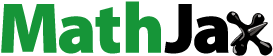
Abstract
Plastic surgeons report neck, shoulder and back pain when wearing head-mounted magnifiers (loupes) during operations. There will be many factors contributing to such pain. In order to explore these factors this paper developed a novel application of Multi-objective Optimization (MOO) which used postural constraints on anthropometric models to determine Rapid Upper Limb Analysis (RULA) scores. For the pain experienced by surgeons wearing loupes, the analyses showed that adjusting the height of table and suitable working distance of loupes for surgeon could decrease the flexion angle of neck. The results demonstrated that it is possible to predict RULA scores for the range of postures and propose that this approach could be used to quantify risk assessment, particularly in the selection and fitting of loupes and in the specification of working height for surgeons.
Public Interest Statement
Plastic surgeons report neck, shoulder and back pain when wearing head-mounted magnifiers (loupes) during operations. There will be many factors contributing to such pain. In order to explore these factors this paper developed a novel application of Multi-objective Optimization which used postural constraints on anthropometric models to determine Rapid Upper Limb Analysis scores. For surgeons wearing loupes, the analyses showed that adjusting the height of table and suitable working distance of loupes for surgeon could decrease the flexion angle of neck. The approach is applied to the surgeons who wear head-mounted magnifiers and report a neck injury.
1. Introduction
Plastic surgery involves a range of specialisms directed at the reconstruction or correction of dysfunctional or defective parts of the body. Given the nature of the work (particularly when working on children or on the hand), it is common for plastic surgeons to employ some form of visual aids, such as microscopes or head-mounted magnifying glasses called loupes. While these visual aids can enhance surgeons’ vision, there can be a need to adopt uncomfortable postures during an operation in order to see the operating site while performing action on that site. Not surprisingly, therefore, plastic surgeons who wear loupes during surgery report a high incidence of musculoskeletal injuries (Capone, Parikh, Gatti, Davidson, & Davison, Citation2010; Nimbarte, Zreiqat, & Chapman, Citation2012). A survey of European surgeons reported more than 80% (n = 284) had discomfort in the neck, shoulder and back muscles associated with operating (Wauben, van Veelen, Gossot, & Goossens, Citation2006). Sivak-Callcott reported 58% of ophthalmic plastic surgeons (n = 139) had neck pain associated with operating. Nearly 10% had to cease operating as a result of neck pain (Sivak-Callcott et al., Citation2011). In a recent survey, authors identified contributory factors as the age of the respondent (older respondents were more likely to report pain), the number of hours operating while wearing loupes (more than 15 h per week performing operations led to higher incidence of pain), and the magnification of the loupes (higher magnification of loupes resulted in more reports of symptoms). While the first two factors could be seen as self-explanatory, it is worth considering the third and why this relates the design of the loupes.
Surgical loupes consist of magnifying lenses mounted on prescription spectacles. Loupes are custom-fitted for an individual surgeon based on two factors: the working distance and the declination angle (Chang, Citation2014). The working distance is influenced by the magnification of the loupes; ranging from around 2x to 5x. For some procedures, there is a recommendation to use 2.5x (1, 2). In our survey, 23% of respondents used 2.5x while 67% used 3.5x (the remainder using 2x, 3x or 4x). The magnification will influence the size of the visual field that can be seen clearly at a given viewing distance, e.g. higher magnification loupes of 4x or 5x will have a smaller visual field in sharp magnification (and might be used for vascular surgery for instance). Loupes have specified viewing distances (ranging from 34 to 50 cm) which are intended to be distances at which images are clear. However, viewing distance will also be influenced by the stature of the surgeon and the working height of the operation. Consequently, wearing loupes creates the need to trade-off the loupe’s viewing distance (for a clear image) and the surgeon’s working distance (to gain access to the patient). Because of the limitation of viewing direction and working distance, the flexion angle of the neck could increase as the surgeon adjusts their posture during an operation.
A our preliminary study, using Vicon Motion Capture compared postures for four experienced surgeons performing simple tasks with and without loupes, while sitting or standing, and at different operating heights, as shown in Figure . From the analysis of surgeon posture, it was found that angles of neck and head were bigger as surgeon with loupes than without loupes on different height table. This indicated how differences in head and neck angle result from wearing loupes, and that these differences vary with the height of the table on which the task was performed.
Figure 1. The impact on the surgeon’s posture of operating with loupes magnification: Lateral views recorded by Vicon Motion Capture whilst performing a manual tasks: (a) The head is held in the “Head forward position” while performing a simple task with loupes. The neck is in anterior flexion. The head is in a protracted position anterior to that of neutral posture: (b) Performing the same tasks without loupes decreases neck flexion and the “Head forward posture”.
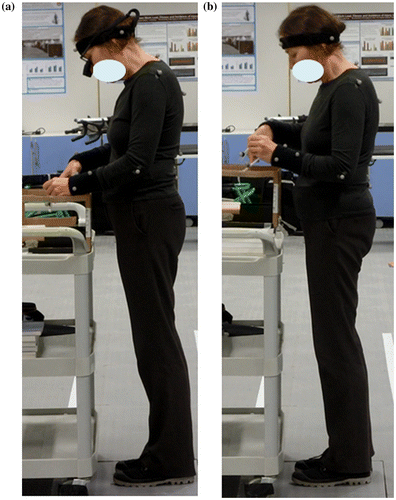
In this paper, the relationship between surgeon stature (SS), working distance of loupes (WD, the distance from surgeon’s eye to the patient’s operation position) and table height (TH, the vertical distance from the patient’s operation position to the floor) will be analyzed. The definition of working distance varies across specialisms and types of operation; some surgeons (particularly those operating on hands or feet) might prefer to remain seated during an operation, whereas surgeons performing other types of operation might prefer to stand. From initial discussion with surgeons it is noted that loupes are often fitted when the surgeon is seated and the viewing distance based on this. This suggests potential problems when moving from sitting to standing. In this paper, author focus on standing surgeons.
2. Methods
In this paper, author employ MOO method combining digital anthropometric modelling, and RULA. Figure illustrates the approach taken in this paper.
Figure 2. Data processing flow chart.
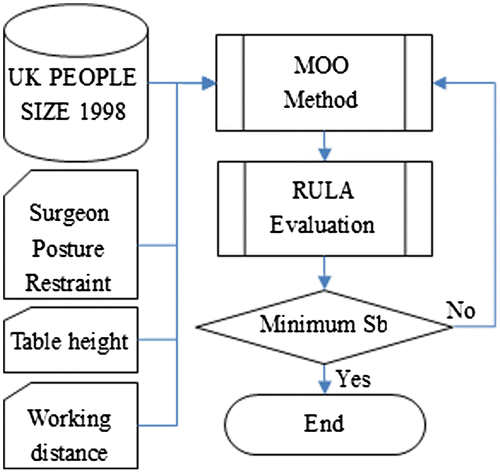
2.1. Digital anthropometric models
A digital anthropometric model is used in this approach to define the working posture in terms of the parameters which can be optimized. In order to simplify the model, a 2-dimensional body-link model is built based on the joints and links, as shown in Figure .
Figure 3. Operation position; I: Instrument; E: Eye line: The line connects the head joint and eye; WD: Working distance of loupes; TH: Table height; W: x-coordinate of operation field: (a) 2-D structure and link constraint. (b) Neck and Head link diagram.
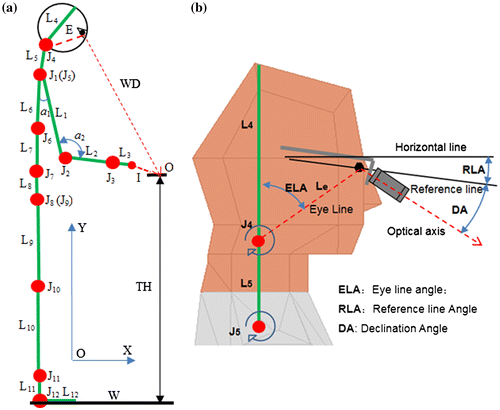
In Figure (a), each joint has a local coordinate (Y axis perpendicular to the floor, X axis in line with the floor). The height of table (TH) is set midway between the umbilicus and sternum. In a study of experienced surgeons performing discectomy (on a spine surgery simulator) when wearing loupes, it is proposed that this table height is optimal for reducing surgeon musculoskeletal fatigue (Park et al., Citation2012). The working distance (WD) is defined by the dotted line linking the operating position (O) being worked on using instrument (I) and the eye (E).
Figure (b) shows the model of the head wearing loupes. This has a reference line connecting the top of the ears to the corner of the eyes. The Reference Line Angle (RLA) is the deviation from a horizontal line from the top of the ears and is taken as 12° (Chang, Citation2014). The declination angle for the loupes is the angle between the reference line and the optical axis of loupes. Through-the-lens (TTL) loupes have declination angles of between 20 and 25°; a Declination Angle (DA) of 25° is assumed. The Eye Line Angle (ELA), defined with reference to a line connecting the head joint (J4) and the eye, is defined as the angle between the eye line and the head link (L4) and is set at 45°.
Joint angle limits are defined by the working posture of surgeons (Chang, Citation2014; Damodaran, Lee, & Lee, Citation2013; Park et al., Citation2014; Steinhilber et al., Citation2015), and determined using the SAMMIE system (Freer, Marshall, & Summerskill, Citation2008). In order to reduce model complexity, author assume that some joints do not contribute significantly to postural variability during the course of an operation, and so these can be frozen in the model. The frozen joints are the hip joint (J8), thigh joint (J9), leg joint (J10), ankle joint (J11) and foot joint (J12). This assumes that postural change happens from the waist upwards, and this paper are particularly interested in the extension and flexion of the neck during an operation. Thus, 7 active joints (J1–J7) are used for the standing model. ai represents the joint angle between Li-1 and Li, as shown in Figure . Joint angle limits for each degree of freedom are listed in Table .
Table 1. Joint angle limits (degree)
In this study it is assumed that the surgeon will also work with surgical instruments, which provide a focal point for their vision with reference their hand position. Table shows the corresponding dimensions of scissors (Item: MB150R, Length: 140 mm) (Aesculap, Inc, Citation2008). It is assumed that half of length of instrument is in the hand, so the valid length of instruments is lt = 70 mm. It is defined that the angle between Y axis and instrument is at = 120∘.
Table 2. Other length and angle variants
In order to accommodate a wide range of the surgeons’ stature, ten digital human models are defined in terms of standing height of adults from 5% female up to 95% male (stature) based on UK PEOPLE SIZE 1998 (Freer et al., Citation2008). The stature range is from 1,534 to 1,864 mm. Table lists the body data for female and male models at 20 or 25 percentile intervals: 5, 25, 50, 75 and 95%.
Table 3. Stature and link length of digital human (mm)
In Table , F25 means the 25% female, and M25 means the 25% male, and so on. One column in Table represents the stature and length of links. For example, in the 3th column, stature = 1,596 and L1 = 274 mean that the stature of F25 is 1,596 mm and length of upper arm is 274 mm (Figure (a) shows the position of L1).
2.2. The RULA method
RULA is a popular tool to evaluate risk of musculoskeletal injury is called (McAtamney & Coreltt, Citation1993). RULA supports classification of posture in terms of potential musculoskeletal risk through a simple pencil and paper pro-forma. This can be completed from observation (either directly in the field or from video recordings) and can provide a consistent and reliable identification of postures which could be harmful. For this paper, authors are interested whether it is possible to use RULA predictively, i.e. as a means of identifying postural problems from models rather than observation. In this respect, this paper follow the lead of Plantard who captured human posture using the Microsoft KINECT sensor and derived corresponding RULA scores for a large set of poses and sensor placements (Plantard, Auvinet, Pierres, & Multon, Citation2015). Authors are interested in relating the RULA classification scheme to the postures defined by an anthropometric model. A core problem that needs to be solved prior to implementing such an approach is the need to determine which parameters are most significant in contributing to musculoskeletal risk classified by RULA. As there are multiple parameters which can contribute to risk, this requires the solution of a multi-objective problem.
In the RULA method, there are three score tables. Table B describes risks associated with neck and trunk angle. Since neck discomfort is the most common disorder reported by plastic surgeons, this paper employ Table B (McAtamney & Coreltt, Citation1993). The score of Table B is defined as Sb. In order to analyse the continuously changing angles of neck and trunk, two functions—Equations (1) and (2)—were created by using quadratic fit of the RULA score and specific joint angles, according to studies by McAtamney and Coreltt (Citation1993). In order to obtain a more precise definition of Sb, Equation (3) was created by using liner fit based on Table B.(1)
(1)
(2)
(2)
(3)
(3)
In Equation (1), Sn is the score for neck angle, and ane is the angle of neck. In Equation (2), St is the score for trunk angle, and atr is the angle of trunk. For consistency with SAMMIE, author defined these joint angles as: ane = a4 + a5; atr = a6 + a7.
2.3. Multi-objective optimization
The process of systematically and simultaneously optimizing a set of objective functions is called Multi-Objective Optimization (Marler & Arora, Citation2004). In general, a multi-objective optimization problem can be posed as follows:
(a) Minimize Function F of (x):
where k is the number of objective functions.
(b) Subject to constraints defined as:
where m is the number of inequality constraints, and e is the number of equality constraints.
x∈En is a vector of design variables, where n is the number of independent variables xi. F(x)∈Ek is a vector of objective (or cost) functions Fi (x): En→E1. xi∗ is the point that minimizes the objective function Fi (x). The feasible design space X (often called the constraint set) is defined as the set {x|gj (x) ≤ 0,j = 1, 2, ...,m; and hi(x)= 0,i = 1, 2, ..., e} (Marler & Arora, Citation2004).
Multi-Objective Optimization has been used to predict: joint displacement; musculoskeletal discomfort; potential energy (Gagg, Yang, & Howard, Citation2012; Marler, Arora, Yang, Kim, & Abdel-Malek, Citation2009). An advantage of this approach is that one just needs to add additional constraints for different scenarios (Yang, Marler, & Rahmatalla, Citation2010).
In this paper, Sb is used as the objective value. Joint angles ai are taken as predictor variables. By using Multi-Objective Optimization, minimal Sb (where risk of neck and trunk is the lowest) can be calculated out when ai is optimal. Based on the standing posture of surgeons, joint angle restraints will be implemented. In the following definition, the reader can refer to Figure (a) for the specific links (l) and specific angles (a) used in the calculation. In this model, the end of instruments must touch the operation position on the operation table. It is assumed that avatar in the digital anthropometric model should see the instruments (I) at the operating position (O) at a working distance (WD) and declination angle (DA) (see Figure (b)). lh is equal to table height (TH), and le is the length of eye line in Figure . The values of a indicate the angles of the various joints in the model (see Table ). The optimization problem is defined as follows:(4)
(4)
(5)
(5)
Subject to:(6)
(6)
(7)
(7)
(8)
(8)
(9)
(9)
(10)
(10)
(11)
(11)
Equations (6–11) define constraints for the model as follows. Equation (6) defines the vision constraint allowing standing surgeon to see clearly the instrument based on the assigned WD and TH. h1 must be equal to zero when minimal Sb is gained. Equation (7) defines the vertical position of instrument. Equation (8) defines the horizontal position of instrument. h2 and h3 must be equal to zero when minimal Sb is gained. Equations (7) and (8) ensure the surgeon to touch the operation field with the instrument meanwhile seeing the instrument. Equation (9) defines the wrist joint keeping no bent. is zero as minimal Sb is gained. Equation (10) defines the upper arm swinging forward. g1 is less than zero as minimal Sb is gained. Equation (11) defines the inequality constraint of joints angle based on Table .
Based on the surgeon’s standing posture, ai will be limited by the upper and lower values that satisfy all of the constraints in Equations (6–11). Thus, the aim is to define the working posture to be modelled, in terms of the key constraints that affect the posture when performing a given type of task using a given set of equipment, and then to calculate the potential risk score (defined by RULA Table B) that relates to a person of a given stature adopting this working posture.
3. Results
In order to demonstrate the application of the approach followed in this paper, ten avatars are considered with standing height from 5% female up to 95% male digital human models. The interrelations among Sb, SS, WD and TH are then analysed based on ten digital human models.
The surgeon stature (SS) range is from 1,534 to 1,864 mm. Table height (TH) is from 800 to 1,250 mm (increment is 50 mm). When SS and TH are changed according to the mentioned values as above, minimal Sb can be calculated out by using Multi-Objective Optimization. The results are shown in Table . Every minimal Sb is got based on the optimal AN and WD. The corresponding AN and WD are separately shown in Tables and .
Table 4. Sb scores as wearing 25 degree loupes
Table 5. AN(degree) as wearing 25 degree loupes (degree)
Table 6. WD(mm) as wearing 25 degree loupes (mm)
3.1 Predicting risk of discomfort (calculating Sb)
Table shows the interactions between Sb, SS and TH. The top zone, with the “–” symbol indicates no solution. For the green zone, 2 ≤ Sb < 3. For the yellow zone, 3 ≤ Sb < 4. For the red zone, Sb ≥ 4. From Table , author make the following observations:
(1) | When TH ∊ {1,200, 1,250}, Surgeon Stature ≤ 95% female and < 50% male do not have solutions. | ||||
(2) | ≤ 5% female does not have a solution when TH = 1,150 mm. | ||||
(3) | When | ||||
(4) | When TH | ||||
(5) | When TH |
3.2. Calculating neck angle (AN)
Table shows the interactions between AN, SS and TH. AN is a response to Sb in Table . The top zone, white and with the “–” symbol indicates no solution. The green zone, AN is 12 ≤ AN < 24. In the yellow zone, AN is 24 ≤ AN < 35. In the red zone, AN is AN ≥ 35. Assuming that cervical symptoms are more common as neck flexion in excess of 15° (Capone et al., Citation2010), and maximum head tilt should be less than 20° (Valachi, Citation2008), author define 15° as the criteria for risky posture and 20° as critical. In Table , risky values of AN are shown in bold and critical values in bold italic. Table shows how TH creates problems for most of the values of SS, and even the range of 1,000 to 1,150 poses risk in terms of neck angle.
3.2. Calculating working distance (WD)
Table shows the interactions between WD, SS and TH. WD is response to Sb in Table .The top zone, white and with the “–” symbol indicates no solution. The green zone, WD is 380 ≤ WD < 700. In the yellow zone, WD is 560 ≤ AN < 760. In the red zone, WD is 620 ≤ WD < 760. Recall that the recommended working distance when wearing loupes is 34–50 cm. From this, it is assumed that values above 500 mm could be problematic because there will be a compromise between the viewing distance at which loupes focus clearly and the working distance of the surgeon. In Table , these values are shown in bold and imply that majority of the surgeons will need to adjust their posture in order to work at these table heights.
4. Discussion
Tables – show how it is possible to decrease the angle of neck by adjusting the table height, or by changing the working distance. For the same SS, WD has an inverse correlation with TH. If TH becomes too low, WD will increase leading to an increase in AN. For example, consider M50 working at different table heights. For all values of TH, the working distanced (WD) exceeds the proposed value of 500 mm and, from 1,200, the neck angle is greater than 15° and the RULA risk increases beyond 3 with TH < 1,000 mm.
From the analysis of ten digital human models, TH = 1,100 mm appears suitable for most surgeons. Using this assumption, and the results shown in Tables –, a virtual scene is built in SAMMIE, as shown Figure . Figure shows surgeons of different stature can have an optimal posture when they work together. In order to achieve comfortable neck angle, they must wear loupes with matching viewing distance, stand at the correct distance from the table and maintain appropriate joint angles of arm to ensure that the instrument can touch the object. While this is the ideal (based on our analysis), this also highlights the potential for risk to vary as the operation progresses. For example, it is often necessary to change posture, either to affect a task, or to improve vision, or to collect or pass over an instrument. Consequently, while the ideal model illustrates the desirable, static postures, these are likely to change with the operation. The point that author would make here is that there are regions (as shown in Tables –) in which the predicted risk of neck injury (defined by RULA scores) will increase for surgeons of specific stature working at WD defined by the viewing distance of their loupes.
Figure 4. Virtual scene of surgeon doing operation as TH = 1,100 mm.
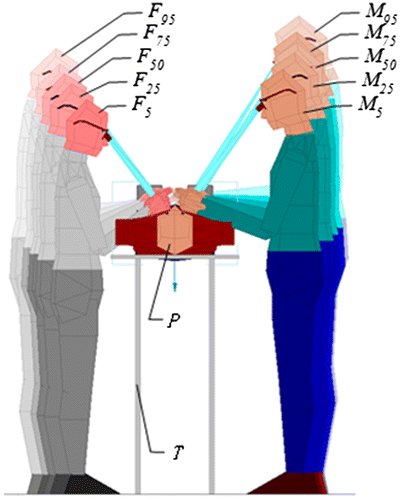
Given the number of reports of musculoskeletal injury (particularly to the neck), it is possible that current practice does not consider the range of the postures that will be encountered. It is proposed that, rather than complicating the fitting procedure, it could be beneficial to use the process outlined in this paper (or a modified and simplified version) to conduct additional checks on the use of the loupes and to provide advice and guidance on appropriate settings for TH and WD, given loupes of a particular prescription and specification and surgeon of a particular stature.
5. Conclusions
The study shows how RULA scores change for different table heights and working distance during an operation. Of particular interest to our work is that fact that head-mounted magnifiers (loupes) constrain the working distance by their viewing distance. It is shown how WD interacts with the stature of surgeon. RULA is a useful assessment value for posture prediction. The result shows that reasonable table height and working distance of loupes for surgeon could decrease the flexion angle of neck. Certainly when loupes are fitted to a surgeon (and all loupes are custom-modified and fitted as bespoke equipment for surgeons), there is the need to consider how the viewing distance and angle corresponds to the likely activity of the surgeon. Anecdotal evidence suggests that fitting typically takes place when the surgeon is seated, without performing surgical (or simulated, at least, surgical) tasks and in an environment which differs from the operating theatre. Consequently, the fitting will rely on the experience of the surgeon (in judging likely values of WD) and the expertise of the loupe-fitter (in terms of calculating viewing angle).
Practitioner summary
This paper demonstrates how risk of musculoskeletal discomfort (defined using RULA scores) can be predicted using virtual human models. The approach is applied to the surgeons who wear head-mounted magnifiers and report a neck injury.
Funding
This work was supported by the China Scholarship Council (CSC). CSC provided Zhelin Li with expenses including travel, living and health-care for his academic visiting in the University of Birmingham.
Cover image
Source: Author
Additional information
Notes on contributors
Zhelin Li
Dr Li Zhelin is a professor in the Design School of South China University of Technology. He is the director of the Laboratory Center at SCUT School of Design. His research fields are ergonomics evaluation of products, digital human modeling, new interface technology and interactive products design. His research projects include ergonomics analysis of head-mounted product, human-machine interface techniques, development of control software in recent years. He was a visiting research fellow in the School of Engineering, Birmingham University UK in 2015. The research topic is about plastic surgeons during surgery and define possible postures that may contribute to cervical musculoskeletal disorders by using ergonomic methods (motion capture techniques) when he was in UK.
References
- Aesculap, Inc. (2008). Surgical instrument catalog supplement. Retrieved July 10, 2015, from http://www.surgical-instruments-usa.info/images/content/en/doc766-surgical_inst_supplement.pdf
- Capone, A. C., Parikh, P. M., Gatti, M. E., Davidson, B. J., & Davison, S. P. (2010). Occupational injury in plastic surgeons. Plastic and Reconstructive Surgery, 125(5), 1555–1561.
- Chang, B. (2014). Key factors for ordering customer loupes, part 1: Declination angle as the key ergonomic factor. ErgoPractice News. Retrieved from http://archive.constantcontact.com/fs113/1113715421610/archive/1117208896207.html
- Damodaran, O., Lee, J., & Lee, G. (2013). Microscope in modern spinal surgery: Advantages, ergonomics and limitations. ANZ Journal of Surgery, 83(4), 211–214.
- Freer, M., Marshall, R., & Summerskill, S. (2008). Manual for SAMMIE System v8.3. SAMMIE CAD Ltd.
- Gagg, J., Yang, J., & Howard, B. (2012). Hybrid method for driver accommodation using optimization-based digital human models. Computer-Aided Design, 44, 29–39.
- Marler, R. T., & Arora, J. S. (2004). Survey of multi-objective optimization methods for engineering. Structural and Multidisciplinary Optimization, 26(6), 369–395.
- Marler, R. T., Arora, J. S., Yang, J., Kim, H.-J., & Abdel-Malek, K. (2009). Use of multi-objective optimization for digital human posture prediction. Engineering Optimization, 41(10), 925–943.
- McAtamney, L., & Coreltt, E. N. (1993). RULA: A survey method for the investigation of work-related upper limb disorders. Applied Ergonomics, 24(2), 91–99.
- Nimbarte, A. D., Zreiqat, M., & Chapman, M. (2012). Physical risk factors for neck pain among oculoplastic surgeons. IIE Annual Conference Proceedings 2012. HighBeam Research. Retrieved November 27, 2015, from https://www.highbeam.com/doc/1P3-2813506991.html
- Park, J. Y., Kim, K. H., Kuh, S. U., Chin, D. K., Kim, K. S., & Cho, Y. E. (2012). Spine surgeon’s kinematics during discectomy according to operating table height and the methods to visualize the surgical field. European Spine Journal, 21(12), 2704–2712.
- Park, J. Y., Kim, K. H., Kuh, S. U., Chin, D. K., Kim, K. S., & Cho, Y. E. (2014). Spine surgeon’s kinematics during discectomy, part II: Operating table height and visualization methods, including microscope. European Spine Journal, 23(5), 1067–1076.
- Plantard, P., Auvinet, E., Pierres, A. S., & Multon, F. (2015). Pose estimation with a kinect for ergonomic studies: Evaluation of the accuracy using a virtual mannequin. Sensors, 15(1), 1785–1803.
- Sivak-Callcott, J. A., Diaz, S. R., Ducatman, A. M., Rosen, C. L., Nimbarte, A. D., & Sedgeman, J. A. (2011). A survey study of occupational pain and injury in ophthalmic plastic surgeons. Ophthalmic Plastic & Reconstructive Surgery, 27(1), 28–32.
- Steinhilber, B., Hoffmann, S., Karlovic, K., Pfeffer, S., Maier, T., Hallasheh, O., … Sievert, K.-D. (2015). Development of an arm support system to improve ergonomics in laparoscopic surgery: Study design and provisional results. Surgical Endoscopy, 29(9), 2851–2858.
- Valachi, B. (2008). Practice dentistry pain-free: Evidence-based ergonomic strategies to prevent pain and extend your career. Portland, ME: Posturedontics Press.
- Wauben, L. S. G. L., van Veelen, M. A., Gossot, D., & Goossens, R. H. M. (2006). Application of ergonomic guidelines during minimally invasive surgery: A questionnaire survey of 284 surgeons. Surgical Endoscopy and Other Interventional Techniques, 20(8), 1268–1274.
- Yang, J., Marler, T., & Rahmatalla, S. (2010). Multi-objective optimization-based method for kinematic posture prediction: Development and validation. Robotica, 29(02), 245–253.