Abstract
The Centers for Disease Control and Prevention estimates 1.6–3.8 million concussions occur each year. Research cites that 21% of traumatic brain injury in children/youth results from participation in sports and recreational activities. However, current methods to assess concussion, which includes balance, neurocognitive, and self-report assessments, provide conflicting validity and reliability. Thus, many sports medicine professionals seek more objective measures to assess the severity and outcomes of a concussive event. The purpose of this study was to utilize a novel technology to determine if there were differences in blink reflex parameters among baseline, active, and concussed athletes. Twenty-four Division I football athletes participated in this study. Routine pre-season baseline data were collected on athletes as well as assessments of their blink reflex parameters using a blink reflexometer. Significant differences were found in blink parameters between baseline and active measurements in latency, differential latency, lid velocity, log of time to open, log of number of oscillations, and log of total blink time. In addition, significant differences were found between baseline and post-head impact in latency, differential latency, log of time to open, and log of number of oscillations. In comparing head impact and active play, blink latency decreased with head impact but increased in active play and differential latency and log of number of oscillations increased with head impact but decreased in active play. The results of this study suggest that use of a blink reflexometer provides quantifiable and objective outcomes to complement the concussion assessment protocol for athletes.
Public interest statement
Athletes participating in collision/contact sports have a significantly higher risk of experiencing a concussion during practice and competition. However, the ability to properly evaluate and diagnosis concussion has been a challenge as is recognized by medical professionals and researchers. Current methods to ascertain the extent of a concussion include self-report measures, balance performance, symptom evaluations, and neurocognitive assessments. However, the problem with many of these measures is the inability to objectively measure the extent of the concussion due to the athlete’s ability to provide spurious data during baseline or post-concussive testing. The outcomes of this study suggest that a blink reflexometer may provide an additional objective measure to support decisions in the diagnosis of concussion. Specifically, it appears to be a valid and reliable tool to discriminate between individuals who have likely suffered a concussion and those who have simply been involved in active play.
1. Introduction
The prevailing definition of a concussive injury is disruption of normal brain activity caused by rapid acceleration and deceleration of brain matter (Centers of Disease Control & Prevention, Citation2015, Citation2016; Jordan, Citation2013). Concussion can occur with or without the loss of consciousness (American Academy of Neurology, Citation1997) and its impact on an individual’s health is wide-spread, including physiological and metabolic changes within the brain affecting cognitive and emotional function (Dimberg & Burns, Citation2006; Jordan, Citation2013; Majerske et al., Citation2008; McCrea, Prichep, Powell, Chabot, & Barr, Citation2010). Athletes participating in collision/contact sports are at heightened risk for suffering a concussive event (Guskiewicz, Weaver, Padua, & Garrett, Citation2000; Powell & Barber-Foss, Citation1999), in fact, roughly 250,000 (individuals age 19 and younger) visit a US emergency department for a sport or recreation-related concussion annually (Centers of Disease Control & Prevention, Citation2016). In addition, the potential for repeated concussive events is of importance with one study finding that of the 6.3% of concussed collegiate football players, 14.7% went on to experience a second concussion (Guskiewicz et al., Citation2000). Repeated head impacts can result in second impact syndrome (Quintana, Citation2016) or chronic traumatic encephalopathy both with the potential for long-term disabilities or death (Vile & Atkinson, Citation2017). Thus, the ability to accurately diagnose concussions and identify athletes at risk for long-term complications is an important clinical goal.
Despite the number of athletes affected, the ability for sports medicine professionals to confidently diagnosis and monitor concussion recovery is a challenge recognized by organizations such as the National Athletic Trainers Association (NATA) (Guskiewicz et al., Citation2004; McCrory et al., Citation2013). Current methods of diagnosing concussion typically include self-report and a battery of tests, including neurocognitive function and balance performance, aimed at evaluating symptoms associated with concussions (Broglio, Macciocchi, & Ferrara, Citation2007; Guskiewicz et al., Citation2004). Of these, only one adult and one pediatric test evaluating neurocognitive function are FDA approved for concussion diagnosis (U.S. Food & Drug Administration, Citation2016). However, research on neurocognitive testing shows poor validity across age groups (Barlow, Schlabach, Peiffer, & Cook, Citation2011; Nakayama, Covassin, Schatz, Nogle, & Kovan, Citation2014) and low test-retest reliability, with 22-46% of healthy controls being misclassified as impaired (Resch et al., Citation2013). This issue is compounded by the admission of student and professional level athletes who have hidden or would hide the symptoms of a concussion to avoid missing participation time (Chrisman, Quitquit, & Rivara, Citation2013; Torres et al., Citation2013). As such, there remains a clinical need for an objective diagnostic test that cannot be manipulated.
This study examined the utility of non-invasive measurements of the blink reflex as a diagnostic test for concussion. The blink reflex is a primitive brainstem response to an external stimulus, such as air, visual cues or electrical signals, which is affected by multiple neurological disorders (Esteban, Citation1999; Helmchen & Rambold, Citation2007), including those that affect the dopaminergic circuit that controls the eyelid (Helmchen & Rambold, Citation2007). Previous studies using electromyography have shown that diffuse axonal injury (Formisano et al., Citation2009) and exercise (Coco et al., Citation2013) result in measurable changes in the blink reflex. Based on this knowledge, we employed an innovative technology incorporating high speed videography with air puffs (Haerich, Citation1998) to determine whether a head impact suspected of causing a concussion results in changes in the blink reflex that can be detected non-invasively. Further, we assessed whether changes in the blink reflex could discriminate between players receiving a head impact and those who had simply been involved in physical activity. We hypothesize that this technology can reliably discriminate between athletes who have and have not suffered from concussion.
2. Methods
Twenty-four Division I male football athletes between the ages of 18–22 were included in this study over the 2015 and 2016 athletic seasons. Prior to the beginning of the study, subjects read and signed an informed consent document, which described the procedures of this study. The consent form informed subjects of their ability to withdraw from the study at any time with no negative consequence. IRB approval for this study and all procedures was obtained through the Institutional Review Board for use of Human Subjects prior to the beginning of the study.
Pre-season baseline data, including an athletic history and physical examination, were collected on each subject. Baseline Biodex Balance System SD (Biodex Medical Systems, Inc., Shirley, NY) assessments and baseline symbols modalities tests were also completed on each athlete. Along with these routine pre-season assessments, we utilized the Blink Reflexometer (Tasi et al., Citation2017) (described below) to obtain baseline blink reflex data on each subject. Data was collected throughout the season if a concussion occurred or was suspected to occur. The specific measurements collected after a concussion included: blink reflex, the Acute Concussion Evaluation (ACE), and a symptoms and severity checklist to assess symptoms such as headache, nausea, difficulty remembering, heart rate, and blood pressure.
The blink reflexometer is a high-speed videography-based device used to trigger, record and analyze a blink reflex. The blink reflexometer consists of a mask, a stimulation system, a housing unit, a camera, an external controller and processor, and a user interface (Figure ). To capture the blink reflex response, a subject placed their face against the mask and aligned their eyes to internal mirrors within the housing unit. The test administrator, who was able to view real-time images of the subject’s eyes on the user interface, confirmed proper eye alignment prior to manually commencing the video-recorded test. The video segment was captured at 280 FPS (frames per second) which gave each frame a 3.5 ms window. To elicit the startle response, the stimulation system administered, via adjustable nozzles, three air puffs over a 20 s time frame to the outer corner of either the right or left eye, with the laterality and timing of the puffs randomly assigned. Microphones (CME-1538-100LB, CUI Inc., Tualatin, OR), connected to the processor, were positioned at the exit of the nozzles to capture the sound of the air exiting. The microphone recording provided a time stamp of stimulation delivery to the eye, which was used for blink reflex parameter calculations. After the 20-s test was administered, the subject had time to rest (approximately 20 s) while the software processed the video. Each subject received a total of two or three 20 s tests during a session (total of 6 to 12 puffs per session), with results of the sessions analyzed and means recorded.
Figure 1. Blink reflexometer housing unit and software interface. Tubing connected to the left end of the housing unit delivers a puff of compressed air to the subject’s eyes.
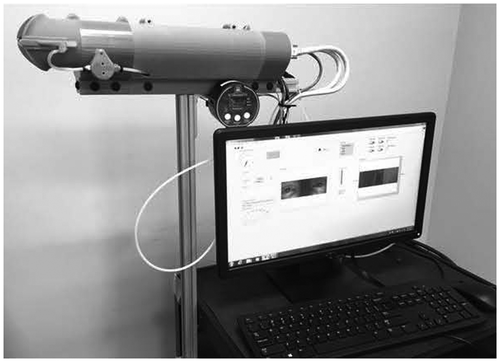
Processing of the video included detecting the edge of the both eyelids using custom LabVIEW software (National Instruments, Austin, TX). The program then tracked, using an edge detection function, the vertical positions of each eyelid through the entire image sequence. Frames were converted to time based on the collection frequency. For each eyelid, pixel location per time was used to chart a displacement profile (Figure (A)). To establish reference positions for blink parameter measurements, a rest position and threshold were defined as follows:
Tonic Lid Position: moving average of the pixel location of the top eyelid when not in a blink
Threshold: 20 pixels below tonic lid position
Figure 2. Illustrations of time-displacement profiles of upper lid movement during and after a stimulated blink. (A) Baseline blink reflex time-displacement profile. (B) Blink reflex time-displacement profile after active play. Latency is increased. Differential latency is decreased. Log of number of oscillations is less. (C) Blink reflex time-displacement profile after a head impact causing a concussive event. Latency is decreased. Differential latency is increased. Log of number of oscillations is increased.
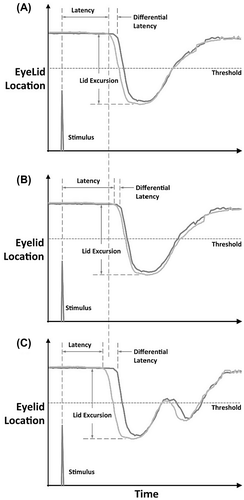
From the displacement profile for each eyelid, differences within and between subjects were assessed for the following parameters:
Ipsilateral: stimulated side
Contralateral: side opposite the stimulation
Latency: time differential between stimulation and ipsilateral eye movement
Differential Latency: time differential between the start of ipsilateral eye movement and the start of contralateral eye movement
Lid Excursion: distance traveled by the eyelid from the tonic lid position to closed position measured in pixels
Lid Velocity: average eyelid speed (pixels/sec) in first 7 frames following start of eyelid movement
Time to Close: time for lid to travel from tonic lid position to the closed position
Time to Open: time for lid to travel from closed position back to tonic lid position
Total Blink Time: time from start of eyelid movement until it returns to its tonic lid position
Time under Threshold: time that the eyelid spends below the threshold position
Number of Oscillations: cycles of up and down upper eyelid movement after a stimulated blink
Delta 30: time difference between the ipsilateral eye and contralateral eye after the lids had moved 30 pixels from the tonic lid position.
Subjects were divided into two groups, “Head Impact” and “Control”, during the study depending on if they were suspected of having suffered a concussive event during the study period. Pre-season blink reflex measurements were taken to establish “baseline” parameters for each subject. Control subjects were also tested after a practice to collect “active” blink reflex parameters. Head Impact subjects were tested as soon after the head impact as possible (1–48 h) to collect “Post-Head Impact” blink reflex parameters.
2.1. Statistical analysis
Athlete measurements were defined into one of 4 categories: (1) Baseline Control, (2) Active Control, (3) Baseline Head Impact, and (4) Post-Head Impact. A linear mixed model (LMM) was used to account for the correlation within subjects which resulted from repeated measures captured on the same subject using a random subject effect. The LMMs included a main effect for athlete type and a random subject effect to account for correlation between measures collected on the same subject. Different correlation structures (e.g. compound symmetry, unstructured) and the final correlation structure was selected based on Akaike’s Information Criterion. Comparisons between baseline and active measures within Control athletes, baseline and post-head impact measures within Head Impact athletes, and between the differences in the changes observed in Control and Head Impact athletes were assessed using a series of linear contrast statements from the models. All model assumptions were checked graphically and log transformations were considered if model assumptions were violated. Blink measures that met the statistical assumptions for an LMM model included latency, differential latency, delta 30, lid excursion, and lid velocity. The blink measures time to open, time to close, time under threshold, log of number of oscillations, time to first oscillation, and total blink time were all log transformed in the analysis to meet statistical assumptions.
All analyses were conducted in SAS 9.4 (SAS Institute, Inc., Cary, NC).
3. Results
Data were collected on 14 athletes with at least one head impact suspected of resulting in a concussion (2 players had 2 head impacts; 1 player had 3 impacts) and 10 control players who were age matched and had no history of concussive events.
3.1. Changes in blink parameters resulting from physical activity in control athletes
Significant differences in blink parameters between baseline and active measurements in Control athletes were observed for latency, differential latency, lid velocity, log of time to open, log of number of oscillations, and log of total blink time (Figure and Table ). Specifically, active play resulted in increased in blink latency (p < 0.001), decreased differential latency (p = 0.017), decreased lid velocity (p = 0.005), longer time to open (p = 0.037), fewer oscillations (p < 0.001), and shorter total blink duration (p = 0.042) (Figure (B)). There were no significant differences in delta 30, lid excursion, log of time to close, or log of time under threshold.
Figure 3. Blink reflex parameter changes from baseline due to active play or head impact.
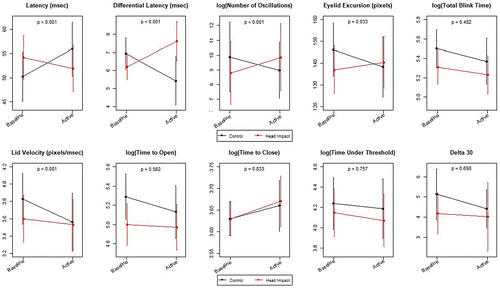
Table 1. Estimated mean differences (and standard errors) within and between groups
3.2. Changes in blink parameters resulting from a head impact
Significant differences between blink parameters measured at baseline and post-head impact in Head Impact athletes were observed for latency, differential latency, log of time to open, and log of number of oscillations (Figure and Table ). Specifically, head impacts resulted in decreased blink latency (p < 0.028), increased differential latency (p < 0.001), decreased log of the time to open (p = 0.044); and increased log of number of oscillations (p = 0.029) (Figure (C)). There were no significant differences in delta 30, lid excursion, lid velocity, log of time to close, log of time under threshold, or log of total blink time. In addition, comparisons between the two groups, control and head impacted players, for values with each outcome at rest were examined using contrasts statements. No statistically significant differences were noted in baseline values for any outcomes between the two groups.
3.3. Discrimination between active controls and post-head impact athletes
Significant differences between the changes observed in blink parameters in Active Controls and Head Impact athletes were observed for blink latency, differential latency, lid excursion, and log of number of oscillations. Head Impact athletes had decreased blink latency post-head impact compared to baseline, while Active Control athletes had increased blink latency after activity relative to baseline (p < 0.001). Head Impact athletes had increased differential latency after post-head impact relative to baseline, while Active Control athletes had decreased differential latencies after activity relative to baseline (p < 0.001). Head Impact athletes had larger lid excursions post-head impact relative to baseline, while Active Control athletes had smaller lid excursions after activity relative to baseline (p = 0.033). Head Impact athletes had increased log of number of oscillations post-head impact, while Active Control athletes exhibited a decrease in the log of number of oscillations relative to baseline (p < 0.001).
4. Discussion
Our study utilized a novel device to assess whether head impacts suspected of resulting in a concussion produced changes in the blink reflex that could be detected using non-invasive measurements. Then we assessed whether those changes could discriminate between a concussive event and mere physical activity, such that the approach has potential as a field-side diagnostic tool. The results show that athletes, who experienced a head impact, had a decrease in latency, increase in differential latency, larger lid excursions, and an increase in oscillations post injury as compared to the active controls. In lay terms, concussed athletes started blinking sooner, had a greater discrepancy between timing of right and left eye lid movement, had a more open tonic lid position, and demonstrated hyperexcitability in their blink response.
Concussive events result in symptomatic deficits in attention, executive function, learning and memory. As such, tools have been developed to assess cognitive changes indicative of brain injury. However, concussive trauma is not restricted to the regions of the brain responsible for cognitive function and does not address physiological changes that may occur with a concussive event. Such trauma has been cited to result in deep white matter hyperintensities (areas in the white matter that indicate due to axonal loss) that have been detected via MRI 24–72 h post concussive event (Hughes et al., Citation2004). Boele and colleagues (Citation2010) cited that lesions in the rabbit cerebellar cortex were related to abnormal blink responses, with shortened latency and amplitude of the conditioned eye blink response. For humans, Fitzek and colleagues also cited specific pathways which were affected by lesions, noting abnormalities in the R2 loop of the blink reflex with that loop passing through the descending trigeminal spinal track and onward to the spinal trigeminal nucleus (Fitzek et al., Citation1999). Thus, understanding the effect of such lesions and areas where these lesions occur will be important in understanding effect on blink reflex.
In addition, concussions which result in diffuse axonal injury produces alterations in neurotransmitter levels, including dopamine (Bales, Kline, Wagner, & Dixon, Citation2010). Changes in dopamine levels and the time course over which those changes occur may explain the results found in our study in both the post-head impact and active control groups. Previous studies have shown that concussions elicit time dependent alterations in dopamine in various regions of the brain (Wagner et al., Citation2005), with low levels found shortly after injury (McIntosh, Yu, & Gennarelli, Citation1994). It has also been shown that exercise alters neurotransmitter levels, including acute increases in dopamine and GABA (Chaouloff et al., Citation1987). Not surprisingly, altered dopamine levels are found in several other neurological disorders, including Parkinson’s disease, Huntington’s disease, and schizophrenia. All of these disorders exhibit changes in latency and excitability of their blink reflexes. While the acute decrease in dopamine levels reported after a concussion and the acute increase in dopamine levels reported after exercise support the opposite trend in blink reflex measurements that we observed between our post-head impact and active control groups, neurotransmitter levels are unlikely to be the sole causative reason for the changes we observed given the complex nature of a concussion. However, understanding the underlying mechanisms responsible for changes in the blink reflex is not necessary for the measurement to be an advantageous diagnostic tool.
Until now, field-side determination of whether an athlete has likely suffered a concussion has been determined based on the symptoms displayed. In this study we demonstrate the potential of the blink reflexometer to rapidly and objectively provide measurements of a primitive reflex that can assist the athletic trainer or medical personnel in determining the concussive status of an athlete. The ability to use reflex measurement to discriminate between individuals who have likely suffered a concussion and those who have simply been involved in active play will allow athletes to be removed from a game when appropriate. The fact that the reflex cannot be manipulated should add a level of confidence. The results of this study are not intended to endorse the use of this system, but are meant to support the blink reflex as a means to objectively assess impact immediately after and during recovery.
Funding
The authors received no direct funding for this research.
Additional information
Notes on contributors
D.P. Garner
D.P. Garner, PhD is a full professor in the Department of Health, Exercise and Sport Science at The Citadel in Charleston, SC. In addition, she serves as the Director of Undergraduate Research and the Director of Research and Grants for the institution. Previous to her position at The Citadel, she complete a post-doctoral fellowship in the Department of Neurology at the Medical University of South Carolina in Charleston, SC. Dr Garner continues her research into aspects of concussion diagnosis and seeks to elucidate blink parameter baselines in healthy and concussed individuals. This current research project meshes within exercise physiology and a field of neuroscience that has tremendous implications for those who work with athletes and the concussion protocol.
References
- American Academy of Neurology . (1997). Report of the quality standards subcommittee of the American Academy of Neurology. Neurology , 48 (3), 581–585.
- Bales, J. W. , Kline, A. E. , Wagner, A. K. , & Dixon, C. E. (2010). Targeting dopamine in acute traumatic brain injury. The Open Drug Discovery Journal , 2 , 119–128.
- Barlow, M. , Schlabach, D. , Peiffer, J. , & Cook, C. (2011). Differences in change score and the predictive validity of three commonly used measures following concussion in the middle school and high school aged population. International Journal of Sports Physical Therapy , 6 (3), 150–157.
- Boele, H. J. , Koekkoek, S. K. E. , & De Zeeuw, C. I. (2010). Cerebellar and extracerebellar invovlement in mouse eyeblink conditioning: The ACDC model. Frontiers in Cellular Neuroscience , 3 (19).
- Broglio, S. P. , Macciocchi, S. N. , & Ferrara, M. S. (2007). Sensitivity of the concussion assessment battery. Neurosurgery , 60 (6), 1050–1058.10.1227/01.NEU.0000255479.90999.C0
- Centers of Disease Control and Prevention . (2015). What is concussion? Author. Retrieved from https://www.cdc.gov/headsup/basics/concussion_whatis.html
- Centers of Disease Control and Prevention . (2016). Get the stats on traumatic brain injury in the United States . Retrieved from https://www.cdc.gov/traumaticbraininjury/get_the_facts.html
- Chaouloff, F. , Laude, D. , Merino, D. , Serrurrier, B. , Guezennec, Y. , & Elghozi, J. L. (1987). Amphetamine and α-methyl-p-tyrosine affect the exercise-induced imbalance between the availability of tryptophan and synthesis of serotonin in the brain of the rat. Neuropharmacology , 26 (8), 1099–1106.10.1016/0028-3908(87)90254-1
- Chrisman, S. P. , Quitquit, C. , & Rivara, F. P. (2013). Qualitative study of barriers to concussion symptom reporting in high school athletics. Journal of Adolescent Health , 52 (3), 330–335.
- Coco, M. , Alagona, G. , Perciavalle, V. , Rapisarda, G. , Costanzo, E. , & Perciavalle, V. (2013). Brainstem excitability is not influenced by blood lactate levels. Somatosensory & Motor Research , 30 (2), 90–95.10.3109/08990220.2013.769949
- Dimberg, E. L. , & Burns, T. M. (2006). Management of common neurologic conditions in sports. Clinics in Sports Medicine , 24 , 637–662.
- Esteban, A. (1999). A neurophysiological approach to brainstem reflexes. Blink reflex. Neurophysiologie Clinique/Clinical Neurophysiology , 29 , 7–38.10.1016/S0987-7053(99)80039-2
- Fitzek, S. , Fitzek, C. , Marx, J. , Speckter, H. , Urban, P. P. , Thomke, F. , … Hopf, H. C. (1999). Blink reflex R2 changes and localisation of lesions in the lower brainstem (Wallenberg’s syndrome): An electrophysiological and MRI study. Journal of Neurology, Neurosurgery & Psychiatry , 67 , 630–636.10.1136/jnnp.67.5.630
- Formisano, R. , Cicinelli, P. , Buzzi, M. G. , Brunelli, S. , Zafonte, R. , Vinicola, V. , … Sabatini, U. (2009). Blink reflex changes in parkinson following severe traumatic brain injury correlates with diffuse axonal injury. Medical Science Monitor , 5 (3), CR101–106.
- Guskiewicz, K. M. , Bruce, S. L. , Cantu, R. C. , Ferrara, M. S. , Kelly, J. P. , McCrea, M. , … McLeod, T. C. (2004). National athletic trainers’ association position statement: Management of sports-related concussion. Journal of Athletic Training , 39 (3), 280–297.
- Guskiewicz, K. M. , Weaver, N. L. , Padua, D. A. , & Garrett, W. E. (2000). Epidemiology of conussion in collegiate and high school football players. The American Journal of Sports Medicine , 28 (5), 643–650.10.1177/03635465000280050401
- Haerich, P. (1998). Using airpuffs to elicit the human blink reflex. Behavior Research Methods, Instruments, & Computers , 30 (4), 661–666.10.3758/BF03209484
- Helmchen, C. , & Rambold, H. (2007). The eyelid and its contribution to eye movements. In A. Straube & U. Buttner (Eds.), Neuro-ophthalmology neuronal control of eye movements (pp. 110–131). Basel: Karger.
- Hughes, D. G. , Jackson, A. , Mason, D. L. , Berry, E. , Hollis, S. , & Yates, D. W. (2004). Abnormalities on magnetic resonance imaging seen acutely following mild traumatic brain injury: Correlation with neuropsychological tests and delayed recovery. Neuroradiology , 46 , 550–558.
- Jordan, B. D. (2013). The clinical spectrum of sport-related traumatic brain injury. Nature Reviews Neurology , 9 , 222–230.10.1038/nrneurol.2013.33
- Majerske, C. W. , Mihalik, J. P. , Ren, D. , Collins, M. W. , Reddy, C. C. , Lovell, M. R. , & Wagner, A. K. (2008). Concussion in sports: Post-concussive activity levels, symptoms, and neurocognitive performance. Journal of Athletic Training , 43 (3), 265–274.10.4085/1062-6050-43.3.265
- McCrea, M. , Prichep, L. , Powell, M. R. , Chabot, R. , & Barr, W. B. (2010). Acute effects and recovery after sport-related concussion: A neurocognitive and quantitative brain electrical activity study. Journal of Head Trauma Rehabilitation , 25 (4), 283–292.10.1097/HTR.0b013e3181e67923
- McCrory, P. , Meeuwisse, W. , Aubry, M. , Cantu, Bob , Dvorak, J. , Echemendia, R. J. , … Sills, A. (2013). Consensus statement on concussion in sport – The 4th international conference on concussion in sport held in Zurich, November 2012. Clinical Journal of Sport Medicine , 23 , 89–117.10.1097/JSM.0b013e31828b67cf
- McIntosh, T. K. , Yu, T. , & Gennarelli, T. A. (1994). Alterations in regional brain catecholamine concentration after experimental brain injury in the rat. Journal of Neurochemistry , 63 , 1426–1433.
- Nakayama, Y. , Covassin, T. , Schatz, P. , Nogle, S. , & Kovan, J. (2014). Examination of the test-retest reliability of a computerized neurocognitive test battery. The American Journal of Sports Medicine , 42 (8), 2000–2005.10.1177/0363546514535901
- Powell, J. W. , & Barber-Foss, K. D. (1999). Traumatic brain injury in high school athletes. JAMA , 282 (10), 958–963.10.1001/jama.282.10.958
- Quintana, L. M. (2016). Second impact syndrome in sports. World Neurosurgery , 91 , 647–649.10.1016/j.wneu.2016.04.035
- Resch, J. , Driscoll, A. , McCaffrey, N. , Brown, C. , Ferrara, M. S. , Macciocchi, S. , … Walpert, K. (2013). ImPact test-retest reliability: Reliability unreliable? Journal of Athletic Training , 48 (4), 506–511.10.4085/1062-6050-48.3.09
- Tasi, N. T. , Goodwin, J. S. , Semler, M. E. , Kothera, R. T. , Van Horn, M. , Wolf, B. J. , & Garner, D. P. (2017). Development of non-invasive blink reflexometer. IEEE Journal of Translational Engineering in Health and Medicine , 5 , 3800204.
- Torres, D. M. , Galetta, K. M. , Phillips, H. W. , Dziemianowicz, E. M. , Wilson, J. A. , Dorman, E. S. , … Balcer, L. J. (2013). Sports-related concussion: Anonymous survey of a collegiate cohort. Neurology: Clinical Practice , 3 (4), 279–287.10.1212/CPJ.0b013e3182a1ba22
- U.S. Food & Drug Administration (2016). FDA allows marketing of first-of-kind computerized cognitive tests to help assess cognitive skills after a head injury, 8–22. Retrieved from https://www.fda.gov/NewsEvents/Newsroom/PressAnnouncements/ucm517526.htm
- Vile, A. R. , & Atkinson, L. (2017). Chronic traumatic encephalopathy: The cellular sequela to repetitive brain injury. Journal of Clinical Neuroscience . doi:10.1016/j-jocn.2017.03.035
- Wagner, A. K. , Sokoloski, J. E. , Ren, D. , Chen, X. , Khan, A. S. , Zafonte, R. D. , … Dixon, C. E. (2005). Controlled cortical impact injury affects dopaminergic transmission in the rat striatum. Journal of Neurochemistry , 95 (2), 457–465.10.1111/jnc.2005.95.issue-2