (Daselaar et al., Citation2007) or illness (Budson & Price, Citation2005). To examine these types of memory, we employed computerized tasks that allowed us to precisely estimate the accuracy and speed of memory processing.
Working memory is the mental ability employed most of the time because it allows us to manage our current perceptions and memories for a brief period of time to perform multiple cognitive functions, such as reasoning and solving problems. Therefore, working memory is essential for dealing with everyday living situations. We examined working memory with the n-back task (Kirchner, Citation1958) in verbal and spatial modalities, and each modality had two levels of difficulty. The task consists of judging whether the current item is equal to the item presented one trial before (low difficulty: 1-back) or two trials before (high difficulty: 2-back). The n-back task evaluates the main processes involved in working memory: storage, binding each item to its temporal order, retrieval, updating the item and its temporal order, and monitoring and controlling interference from items that do not correspond to the specific lag under evaluation.
Episodic memory is the mental ability that allows us to recall personal experiences and is characterized by a subjective sense of time (chronesthesia) (Tulving, Citation2002) and by the consciousness of existing in our subjective time (autonoetic) (Tulving, Citation1985). The experience of identity and self-continuity depends on these properties (Tulving, Citation1985); therefore, episodic memory is of utmost importance because it provides us with the sense of a unique personal history, which is essential for autonomous living. Within episodic memory, two processes have been identified: familiarity and recollection (Mandler, Citation1980). Familiarity is the ability to recognize that an event has occurred before, although further details of the event are not retrieved, whereas recollection is the ability to retrieve the event and the relevant details associated with the event, such as the moment and place where it took place. Recollection is vulnerable to aging and illness, although selective familiarity deficits have also been reported (Yonelinas et al., Citation2010). Therefore, to truly investigate the effects of diabetes on episodic memory, it is essential to use a procedure that can distinguish recollection from familiarity. We employed a source memory task (Cansino et al., Citation2002) that allows us to measure recollection because after randomly displaying several images in the quadrants of the screen, the images are presented in the center of the screen and participants must report the exact quadrant in which each image was displayed. Thus, this procedure objectively evaluates the ability to retrieve the details of a previous experience. Familiarity within this procedure corresponds to incorrect source responses; however, these values do not provide further information because they are the counterpart of correct source responses. Therefore, we report recognition, i.e., old images correctly identified as old, in which familiarity and recollection are mixed.
Memory, assessed through different neuropsychological tests, has been identified as one of the cognitive domains more affected in diabetic individuals than in persons without diabetes (Awad et al., Citation2004; Biessels et al., Citation2008). However, memory has also been shown to be almost unaffected or spared in diabetic individuals (Asimakopoulou et al., Citation2002; Van Harten et al., Citation2007). Episodic memory in particular, measured with an immediate and delayed word recall list, was shown to decrease in a longitudinal study (Pappas et al., Citation2017) that followed a sample of 950 individuals affected by diabetes for six years. The most frequent task that has been used to examine working memory in studies about diabetes is digit span forward and backward tests (Palta et al., Citation2014). Working memory performance in persons with diabetes is worse than that in persons without diabetes, with low (Palta et al., Citation2014) to moderate (Monette et al., Citation2014) effect sizes.
In the present study, memory performance in participants diagnosed with diabetes was compared with that in participants not affected with this disease. To control for comorbidities, participants from both groups were free of hypertension, hypotension, hypercholesterolemia or hypertriglyceridemia. Moreover, hierarchical regression analyses were conducted to control for demographic and biological variables that may influence working memory and episodic memory performance; therefore, the exact variation explained by diabetes on memory performance was estimated. As in previous studies, we expected that diabetic participants would perform lower than nondiabetic individuals in both types of memory but that this performance would be worst for recollection processes in episodic memory and for highly difficult tasks in working memory. This prediction is based on the fact that tasks that require greater effort would be more difficult to perform in individuals affected with diabetes because, as observed in the aging process, fewer resources are available (Luo & Craik, Citation2008). Previous studies with diabetic individuals have not systematically examined the effects of task difficulty on memory performance. The integrity of white matter is important for fast memory processing; however, white matter lesions have been observed in diabetic patients (Biessels & Despa, Citation2018). Thus, we expected that memory processing would be slower in diabetic participants than in nondiabetic individuals.
2. Methods
2.1. Participants
A total of 1656 adults between the ages of 21 and 80 initially participated in this study, and data were collected over 6.5 years. The present analysis represents part of a large-scale study named “Influence of quality of life on the ontogenetic development of memory”; to date, approximately ten articles have been published in relation to the study, although none of these papers focused on diabetes. In the original sample, 148 participants were diagnosed with type 2 diabetes mellitus. Individuals who were also diagnosed with hypertension, hypotension, hypercholesterolemia or hypertriglyceridemia were excluded from this group. This procedure allowed for the formation of a group of 100 individuals exclusively affected by diabetes. Of the remaining sample, those who were free of hypertension, hypotension, hypercholesterolemia and hypertriglyceridemia were eligible for inclusion in the nondiabetic group. For each diabetic participant, a nondiabetic individual was randomly selected to avoid any bias selection after matching by sex, age and years of education. The characteristics of both groups are displayed in . All diabetic individuals were receiving glycemic control medication.
Table 1. Participant characteristics and scores on neuropsychological tests for the diabetic and nondiabetic groups
The entire sample was recruited through advertisements, flyers, appeals to community groups and word of mouth. The inclusion criteria were a minimum of eight years of education, normal or corrected-to-normal vision, a score ≤ 20 on the Beck Depression Inventory (BDI) (Beck, Citation1987), a score ≥ 24 on the Mini-Mental State Exam (MMSE) (Folstein et al., Citation1975), and a score ≥ 26 on the vocabulary subtest of the Wechsler Adult Intelligence Scale-Revised (WAIS-R) (Wechsler, Citation1981). These performance scores were required to ensure that participants were not suffering from depression, dementia, or intellectual difficulties. The exclusion criteria were addiction to drugs or alcohol, consumption of medication that acts on the nervous system in the previous six months, neurological or psychiatric diseases, or head trauma. All participants provided informed consent and received a monetary reward for their participation. The study was approved by the Bioethics Committee of the School of Medicine at the National Autonomous University of Mexico (124–2011). All experiments were performed in accordance with the Declaration of Helsinki.
2.2. Stimuli
A set of 122 color images of common objects were used in the source memory task. The images were taken from the internet and edited to have the same resolution and size.
Half of the images represented natural objects (e.g., dog or apple), and the remaining images represented artificial objects (e.g., chair or hat). The images subtended a vertical and horizontal visual angle ranging from 2.9° to 4.3° and were displayed on a white background screen. Because 12 images were employed in a practice session and 2 were presented at the beginning of the encoding and retrieval tasks, data from these 14 were not analyzed. During the encoding phase, each participant was presented with a different set of 72 randomly selected images from among the 108 available images, with each set containing the same proportion of natural and artificial objects. For the retrieval task, the complete set of 108 images was randomly presented, 36 of which were new images.
Twelve uppercase letters (B, F, G, K, L, N, P, Q, R, S, T and X) were used in the verbal version of the n-back task (vertical and horizontal visual angles of 1.5° and 1°, respectively) and presented at the center of the screen. For the spatial n-back task, a circle (visual angle diameter of 1.5°) was displayed in one of 12 possible positions around the center of the screen. A black cross (vertical and horizontal visual angles of 0.5°) was continuously displayed at the center of the screen. The distance between the circle and the center of the screen was 4°. The letters for the verbal task and the positions for the spatial task were selected randomly and with the same probability. All stimuli were presented in a dark gray color on a white screen to maintain a low contrast.
2.3. Procedure
During recruitment, prescreening questions were asked to address the specific inclusion and exclusion criteria. Only potential participants were invited to partake in the first session. Each participant partook in two sessions that each lasted two hours. The first session took place in a silent room, and only the participant and the experimenter were present. During this session, the participants were interviewed to ensure that they satisfied the inclusion and exclusion criteria, psychological tests were administered, and the participants’ vision was tested. If the participants were eligible for the study, informed consent was obtained. Afterwards, the participants were further interviewed about their health and other aspects of their lifestyle. Their health status was examined for the following systems: nervous, respiratory, cardiovascular, immune, sensory, digestive, renal, reproductive, endocrine, musculoskeletal, hepatic portal, and sleep. Participants were requested to report any diseases that had been formally diagnosed by a physician in each of the above systems during their lifetime, the age at which each disease started and the treatment they received. Finally, the participants’ weight and height were measured.
During the second session, participants’ glucose, cholesterol and triglyceride levels were measured in a nonfasting state with an Accutrend Plus System, Roche Diagnostics, Rotkreuz, Switzerland. These measurements were taken in a counterbalanced order. Blood pressure and heart rate were measured twice, approximately half an hour apart, with a Hem-712 C digital upper arm sphygmomanometer (Omron, Kyoto, Japan). The averages of both measures were included in the data analysis. The participants performed the working memory task and the source memory task, which consisted of an encoding and a retrieval phase, in a sound-damped chamber. The participants were seated in a high-back armchair 100 cm away from the monitor screen. The response panel was located on a platform on the arm of the chair at a comfortable distance from the left or right hand according to the participants’ handedness. The response panel consisted of five keys adapted for right- or left-handed participants. Four keys were arranged in two columns of two rows each so they could be pressed by the index and middle finger. The four keys represented the quadrants of the screen where the images were presented during the encoding phase. The fifth key was located in the lower portion of the response panel so it could be pressed by the thumb. Only the two keys in the second row were used during the encoding phase and the working memory tasks, while all five keys were used during the retrieval phase. The participants learned how to use the response panel during a training period that involved a shorter version of the encoding and retrieval tasks. The stimuli presentation and response recording were controlled by the software E-Prime v1.0 from Psychological Software Tools, Pittsburgh, PA, USA.
2.3.1. Source memory paradigm
During the encoding phase, the screen was divided into quadrants by a centered cross. Each image was randomly displayed in one of the quadrants with an equal probability of appearing in each quadrant (). The location of the image ranged from 0.5° to 1.25° away from the axes of the cross dividing the screen. Each trial started with the presentation of an image for 1000 ms, which was followed by a 3000-ms period when only the cross remained on the screen. The assigned task was to indicate whether each image represented a natural or an artificial object by pressing one of two keys, and the participants were able to record their response during an interval of 3500 ms after the onset of the stimulus. Because the participants knew that their memory would be tested, they were instructed to concentrate on the encoding task.
Figure 1. Events during the encoding and retrieval phases of the source memory paradigm and during the visual and spatial working memory tasks. Additionally, an illustration of the response panel used in the source memory paradigm for the right-handed participants is shown
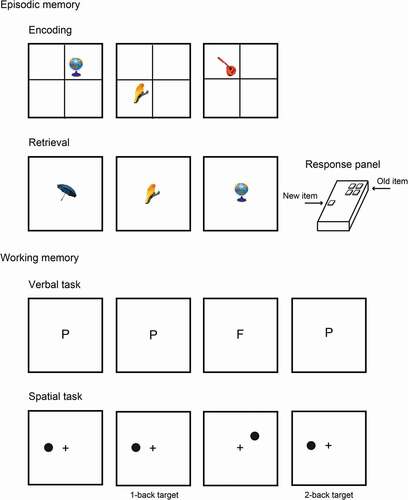
During the retrieval phase, the same events and timing were used as in the encoding phase; however, the cross dividing the screen in quadrants was not displayed and all images were presented in the center of the screen. The participants were asked to judge whether the image was new or old. If the image was old, they indicated the quadrant where the image was originally presented during the encoding phase by pressing one of the four keys that represented each quadrant of the screen. If the image was new, then the participants used their thumb to press the lower (fifth) key on the response panel. The participants were instructed to randomly select one of the four quadrants if they were confident that the image was old but were unable to remember its exact position.
2.3.2. Working memory paradigm
The verbal and spatial n-back tasks were assigned to each participant in counterbalanced order, and the two levels of difficulty (1-back and 2-back) within each domain were also presented in counterbalanced order. The participants performed brief versions of each task as a training procedure before performing each of the four n-back tasks. Each trial from both the verbal and spatial n-back tasks started with the presentation of the stimulus (letter or circle) for 300 ms, followed by a period of 2700 ms. After this time, the next stimulus was displayed. The participants were allowed to provide their response during the 3000-ms period following the onset of the stimulus. In the verbal tasks, the participants had to indicate whether the current letter was equal or not equal to the one presented in the last trial (1-back) or two trials before (2-back). In the spatial tasks, the participants were required to indicate whether the current circle was presented in the same position as the one displayed in the last trial (1-back) or two trials before (2-back). During each task, 72 trials were conducted and 33% of the trials were target trials, i.e., the letter or position of the current trial was equal to that presented one or two trials before according to the level of difficulty.
2.4. Data analysis
For the source memory task, only images that were correctly classified during the encoding task were included in the subsequent analysis. Recognition hits were defined as images presented in the encoding phase correctly classified as old in the retrieval phase. Source memory accuracy or recollection was estimated as the percent of recognition hits with a correct source response. False alarms were defined as incorrect responses for new images. Working memory in the verbal and spatial n-back tasks at each level of difficulty (1-back and 2-back) was estimated as discrimination levels by using d-prime (d′) values because they are not affected by the participants’ criteria for performing the tasks. All variables were examined with descriptive analyses, and variables had skewness within ± 2.2, and none of them had missing values. Differences in accuracy and speed in the source memory tasks, in discrimination and speed in the working memory tasks, and in participant characteristics between diabetic and nondiabetic groups were analyzed using independent-sample t-tests (two-tailed); Cohen’s d values are also reported for these analyses. For variables with unequal variance between groups, the degree of freedom was corrected with Satterthwaite’s procedure. The Holm–Bonferroni procedure was used to correct for multiple comparisons in the t-tests conducted on memory performance variables.
Hierarchical regression analyses were conducted to further determine the influence of diabetes on episodic and working memory performance. In the first step, we introduced the following demographic variables: age, sex years of education, vocabulary scores, MMSE scores and BDI scores. For the second step, we entered the following biological variables: body mass index (BMI), glucose, cholesterol, triglycerides, heart rate, systolic blood pressure (SBP) and diastolic blood pressure (DBP). Finally, for the third step, we entered the diabetes status. This procedure can determine the exact influence of diabetes on memory performance after controlling for possible confounders. The sample size was constraint by the number of participants diagnosed exclusively with diabetes (n = 100). Linear regression analyses were conducted to estimate whether the duration of diabetes, which was defined as the time since diagnosis, predicted memory performance. Age was included in these models to control for its influence on memory decay. All analyses were conducted by using Stata v. 16 (Texas, USA).
3. Results
Statistically significant differences were determined using the appropriate p-value cutoff according to the Holm–Bonferroni procedure. Images that were incorrectly classified during the encoding phase were excluded from the analyses (total exclusions = 5.6% and 4.8% for diabetic and nondiabetic participants, respectively). Source memory accuracy (t(198) = −6.72, p < .001, d = −0.95) and recognition hits (t(198) = −3.53, p < .001, d = −0.50) significantly differed between diabetic and nondiabetic individuals. Participants’ performance in the source memory task is depicted in . However, neither reaction times for correct source responses (t(198) = 0.06, p = .950, d = 0.01) nor recognition hits (t(198) = 0.18, p = .861, d = 0.02) differed between groups. The false alarm rates did not differ (t(198) = 1.45, p = .149, d = 0.20) between diabetic (mean ± standard deviation = 12.11 ± 9.76) and nondiabetic (10.03 ± 10.54) participants.
Figure 2. Mean and individual performance in the source memory paradigm for diabetic and nondiabetic groups. The lines indicate significant differences between groups based on t-tests. Error bars represent the 95% confidence intervals for the mean. *** p < .001
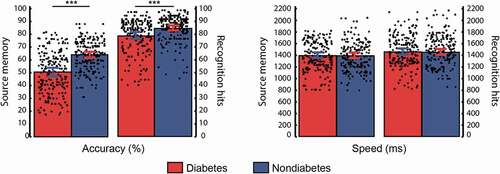
displays the participants’ performance in the working memory tasks. Discrimination levels were higher in nondiabetic individuals than in diabetic participants in the verbal 1-back task (t(198) = −3.53, p < .001, d = −0.50), verbal 2-back task (t(198) = −3.50, p < .001, d = −0.50), spatial 1-back task (t(198) = −2.43, p = .016, d = −0.34) and spatial 2-back task (t(198) = −3.14, p = .002, d = −0.44). Reaction times were faster in the nondiabetic participants than in the diabetic participants in the verbal 1-back task (t(198) = 2.91, p = .004, d = 0.41), verbal 2-back task (t(198) = 3.01, p = .003, d = 0.43) and spatial 1-back task (t(198) = 2.75, p = .007, d = 0.39) but not in the spatial 2-back task (t(198) = −0.10, p = .924, d = −0.01).
Figure 3. Mean and individual performance in the working memory tasks for diabetic and nondiabetic groups. The lines indicate significant differences between groups based on t-tests. Error bars represent the 95% confidence intervals for the mean. * p < .05, ** p < .01, *** p < .001
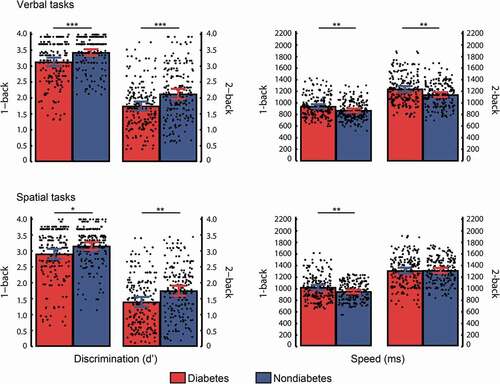
The results of the hierarchical regression analyses for variables predicting episodic memory in the final step are displayed in ; the results for variables predicting verbal working memory are shown in ; and the results for variables predicting spatial working memory are depicted in . Additional results from the first and second steps of these analyses are described in the Supplementary Material.
Table 2. Hierarchical regression analyses predicting episodic memory performance
Table 3. Hierarchical regression analyses predicting verbal working memory performance
Table 4. Hierarchical regression analyses predicting spatial working memory performance
Complete linear regression analysis results are displayed in Supplementary Material (Table S1). The time since the diagnosis of diabetes significantly predicted recognition (β = −.35, p < .001) after controlling for participant age (β = −.26, p = .009), discrimination levels in the verbal low difficulty working memory tasks (β = −.23, p = .035) after controlling for participant age (β = −.08, p = .444) and speed in the spatial 1-back task (β = .22, p = .047) after controlling for participant age (β = .10, p = .378).
4. Discussion
The present study reveals that diabetic participants showed less precise performance when they employed episodic memory and working memory compared with participants free from this disease. This lower performance was evident on both processes within episodic memory, recollection and recognition. Similarly, this performance reduction was observed in both verbal and spatial working memory domains and at both low and high levels of difficulty. Additionally, memory process speed in diabetic individuals was lower in the verbal 2-back task and spatial 1-back task than in nondiabetic individuals. These outcomes occur in individuals free from hypertension, hypotension, hypercholesterolemia and hypertriglyceridemia and after controlling for several demographic and biological variables, thus confirming the indubitable impact of diabetes disease on memory. Moreover, the time since participants were formally diagnosed as having diabetes significantly predicted the decline in recognition as well as the decay in discrimination levels in the verbal 1-back task and speed in the spatial 1-back task.
The tasks employed to measure episodic memory and working memory are distinguished for being highly reliable procedures to examine each of these two types of memory in detail and have been used worldwide (Diana et al., Citation2007; Owen et al., Citation2005). Therefore, the findings obtained in the present study can be directly compared with other studies (e.g., Zhang et al., Citation2016) and may be generalized to individuals sharing similar demographic and biological characteristics as those that participated in the present study.
As expected, recollection was lessened in diabetic individuals, and recognition was lessened as well, indicating the strong effect of diabetes on episodic memory. Compared with previous studies that have found minimal or no effects on memory (Asimakopoulou et al., Citation2002; Van Harten et al., Citation2007) after controlling for potential confounding variables, such as BMI, depression or hypertension, we observed memory deficits in diabetic participants after controlling for these same variables and several others. Episodic memory impairment in diabetic individuals may be explained by the finding that the hippocampus, the main brain region involved in recollection, suffers volume loss in diabetic patients and is sensitive to acute metabolic changes (McCrimmon et al., Citation2012). Source memory shows a progressive decline with advancing age in healthy individuals (Cansino et al., Citation2013), whereas in diabetic participants, this memory decay seems to occur more rapidly, which is consistent with the proposal that the diabetic brain is more susceptible to the aging process (Ryan & Geckle, Citation2000).
The lower working memory performance observed in diabetic participants compared to nondiabetic individuals in both modality domains and complexity tasks indicates that diabetes has a broad effect on this type of memory. This outcome is consistent with previous studies that have found lower global working memory performance in diabetic participants than in control participants, with moderate effect sizes (Monette et al., Citation2014). However, the present study provides further evidence that diabetes leads to verbal and visual working memory deficits independent of the demands of the task. The fact that diabetic participants only showed reductions in the speed memory processes in the verbal 2-back and spatial 1-back tasks should be attributed to task difficulty. These two tasks have moderate difficulty, thus allowing for the differentiation of speed differences between groups; however, the spatial 2-back task is highly demanding, thus leading to large speed variability that masks possible differences. Although speed in the verbal 1-back task was significantly different between groups, hierarchical regressions showed that this difference was explained by the BDI score rather than diabetes.
The diabetic group was characterized by higher BDI scores than the nondiabetic group, and these scores were significant predictors of lower recognition and verbal working memory performance. Depression and diabetes are highly related, as revealed by a meta-analysis (Anderson et al., Citation2001). However, because the causal relationship between these two conditions remains unclear, it is likely that their relationship is bidirectional (Lee et al., Citation2016). Depression is also a major risk factor for memory deficits, as observed in a 12-year longitudinal study that concluded that depression symptoms precede memory decline (Zahodne et al., Citation2014). Therefore, the effects of diabetes on memory are intensified due to its comorbidity with depression.
Triglyceride nonfasting levels were higher in diabetic participants than in nondiabetic participants, but only recognition was slightly influenced by this variable. High triglyceride levels are expected in diabetes due to excessive energy intake, insulin resistance, uncontrolled glucose, elevated levels of free fatty acids and low-grade inflammation (Parhofer, Citation2015). Heart rate and SBP were higher in diabetic participants than in individuals without diabetes, as has been observed previously (Smulyan et al., Citation2016). Neither heart rate nor SBP influenced memory performance in the present study, which was probably because these measurements were still within the normal range for this type of patient (Lipman & Schiffrin, Citation2012).
The finding that diabetic participants’ nonfasting glucose levels were higher than those for nondiabetic participants indicates that even under pharmacological treatment, diabetic individuals do not reach the most preferable glucose level. Thus, the strong effect of diabetes on memory may also be attributed to poor glucose control leading to hyperglycemia and in turn to several concurrent events associated with cognitive impairment, such ischemic damage, brain insulin deficiency and vascular dysfunction (Kim, Citation2019).
One limitation of the study is that the time in which the participants were affected by diabetes because this information might be imprecise due to memory mistakes. Another limitation is the use of a cross-sectional design that does not allow real-time follow-up of the course of the diabetic disease and its gradual effects on memory. Although we controlled for the influence of several potential confounders to reliably examine the effects of diabetes on memory, the list of control variables is not exhaustive. These limitations should be considered when interpreting the results of the present study.
5. Conclusion
The main implication of our study is that diabetes has a broad effect on episodic memory and working memory because both the most and least demanding processes within each of these types of memory were diminished in diabetic participants, and some of these memory deficits intensified with the time since diagnosis. This wide effect of diabetes on memory suggests that diabetic individuals experience advancing brain aging, which is further supported by the fact that episodic memory and working memory are the first to decline with advancing age and are the most essential memory functions to maintain efficient control of everyday activities and preserve autonomy.
Supplemental Material
Download PDF (103.8 KB)Supplementary material
Supplemental data for this article can be accessed here.
Disclosure statement
No potential conflict of interest was reported by the author(s).
Data availability statement
The data that support the findings of this study are openly available in the repository Open Science Framework at http://doi.org/10.17605/OSF.IO/QHK5M.
Additional information
Funding
Notes on contributors
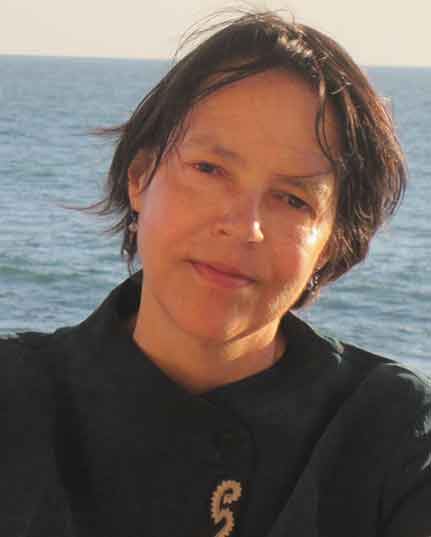
Selene Cansino
Selene Cansino is a professor and head of the Laboratory of NeuroCognition in the Faculty of Psychology at the National Autonomous University of Mexico (UNAM, for its acronym in Spanish). Cansino’s research focuses on the behavioral, anatomical and physiological bases of cognitive processes, a subject that she studies through the techniques of event-related potentials, magnetoencephalography, and functional magnetic resonance imaging. In particular, she is interested in the neurofunctional interactions of working memory and episodic memory with other processes, such as perception, attention, cognitive control, emotion, learning, plasticity, and aging. She earned her PhD at UNAM and completed postdoctoral training at the University of Paris VI and New York University.
References
- Anderson, R. J., Freedland, K. E., Clouse, R. E., & Lustman, P. J. (2001). The prevalence of comorbid depression in adults with diabetes: A meta-analysis. Diabetes Care, 24(6), 1069–15. https://doi.org/10.2337/diacare.24.6.1069
- Asimakopoulou, K. G., Hampson, S. E., & Morrish, N. J. (2002). Neuropsychological functioning in older people with type 2 diabetes: The effect of controlling for confounding factors. Diabetic Medicine, 19(4), 311–316. https://doi.org/10.1046/j.1464-5491.2002.00680.x
- Awad, N., Gagnon, M., & Messier, C. (2004). The relationship between impaired glucose tolerance, type 2 diabetes, and cognitive function. Journal of Clinical and Experimental Neuropsychology, 26(8), 1044–1080. https://doi.org/10.1080/13803390490514875
- Beck, A. T. (1987). Beck depression inventory. The Psychological Corporation.
- Biessels, G. J., Deary, I. J., & Ryan, C. M. (2008). Cognition and diabetes: A lifespan perspective. The Lancet Neurology, 7(2), 184–190. https://doi.org/10.1016/S1474-4422(08)70021-8
- Biessels, G. J., & Despa, F. (2018). Cognitive decline and dementia in diabetes mellitus: Mechanisms and clinical implications. Nature Reviews Endocrinology, 14(10), 591–604. https://doi.org/10.1038/s41574-018-0048-7
- Budson, A. E., & Price, B. H. (2005). Memory dysfunction. The New England Journal of Medicine, 352(7), 692–699. https://doi.org/10.1056/NEJMra041071
- Cansino, S., Estrada-Manilla, C., Hernández-Ramos, E., Martínez-Galindo, J. G., Torres-Trejo, F., Gómez-Fernández, T., Ayala-Hernández, M., Osorio, D., Cedillo-Tinoco, M., Garcés-Flores, L., Gómez-Melgarejo, S., Beltrán-Palacios, K., Guadalupe García-Lázaro, H., García-Gutiérrez, F., Cadena-Arenas, Y., Fernández-Apan, L., Bärtschi, A., Resendiz-Vera, J., & Rodríguez-Ortiz, M. D. (2013). The rate of source memory decline across the adult life span. Developmental Psychology, 49(5), 973–985. https://doi.org/10.1037/a0028894
- Cansino, S., Maquet, P., Dolan, R. J., & Rugg, M. D. (2002). Brain activity underlying encoding and retrieval of source memory. Cerebral Cortex, 12(10), 1048–1056. http://doi.org/10.1093/cercor/12.10.1048
- Daselaar, S. M., Dennis, N. A., & Cabeza, R. (2007). Ageing: Age-related changes in episodic and working memory. In S. A. R. B. Rombouts, F. Barkhof, & P. Scheltens (Eds.), Clinical applications of functional brain MRI (pp. 115–148). Oxford University Press.
- Diana, R. A., Yonelinas, A. P., & Ranganath, C. (2007). Imaging recollection and familiarity in the medial temporal lobe: A three-component model. Trends in Cognitive Sciences, 11(9), 379–386. https://doi.org/10.1016/j.tics.2007.08.001
- Folstein, M. F., Folstein, S. E., & McHugh, P. R. (1975). Mini-mental state: A practical method for grading the cognitive state of patients for the clinician. Journal of Psychiatric Research, 12(3), 189–198. https://doi.org/10.1016/0022-3956(75)90026-6
- Hugenschmidt, C. E., Hsu, F. C., Hayasaka, S., Carr, J. J., Freedman, B. I., Nyenhuis, D. L., Williamson, J. D., & Bowden, D. W. (2013). The influence of subclinical cardiovascular disease and related risk factors on cognition in type 2 diabetes mellitus: The DHS-Mind study. Journal of Diabetes and Its Complications, 27(5), 422–428. https://doi.org/10.1016/j.jdiacomp.2013.04.004
- Kim, H. G. (2019). Cognitive dysfunctions in individuals with diabetes mellitus. Yeungnam University Journal of Medicine, 36(3), 183–191. https://doi.org/10.12701/yujm.2019.00255
- Kirchner, W. K. (1958). Age differences in short-term retention of rapidly changing information. Journal of Experimental Psychology, 55(4), 352–358. https://doi.org/10.1037/h0043688
- Lee, S. H., Zabolotny, J. M., Huang, H., Lee, H., & Kim, Y. B. (2016). Insulin in the nervous system and the mind: Functions in metabolism, memory, and mood. Molecular Metabolism, 5(8), 589–601. https://doi.org/10.1016/j.molmet.2016.06.011
- Lezak, M. D. (1995). Neuropsychological assessment (3rd ed.). Oxford University Press.
- Lipman, M. L., & Schiffrin, E. L. (2012). What is the ideal blood pressure goal for patients with diabetes mellitus and nephropathy? Current Cardiology Reports, 14(6), 651–659. https://doi.org/10.1007/s11886-012-0308-4
- Luo, L., & Craik, F. I. (2008). Aging and memory: A cognitive approach. Canadian Journal of Psychiatry, 53(6), 346–353. https://doi.org/10.1177/070674370805300603
- Mandler, G. (1980). Recognizing: The judgment of previous occurrence. Psychological Review, 87(3), 252–271. https://doi.org/10.1037/0033-295X.87.3.252
- McCrimmon, R. J., Ryan, C. M., & Frier, B. M. (2012). Diabetes and cognitive dysfunction. Lancet, 379(9833), 2291–2299. https://doi.org/10.1016/S0140-6736(12)60360-2
- Milles, W. R., & Root, H. F. (1922). Psychologic tests applied to diabetic patients. Archives of Internal Medicine, 30(6), 767–777. https://doi.org/10.1001/archinte.1922.00110120086003
- Monette, M. C. E., Baird, A., & Jackson, D., . L. (2014). Meta-analysis of cognitive functioning in nondemented adults with type 2 diabetes mellitus. Canadian Journal of Diabetes, 38(6), 401–408. https://doi.org/10.1016/j.jcjd.2014.01.014
- Owen, A. M., McMillan, K. M., Laird, A. R., & Bullmore, E. (2005). N-back working memory paradigm: A meta-analysis of normative functional neuroimaging studies. Human Brain Mapping, 25(1), 46–59. https://doi.org/10.1002/hbm.20131
- Palta, P., Schneider, A. L., Biessels, G. J., Touradji, P., & Hill-Briggs, F. (2014). Magnitude of cognitive dysfunction in adults with type 2 diabetes: A meta-analysis of six cognitive domains and the most frequently reported neuropsychological tests within domains. Journal of the International Neuropsychological Society, 20(3), 278–291. https://doi.org/10.1017/S1355617713001483
- Pappas, C., Andel, R., Infurna, F. J., & Seetharaman, S. (2017). Glycated haemoglobin (HbA1c), diabetes and trajectories of change in episodic memory performance. Journal of Epidemiology and Community Health, 71(2), 115–120. https://doi.org/10.1136/jech-2016-207588
- Parhofer, K. G. (2015). Interaction between glucose and lipid metabolism: More than diabetic dyslipidemia. Diabetes & Metabolism Journal, 39(5), 353–362. https://doi.org/10.4093/dmj.2015.39.5.353
- Ryan, C. M., & Geckle, M. (2000). Why is learning and memory dysfunction in type 2 diabetes limited to older adults? Diabetes/Metabolism Research and Reviews, 16(5), 308–315. https://doi.org/10.1002/1520-7560(2000)9999:9999<::aid-dmrr141><::aid-dmrr141>3.0.co;2-x
- Smulyan, H., Lieber, A., & Safar, M. E. (2016). Hypertension, diabetes type II, and their association: Role of arterial stiffness. American Journal of Hypertension, 29(1), 5–13. https://doi.org/10.1093/ajh/hpv107
- Tulving, E. (1985). Memory and consciousness. Canadian Psychology, 26(1), 1–12. https://doi.org/10.1037/h0080017
- Tulving, E. (2002). Chronesthesia: Conscious awareness of subjective time. In D. T. Stuss & R. T. Knight (Eds.), Principles of frontal lobe function (pp. 311–325). Oxford University Press. https://doi.org/10.1093/acprof:oso/9780195134971.003.0020
- van Harten, B., Oosterman, J., Muslimovic, D., Van Loon, B. J., Scheltens, P., & Weinstein, H. C. (2007). Cognitive impairment and MRI correlates in the elderly patients with type 2 diabetes mellitus. Age Ageing, 36(2), 164–170. https://doi.org/10.1093/ageing/afl180
- Wechsler, D. (1981). WAIS-R manual. The Psychological Corporation.
- Yonelinas, A. P., Aly, M., Wang, W. C., & Koen, J. D. (2010). Recollection and familiarity: Examining controversial assumptions and new directions. Hippocampus, 20(11), 1178–1194. https://doi.org/10.1002/hipo.20864
- Zahodne, L. B., Stern, Y., & Manly, J. J. (2014). Depressive symptoms precede memory decline, but not vice versa, in non-demented older adults. Journal of the American Geriatrics Society, 62(1), 130–134. https://doi.org/10.1111/jgs.12600
- Zhang, Y., Lu, S., Liu, C., Zhang, H., Zhou, X., Ni, C., Zhang, Y., Zhang, H., Qin, W., & Zhang, Q. (2016). Altered brain activation and functional connectivity in working memory related networks in patients with type 2 diabetes: An ICA-based analysis. Scientific Reports, 6(1), 23767. https://doi.org/10.1038/srep23767