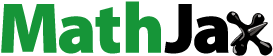
Abstract
Roller compacted concrete (RCC) pavement usage is limited to low-speed roads due to its low skid resistance, caused by the smooth surface texture that makes it unsuitable for use in high-speed traffic pavements. If used in high-speed roadways will lead to increased skid related accidents. In this study, crumb rubber was used as partially replaced with fine aggregate at levels 10, 20, and 30% by volumeto produce roller compacted rubbercrete (RCR) so as to improve the skid resistance of RCC pavement, and nano silica was added at 0, 1, 2 and 3% by weight of cementitious materials to mitigate loss of strength with incorporation of crumb rubber. The British Pendulum Number (BPN) was used to measure the skid resistance. Incorporating up to 20% crumb rubber and 2% nano silica increases both skid and impact resistance. The findings showed that RCR with 20% CR and 2% NS can be used for motorways, trunk, and class 1 roads. Finally, response surface methodology was used to develop the relationship between BPN in wet/dry conditions and crumb rubber/nano-silica. The analysis of variance for response surface methodology analysis shows that the quadratic models developed has a very good degree of correlation, and can be used to predict the skid resistance of RCR. The results of multi-objective optimization showed that an optimum mixture can be achieved with a 17.20% volumereplacement of fine aggregate and 1.87% nano silica addition by weight of cementitious materials to have the highest skid resistance RCR for pavement applications.
Public Interest Statement
The major problem of related to RCC pavement usage is its low skid resistance which limits its use to only low-speed roads. If RCC pavement is used for high-speed road, there will be high tendency of increased skid resistance accident. For RCC pavement to be used for high speed traffic roadways, a thin wearing surface course is normally laid above its surface. However, this increases the overall construction cost. Another option is to the use of high quality crush aggregates which will increase surface roughness and consequently skid resistance. Therefore this study looks into the possible ways of improving the skid resistance of RCC pavement in a more economical way. Hence, crumb rubber was used as partial replacement to fine aggregate due to higher friction coefficient between rubber (tire) and rubber (crumb rubber) compared to rubber (tire) and concrete (RCC pavement). Nano silica was added by weight of cement to also improve skid resistance of RCC pavement.
1. Introduction
According to ACI 207.5R-11 definition, roller compacted concrete (RCC) is a special type of concrete having zero-slump consistency in its fresh state and conveyed, cast and compacted using rock fill and earthwork equipment similar to those of asphaltic pavement construction (ACI 207.5R-11, Citation2011). It is highly economical compared to asphaltic and conventional concrete pavement. The reduction in the cost of pavement when RCC is used can be up to 30% and 20% compared to the asphaltic pavement and conventional concrete pavement respectively (Modarres & Hosseini, Citation2014). Due to the way it is consolidated and compacted, RCC is used for pavement and dam applications (Adamu, Mohammed, & Shafiq, Citation2017a; Adamu, Mohammed, Shafiq, & Liew, Citation2018). When used for pavement, its surface is exposed to heavy duty and low-speed vehicular operations (ERMCO, Citation2013). The sliding, skidding and rubbing of the vehicular wheels on the surface of RCC pavement causes wearing, tearing and abrasion on the pavement. Skid resistance which is mainly related to concrete or asphaltic pavement is a measure of the resistance to rotating and sliding of vehicles tires in contact with the concrete surface, and it is dependent on the surface texture of the concrete/asphalt (ERMCO, Citation2013). RCC pavement usage is limited to low-speed roads due to its low skid resistance and is caused by the smooth surface texture that makes it unsuitable for use in high-speed traffic pavements (Shoenberger, Citation1994). If used in high-speed roadways will lead to increase in skid related accidents (Asi, Citation2007). For RCC pavement to be used for high-speed traffic roadways, a thin wearing surface course is normally laid above its surface (ERMCO, Citation2013). However, this increases the overall construction cost. Another option is to the use of high-quality crush aggregates which will increase surface roughness and consequently skid resistance. The skid resistance of pavement is most commonly determined using the British Pendulum Number (BPN). For example, Gonzalez, Medley, and Muzzin (Citation2015) evaluated the skid resistance of nano silica concrete using the BPN test. Similarly, Ninomiya, Kuroiwa, and Takao (Citation2013) used the BPN test to determine the skid resistance of concrete paving blocks, Asi (Citation2007), also evaluated the skid resistance of asphaltic concrete mixes using BPN test method and concluded the method was effective. Yoshitake, Ueno, Ushio, Arano, and Fukumoto (Citation2016), evaluated the skid resistance of recycled concrete pavement using BPN test, and they reported the skid resistance of recycled concrete pavement is similar to the normal aggregate pavement. Frolova and Salaiová (Citation2017), evaluated the roughness of road section made with crumb rubber, and they reported the addition of crumb rubber increases the skid resistance of the road section.
It is clear that incorporating crumb rubber as a partial replacement to fine aggregate, and the addition of nano silica into concrete will enhance its skid t resistance. Therefore in this study, crumb rubber will be used as a partial replacement to fine aggregate, and nano silica will be added by weight of cementitious materials to improve the skid resistance of RCC pavement.
2. Materials and methods
2.1. Materials
In this study, Type I ordinary Portland cement which conforms to ASTM C150 M-1, having properties as shown in Table was used. Natural sand having nominal maximum size aggregate of 4.75 mm, specific gravity 2.65, fineness modulus 2.86, water absorption 1.24%, and particle size gradation was used as fine aggregate. Two sizes of coarse aggregate which are 19 mm maximum size aggregate having a specific gravity 2.66 and water absorption 0.48%, and chips of 6.35 mm maximum size with a specific gravity 2.55 and water absorption 1.05% was used. Three sizes of crumb rubber were combined so as to achieve gradation similar to fine aggregate. After several series of trial combinations, using sieve analysis according to ASTM D5644, final proportion of 40% of 0.595 mm (mesh 30) size, 40% of 1–3 mm size, and 20% of 3–5 mm size were used. Their combined particle size curve is shown in Figure . One of the basic requirement for any RCC pavement is that 2–8% of the aggregate should be materials finer than 75 μm so as to produce a cohesive paste with lower void contents (ACI 211.3R, Citation2002; Adamu, Mohammed, & Shafiq, Citation2017b). Therefore, in this study, class F fly ash which conforms to ASTM C612 and having properties as shown in Table was used as mineral filler. Strong hydrophobic nano silica size 10–25 nm has been used as an additive to the cement.
Table 1. Chemical compositions and physical properties of cement and fly ash
2.2. Mix procedure
The mix proportioning has been carried out using the soil compaction geotechnical approach according to ACI 211.3R. It involves a series of stages.
(1) | Determination of optimum proportions of fine aggregate, coarse aggregate, and mineral filler so that the combined aggregate grading curve falls within the limit recommended by and US army corps of Engineers (CRD-C 162, Citation1992). The combined aggregate gradation curve showed in Figure was obtained using a combination of 55% fine aggregate, 20% of 19 mm coarse aggregate, 20% of 6.3 mm chips coarse aggregate, and 5% of fly ash as a mineral filler. | ||||
(2) | The optimum moisture content (OMC) and maximum dry density determination in accordance with ASTM D 1557-12e (ASTM D1557, Citation2012). The OMC and MDD of four RCC mixes have been produced using different cement contents; 12, 13, 14, and 15% by weight of dry aggregates. For each cement content, five mixes were produced using different water content ranging from 4.5 to 6.5% by weight of dry aggregate, to obtain the moisture content -density relationship. The optimum moisture content for 12, 13, 14 and 15% cement contents have been found to be 5.46, 5.56, 5.92 and 6.09% respectively. | ||||
(3) | Four RCC mixes have been produced utilizing 12, 13, 14 and 15% cement content using their corresponding OMC obtained from step ii as the amount of water for the mix. The flexural strength of each mix at 28 days have been determined, and the relationship between cement content and flexural strength has been plotted as shown in Figure . | ||||
(4) | Based on target flexural strength of 4.8 MPa, 13% cement content was selected which will be used to derive the proportion of all the mixes in this study. | ||||
(5) | Based on the required flexural strength and calculations of constituent materials, 0.42 water to cement ratio was used. Superplasticizer was added at 1% by weight of cementitious materials, and the water content reduced by 12%, thus lowering the water to cement ratio to 0.37. |
To study the effect of crumb rubber and nano silica on the skid resistance of RCC pavement, thirteen mixtures have been prepared with different variations of crumb rubber at 10, 20 and 30% replacement by volumeof fine aggregate, while nano silica was added at 0, 1, 2 and 3% by weight of cementitious materials, to produce roller compacted rubbercrete (RCR).
Each mix was assigned a designation code based on crumb rubber and nano silica used, for example, M10C2 N refers to RCR mixture with 10% of crumb rubber and 2% of nano-silica. RCR mix proportions are shown in Table .
Table 2. Summary of constituent materials for RCR
2.3. Samples preparations and test methods
2.3.1. Compaction method
RCR mix for pavement applications is very steep, adequate compaction using vibration table cannot be achieved, which can lead to voids and honeycombs in the hardened mix thereby reducing its mechanical energy. Therefore in order to achieve proper compaction and consolidation, vibration hammer of 50 Hz capacity. Each sample was compacted in three equal layers. Full compaction is achieved when a ring of mortar forms across the periphery of the base plate.
2.3.2. Skid resistance test
The skid resistance of RCR was measured after 28 days using the British Pendulum Tester (BPT) in accordance with ASTM E303 (2013). Specimens of 90 mm length by 50 mm width and 15 mm thickness were produced using the BPT mold as shown in Figure (a). Due to the smaller sizes of the BPT molds, as shown in Figure (b), hand mixing was done, and the maximum coarse aggregate size of 6.35 mm was used. After casting, the specimens were left in the mold for 24 h prior to demolding. The specimens were then cured in clean water for 28 days before testing. For each mixture, three specimens were prepared and tested; the average values were then recorded. The skid resistance test was determined in both wet and dry condition to simulate the real behavior of the pavement. The specimen was placed in the test specimen holder on the lower surface of the BPT and held in position by tightening the bolts. To determine the BPN in a dry condition, the pendulum arm was raised up until it is horizontal and locked; it was then swung across the specimen so that the edge of the rubber slider slides over the top of the specimen, then the pendulum immediately stopped. The BPN is then recorded from the calibration scale attached to a pointer. For each mix and sample, five readings were taken and the average value recorded. The same procedure was repeated for all the specimens in a wet condition by applying sufficient water so as the surface becomes totally wet. For each swinging, the surface was rewetted again until the last reading was recorded
3. Results and discussions
3.1. Skid resistance of RCR
The skid resistance for roller compacted rubbercrete (RCR) mixtures was measured using the British Pendulum Number (BPN). The higher the BPN of a mixture, the rougher the surface and the higher its skid resistance (frictional coefficient). As shown in Figure , the BPN of RCR increases with up to 20% crumb rubber as a replacement to fine aggregate. This is mainly due to the projection of crumb rubber particles above the surface of RCR which makes it rougher as shown in Figure , thereby increasing the frictional force/skid resistance (Thomas et al., Citation2016). The projected crumb rubber on the RCR surface increases the contact area between the vehicle tire and rubber-aggregate particles. With the friction coefficients between rubber and rubber been higher (1.16) compared to rubber-concrete (0.85), this resulted to increase in skid resistance (Barnes, Citation2015). However, the skid resistance decrease when 30% crumb rubber was used, and this is due poor bonding between crumb rubber and hardened cement paste, which leads to loss of materials (abrading) when skid/frictional force is applied and consequently reduction in skid resistance (Ling, Nor, & Lim, Citation2010). Furthermore, the BPN of RCR in dry condition was higher than in wet condition, and this due to the fact that wet pavements in wet conditions produce more slippery surface texture, thus decreasing skid resistance.
The addition of nano silica increases the skid resistance of RCR for all crumb rubber contents as shown in Figure . For example, the addition of 1, 2 and 3% nano silica to RCR with 20% crumb rubber increases the skid resistance by 4.1, 12.12, and 0.58% respectively compared to 0% nano-silica. This is due to the bonding enhancement between crumb rubber and hardened cement paste caused by nano silica, which reduces material lose in RCR and consequently increases skid resistance. It is also due to the ability of nano silica to act as an abrasive/skidding powder, thereby increasing the resistance of RCR to skidding forces (Nazari & Riahi, Citation2011).
Comparing the skid resistance values (BPN) with the recommended minimum skid resistance values (wet condition) by ASTM E303 (Citation1997) as shown in Table , none of the RCR mixes can be used for category A such as roundabouts as their skid resistance values (BPN) in wet condition were less than 65. In addition, only RCR mixture with 20% CR and 2% NS (M20C2 N) can be used for category A such as motorways, trunk and class 1 roads as it has a BPN greater than 55. However, all the RCR mixes can be used for category C i.e. other sites such as rural roads or freeways except M30C0 N and M30C3 N.
Table 3. Recommended minimum skid resistance (based on BPN) (ASTM E303, Citation1997)
The relationship between skid resistance of RCR in wet and dry conditions is shown in Eqn 1, and Figure . A good correlation exists between them with a high degree of determination (R
2 = 0.914).(1)
(1)
where S d and S w are the skid resistances (BPN) of RCR at dry and wet conditions respectively.
3.2. Response surface methodology
3.2.1. Model development
Response surface methodology (RSM) is the most suitable statistical and mathematical technique that can be used. In addition, RSM can be used for model multi-objective optimization by setting defined desirable goals based on either the responses or the variables (Mohammed & Adamu, Citation2018a, Citation2018b). The response surface can be expressed mathematically by a single formulation shown in Equation (2) (Montgomery, Citation2008).(2)
(2)
where ε is the observed error for the response R, v 1 and v 2 are the variables. The predictable response can be rewritten as G(r) = f (v 1, v 2) = β. Then β = f (v 1, v 2) is called the response surface.
In this study, the Design expert software version 10 was utilized for the RSM analysis, where the face-centered central composite design (FCCCD) method was used to develop the model for predicting the British pendulum number (BPN) i.e. skid resistance of RCR in both dry and wet conditions. The independent variables used are; crumb rubber (CR) with variation levels 10, 20, and 30%; and nano silica (NS) with levels 1, 2, and 3%. Based on the different combinations of the variables, 13 runs were developed by the RSM software as shown in Table .
Table 4. Variable combinations and responses
3.2.2. Analysis of variance for the developed models
Table shows the summary of the analysis of variance (ANOVA) result for the developed response models i.e. the British pendulum number (BPN) in dry and wet conditions. For both dry and wet conditions, the quadratic model was more suitable and was therefore selected. Both models were found to be significant having 95% confidence interval (p ˂ 0.05), with F values of 53.16 and 81.49 for BPN (wet) and BPN (dry) respectively. Similarly, the significance of all the model terms was verified using the 95% confidence interval. For BPN (wet) model, all the model terms were found to be significant as their P-values were less than 0.05, while for BPN (dry) model all model terms were significant except the interaction between crumb rubber and nano silica (CR*NS). The positive and negative signs before a model term designate synergistic and antagonistic effect of the individual variable on the Cantabro loss of RCR respectively. The significance of the model can be further explained using the lack of fit (Mohammed, Adamu, & Liew, Citation2018). From Table , the lack of fit for both models was not significant relative to pure error, therefore the models were said to have a good fit, as non-significant lack of fit implies a good fit (Montgomery, Citation2008).
Table 5. ANOVA for developed response models
The developed model equations with all the model terms are given in Equations (3) and (4) for BPN (wet) and BPN (dry) respectively. The positive and negative signs before a model term designate synergistic and antagonistic effect of the individual variable on the skid resistance (BPN) of RCR(3)
(3)
(4)
(4)
where BPN = British Pendulum Number; CR = Crumb rubber; NS = Nano silica.
The adequacy and degree of correlation (determination) of each response model can also be explained by the high regression coefficient (R 2) which also explains the fitness and quality of the models as shown in Table . The BPN (wet) and BPN (dry) have R 2 values close to unity, this shows that the models have a higher degree of correlation between the predicted models and experimental data, and there is a close fitness between the data and their regression lines. To further explain the fitness and validity of the models, for all models, the adjusted R 2 and the predicted R 2 were in reasonable agreement to each other as their differences are less than 0.2. The variability of the models with reference to the experimental data was also checked using their standard deviations and coefficient of variations. The low values of the models show that the experimental data fitted the developed models with higher correlation degree. Furthermore, for all the developed models their adequate precision values are desirable with the adequate signal, as they are greater than 4, therefore models can be used in navigating the design space
Table 6. Models validation
The adequacy and degree of correlation of the models are checked graphically by plotting normal probability against residuals and the predicted versus actual data. From Figure (a) and (b), it can be seen that all the models are normally distributed, as all the data points were aligned along the straight normal distribution line. In addition, from Figure (a) and (b), the predicted responses and the actual (experimental) data for all the models are in agreement to each other with a high degree of correlation, as the data points aligned along the straight trend line. Therefore, the developed models in Equations (3) and (4) can be used to predict the skid resistance of RCR in wet and dry conditions respectively.
The 3-dimensional (3D) response surface plots for BPN (wet) and BPN (dry) are shown in Figure (a) and (b) respectively. It can be seen the incorporation of crumb rubber up to 20% as a partial replacement to fine aggregates and the addition of up to 2% nano silica by weight of cementitious materials increases the skid resistance of RCR both in wet and dry condition. The highest BPN values for both wet and dry condition were shown as the reddish region in the 3D curves and is obtained using a combination of 29% crumb rubber and 2% nano-silica.
The 2-dimensional contour plots the developed models are shown in Figure . It can be seen that all the contour lines are oval in shape meaning there is a perfect interaction between CR and NS for all the responses, BPN (wet) response having the best interaction (Mohammed & Adamu, Citation2018a). The reddish regions on the contour plots show the best combinations to give the optimum values, while the bluish region on the plots. In this case combination of 20% crumb rubber with 2% NS gave the optimum combinations.
3.2.3. Multi-objective optimization
A multi-objective optimization has been carried to using RSM to determine the best optimum combinations of crumb rubber and nano silica that could yield maximum skid resistance (BPN) of RCR pavement both in wet and dry conditions. The optimization goals and the results for the multi-objective optimization are presented in Table . Based on the optimization goals, the best optimal mixture proportions selected by the RSM software is obtained by combining 17.2% of crumb rubber as a fine aggregate replacement with 1.187% of nano silica as addition to cement to give the maximize responses with the combined desirability of 95.3%. This can also be shown graphically in Figure .
Table 7. Multi-objective optimization
4. Conclusions
The following conclusions can be drawn from this study
• | The skid resistance of RCR which was measured using the British Pendulum Number (BPN) increases with increment in percentage replacement of fine aggregate with crumb rubber up to 20%. | ||||
• | The addition of nano silica up to 2% by weight of cementitious materials increases the skid resistance of RCR. The skid resistance of RCR is more dependent on its surface texture compared to its strengths. | ||||
• | Based on the recommended skid resistance values, RCR with 20% CR and 2% NS can be used for motorways, trunk, and class 1 roads, and all the other RCR mixes can be used for any other sites such as freeways or rural roads except RCR with 30% CR and 0% NS, and RCR with 30% CR and 3% NS. | ||||
• | A quadratic model was developed using RSM to predict the skid resistance of RCR in both wet and dry conditions using crumb rubber and nano silica as the variables. The result of the RSM analysis shows there is a good correlation between the variables and the response | ||||
• | The results of the optimization process using RSM shows that the combination of 17.20% crumb rubber as a fine aggregate replacement by volumeand addition of 1.87% nano silica by weight of cement will be yielding the best skid resistance both in wet and dry conditions. |
Funding
This work was supported by Ministry for Higher Education Malaysia [under grant number PRGS/1/13/TK03/UTP/02/02].
Acknowledgment
The authors would like to thank Universiti Teknologi PETRONAS (UTP) Malaysia for fully supporting this work, and the Technologist of Highway Engineering Laboratory UTP.
Additional information
Notes on contributors
Musa Adamu
Musa Adamu is currently undergoing his Ph.D in Civil Engineering at Universiti Teknologi Petronas (UTP) Malaysia. His specialization is materials and structural engineering, and his current research is utilization of crumb rubber and Nano silica in roller compacted concrete pavement.
Bashar S. Mohammed
Bashar S. Mohammed currently an Associate Professor in the Department of Civil and Environmental Engineering, UTP Malaysia. He has been involved in teaching and research for the many years. His current research interest is application of crumb rubber and nano silica in concrete, Engineered cementitious concrete (ECC).
Mohd Shahir Liew
Mohd Shahir Liew is currently a full Professor in the Department of Civil and Environmental Engineering, UTP Malaysia. He has been involved in teaching, research for many years. His area of research interest is structural dynamics, and offshore engineering structures.
References
- ACI 207.5R-11 . (2011). Report on roller-compacted mass concrete . Michigan: American Concrete Institute.
- ACI 211.3R . (2002). Guide for selecting proportions for no-slump concrete Michigan . Michigan: American Concrete Institute.
- Adamu, M. , Mohammed, B. S. , & Shafiq, N. (2017a). Flexural performance of nano silica modified roller compacted rubbercrete. International Journal of Advanced and Applied Sciences , 4 , 6–18.10.21833/ijaas.2017.09.002
- Adamu, M. , Mohammed, B. S. , & Shafiq, N. (2017b). Mechanical performance of roller compacted rubbercrete with different mineral fillers. Jurnal Teknologi , 79 , 75–88.
- Adamu, M. , Mohammed, B. S. , Shafiq, N. , & Liew, M. S. (2018). Effect of crumb rubber and nano silica on the fatigue performance of roller compacted concrete pavement. Cogent Engineering , 5 , 1436027.
- Asi, I. M. (2007). Evaluating skid resistance of different asphalt concrete mixes. Building and Environment , 42 , 325–329.10.1016/j.buildenv.2005.08.020
- ASTM D1557 . (2012). Standard test methods for laboratory compaction characteristics of soil using modified effort . West Conshohocken, PA: ASTM International.
- ASTM E303 . (1997). Standard test method for measuring surface frictional properties using the british pendulum tester . West Conshohocken, PA: ASTM International.
- Barnes, R. (2015). Friction between materials . United Kingdom: Concrete Society.
- CRD-C 162 . (1992). Standard practice for selecting proportions for roller compacted concrete (RCC) pavement mixtures using soil compaction concepts . Washington, DC: Department of the Army, Corps of Engineers.
- ERMCO . (2013). Guide to roller compacted concrete for pavement . Bruxelles: Author.
- Frolova, O. , & Salaiová, B. (2017). Analysis of road cover roughness on “control” road section with crumb tire rubber. Procedia Engineering , 190 , 589–596.10.1016/j.proeng.2017.05.384
- Gonzalez, S. L. T. M. , Medley, J. B. , & Muzzin, N. (2015). Assessing friction response of nanoconcrete for rigid pavements using British Pendulum Tester and Tribometer. In 94th Annual Meeting . Transportation Research Board.
- Ling, T. , Nor, H. , & Lim, S. (2010). Using recycled waste tyres in concrete paving blocks. In Proceeding of the Institution of Civil Engineers (pp. 37–45).
- Modarres, A. , & Hosseini, Z. (2014). Mechanical properties of roller compacted concrete containing rice husk ash with original and recycled asphalt pavement material. Materials & Design , 64 , 227–236.10.1016/j.matdes.2014.07.072
- Mohammed, B. S. , & Adamu, M. (2018a, January 1). Mechanical performance of roller compacted concrete pavement containing crumb rubber and nano silica. Construction and Building Materials , 159 , 234–251.10.1016/j.conbuildmat.2017.10.098
- Mohammed, B. S. , & Adamu, M. (2018b). Non-destructive evaluation of nano silica-modified roller-compacted rubbercrete using combined SonReb and response surface methodology. Road Materials and Pavement Design , 1–21. 10.1080/14680629.2017.1417891
- Mohammed, B. S. , Adamu, M. , & Liew, M. S. (2018). Evaluating the static and dynamic modulus of elasticity of roller compacted rubbercrete using response surface methodology. International Journal , 14 , 186–192.
- Montgomery, D. C. (2008). Design and analysis of experiments . Arizona State University, John Wiley & Sons.
- Nazari, A. , & Riahi, S. (2011). Abrasion resistance of concrete containing SiO2 and Al2O3 nanoparticles in different curing media. Energy and Buildings , 43 , 2939–2946.10.1016/j.enbuild.2011.07.022
- Ninomiya, Y. , Kuroiwa, Y. , & Takao, N. (2013). A Study on concrete paving with limestone aggregate and limestone powder. Cement Science and Concrete Technology , 67 (1).
- Shoenberger, J. E. (1994). User’s guide: Roller-compacted concrete pavement ( DTIC Document).
- Thomas, B. S. , Kumar, S. , Mehra, P. , Gupta, R. C. , Joseph, M. , & Csetenyi, L. J. (2016). Abrasion resistance of sustainable green concrete containing waste tire rubber particles. Construction and Building Materials , 124 , 906–909.10.1016/j.conbuildmat.2016.07.110
- Yoshitake, I. , Ueno, S. , Ushio, Y. , Arano, H. , & Fukumoto, S. (2016). Abrasion and skid resistance of recyclable fly ash concrete pavement made with limestone aggregate. Construction and Building Materials , 112 , 440–446.10.1016/j.conbuildmat.2016.02.185